the Creative Commons Attribution 4.0 License.
the Creative Commons Attribution 4.0 License.
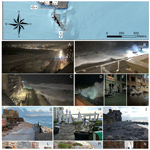
Characteristics and coastal effects of a destructive marine storm in the Gulf of Naples (southern Italy)
Gaia Mattei
Diana Di Luccio
Guido Benassai
Giorgio Anfuso
Giorgio Budillon
Pietro Aucelli
Destructive marine storms bring large waves and unusually high surges of water to coastal areas, resulting in significant damages and economic loss. This study analyses the characteristics of a destructive marine storm on the strongly inhabited coastal area of Gulf of Naples, along the Italian coasts of the Tyrrhenian Sea. This is highly vulnerable to marine storms due to the accelerated relative sea level rise trend and the increased anthropogenic impact on the coastal area. The marine storm, which occurred on 28 December 2020, was analyzed through an unstructured wind–wave coupled model that takes into account the main marine weather components of the coastal setup. The model, validated with in situ data, allowed the establishment of threshold values for the most significant marine and atmospheric parameters (i.e., wind intensity and duration) beyond which an event can produce destructive effects. Finally, a first assessment of the return period of this event was evaluated using local press reports on damage to urban furniture and port infrastructures.
- Article
(16992 KB) - Full-text XML
- BibTeX
- EndNote
Impacts of storm-driven erosion and flooding are the most serious hazards being faced by coastal systems worldwide due to the strong urbanization of these areas, especially because ca. 50 % of the world's coastline is currently under pressure from excessive human development. Furthermore, according to the recent Intergovernmental Panel on Climate Change (IPCC) report (IPCC, 2021), global mean sea level rise is expected to rise 1.1 m by 2100, enhancing the effects of extreme marine events that, in the future, will probably hit increasingly wide coastal areas (Antonioli et al., 2020; Bamber et al., 2019; Aucelli et al., 2017b). Other relevant physical drivers are changes in storm and hurricane characteristics such as wave height and tracks (Buccino et al., 2020; Di Luccio et al., 2019). In the last years, additionally, global climate change has increased the intensity and frequency of coastal flooding observed in the Mediterranean due to severe storms and relative surges and often in response to the occurrence of extra tropical-like cyclones, better known as medicanes (MEDIterranean hurriCANES) (Scicchitano et al., 2021; Bakkensen, 2017; Portmann et al., 2019). Climate-change-related impacts, such as shoreline changes, under these conditions and their prediction are essential for integrated coastal zone management (Di Paola et al., 2020).
Impacts of storms on coastal areas induce relevant economic and human losses that demand better knowledge of coastal exposure and require us to reflect on the adoption of measures to reduce the impacts of these events (Costas et al., 2015). Erosive effects on the coasts are controlled by the interplay between storm characteristics and coastal geomorphology (Lionello and Scarascia, 2020). Moreover, coastal damage, strongly related to storm-induced processes (i.e., flooding or erosion), can be exacerbated by the presence of intensive human activities or other developments in residential localities such as ports or touristic infrastructures (Godschalk et al., 2000; Esnard et al., 2001; Jiménez et al., 2012).
Storm impact assessment in urbanized coastal areas in the Mediterranean Sea (Sanuy et al., 2018; Lira-Loarca et al., 2020; Amores et al., 2020; Cavaleri et al., 2019; Jiménez et al., 2018; Amarouche et al., 2020; Anfuso et al., 2021) has become an issue of high scientific and social interest due to the alarming effects related to the climate changes observed over the last decades (Young and Ribal, 2019; Lionello and Scarascia, 2020; Gulev and Grigorieva, 2004), not only in the form of an increasing trend in significant wave heights (Hs) and wind speed (Ws) (Dobrynin et al., 2012; Reguero et al., 2019; Vieira et al., 2020; Meucci et al., 2020) but also due to the accelerated relative sea level rise.
The examination of the behavior, evolution, and consequences of the coastal storm disasters is necessary to assess their danger and the population's ability to adapt and mitigate their effects (Tsai and Chen, 2011). In this respect, many authors (Van Westen, 2013) have recognized the relevance of a risk analysis based on its estimation, identification, and understanding.
To this aim, this paper deals with the characterization of a significant storm that occurred in the Gulf of Naples (Italy) that was reconstructed by analyzing weather and marine data obtained from in situ instruments and numerical simulations. These simulations, based on Weather Research and Forecasting (WRF) and Wavewatch III (WW3) wind and wave models, have been configured by the University of Naples “Parthenope” in the Gulf of Naples since 2006 (Benassai and Ascione, 2006) and have been successfully validated with in situ and satellite data (Benassai et al., 2015, 2013, 2012).
The study area exhibits a seasonal wave climate as observed along the whole coastal area of the Tyrrhenian Sea (Morucci et al., 2016; Saviano et al., 2020). Low Hs (on the order of a few tens of centimeters) is measured during the summer (from June to August), and significant waves up to 3 m are observed during winter and autumn (from November to February). However, coastal effects of the most intense winter events on a specific coastal sector depend not only on wave height and period but also storm groups and interaction of the storm waves with the bathymetry and the shoreline. Consequently, a precise assessment of the parameters that characterize a storm event is a significant challenge to better understand final effects on the coast associated with potential damages (Biolchi et al., 2019; Ferrando et al., 2021; Anfuso et al., 2016).
Therefore this paper focuses on defining and interpreting the dangerousness of the extreme storm waves that occurred in the Gulf of Naples on 28 December 2020. The numerical assessment of the storm surge on the most impacted coastal sector was carried out through a procedure, validated with in situ data, which took into account the main marine weather components of the coastal setup. The aim of this paper is twofold: on one hand to characterize the event by applying a large-scale evaluation of the marine weather variability during the maximum peak and, on the other, to define a fast procedure able to establish threshold values for the most significant marine and atmospheric parameters (i.e., wind intensity and duration) beyond which an event can produce destructive effects on human activities and coastal infrastructures. This procedure, coupled with the high-precision marine weather forecasting, provided by the network in the Gulf of Naples belonging to the University of Naples “Parthenope”, might be intended as a tool for civil protection and coastal damage prevention purposes. The approach proposed in this study can be efficiently used to define the level of sensitivity of urbanized coasts to storms (Williams et al., 2018; Molina et al., 2019, 2020).
The paper is organized as follows: Sect. 1 introduces the themes, Sect. 2 details the study area characteristics, Sect. 3 describes the methodologies, Sect. 4 presents the reconstruction of the storm event within the framework of historical wind events, Sect. 5 discusses the coastal effect of the storm surge, and Sect. 6 concludes.
The Gulf of Naples (Fig. 1), Tyrrhenian Sea, is one of the most populated Italian areas, with 3 016 762 inhabitants and a medium density of 2672 inhabitants/km2. The urban territory includes 92 municipalities on a surface of 1171 km2, with 60 % consisting of small municipalities (surface <10 km2) and an 11 % of big ones (surface >25 km2). The latter include the coastal cities of Naples, Torre Annunziata, and Pozzuoli. The urbanized area in the gulf occupies only 32.54 % of the total surface, and consequently, the population density in this area is more than 8000 inhabitants/km2. Its coasts, with an extension of 153 km, have a high cultural and natural value as demonstrated by the presence of several protected areas under different legal coverages, such as marine protected areas (marine protected areas of Punta Campanella and Regno di Nettuno) and archeological parks (underwater archeological parks of Baia and Gaiola). However, the numerous submerged archeological sites scattered along the coasts are significantly vulnerable to coastal processes (Mattei et al., 2019). Under these circumstances, the urbanized coasts of the gulf can be certainly considered highly sensitive from both a socio-cultural and economic point of view to severe marine storms. On the other hand, main cities are located in narrow coastal plains, with commercial activities and infrastructures located only a few meters above sea level (Ascione et al., 2020). In fact, the present coastal morphology in the gulf (Fig. 1) is characterized by an alternation of articulated sea cliffs with sheltered pocket beaches and narrow coastal plains, often strongly urbanized (Ascione et al., 2020; Aucelli et al., 2017a). In particular, the high-coastal sectors in the gulf can be divided into sea cliffs made of volcanic deposits typically bordered by wide shore platforms (often of polycyclic origin) and plunging cliffs in hard limestones located along the eastern side of the gulf (Aucelli et al., 2016a, b; Pappone et al., 2019; Aucelli et al., 2019; Mattei et al., 2020). The main coastal plains in the gulf, which are Fuorigrotta, Chiaia, Sebeto, and Sarno plains (filled by successions of volcanoclastic deposits), host the most populated cities in the gulf, i.e., Naples and Torre Annunziata (Romano et al., 2013; Vacchi et al., 2016; Cinque, 1991).
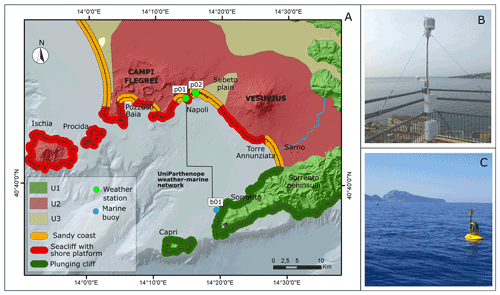
Figure 1(a) Geological and geomorphological sketch of the study area. U1: limestones, dolomites, and marls of carbonate platform units (Meso-Cenozoic); U2: volcanoclastic deposits (Quaternary); U3: alluvial, coastal, palustrine-lacustrine, and slope deposits (Quaternary). The digital terrain model (DTM) of the emerged area was downloaded from ISPRA webGIS (http://www.sinanet.isprambiente.it, last access: 1 February 2021); the DTM of the emerged area was downloaded from the GEBCO website (http://www.gebco.net, last access: 1 February 2021). Photos of the (b) weather station and (c) ondameter belong to the Parthenope University network.
From a geological point of view, the Gulf of Naples is an active peri-Tyrrhenian basin extending for about 1000 km2. It is characterized by physiographic features typical of a passive continental margin sector, with a continental shelf between −140 and −180 m of depth (Milia and Torrente, 1999, 2003). The structure of the Gulf of Naples is controlled by numerous Quaternary fault systems, NE–SW trending and SE-dipping, and NW–SE trending and SW dipping, linked to the last stages of the opening of the Tyrrhenian Sea (Fedele et al., 2015; Milia, 2010). Between the Middle and Upper Pleistocene, the fault systems were responsible for the development of the half-graben of the Gulf of Naples and Sorrento Peninsula fault block ridge (Milia and Torrente, 2003). The landscape of this area is strongly influenced by the presence of two active volcanos: the Campi Flegrei poly-caldera in the west and the Vesuvius stratovolcano in the east (Fig. 1), which interfered with its Late Pleistocene–Holocene evolution (Iannace et al., 2015; Isaia et al., 2018; Santacroce et al., 2003).
During the Holocene, the morpho-evolutive trends of the coasts of the Gulf of Naples have been characterized by sudden coastal changes strongly related to the interplay between glacio-isostatic sea level rise and volcanic forcing (Cinque et al., 2011; Aucelli et al., 2020, 2019, 2018a, b; Mattei et al., 2020). The latter was driven by the combined effects of volcanic eruptions with consequent landscape mantling by pyroclastic products and vertical ground movements of a metric entity related to sudden uplift for inflating and subsidence for deflating of the magmatic chamber. Since historical times, the anthropic impact started interfering with these natural forcings, often producing permanent modifications of the original coastal landforms (Aucelli et al., 2021; Pappone et al., 2019; Mattei et al., 2018), through mining activities and construction of port structures and infilling. However, the major forcing factor to be taken into account as the main cause of the recent coastal changes in the gulf certainly is the local wave climate. In detail, main stormy events (Menna et al., 2007) with wave height values up to 4.8 m, are associated with atmospheric low-pressure systems and occur during winter (December–February). According to Saviano et al. (2019), high-frequency radar (HFR) data show that the highest waves mainly approach from 180 to 210∘ N, thus confirming a marked southwest directionality, as expected from the local morphology of the gulf.
In contrast, in late spring and summer periods, the main wind regime is represented by breezes, with a SSW direction and maximum speed values of 8 m/s (Menna et al., 2007), that produce low wave height values ranging from 0.4 to 0.6 m (Benassai et al., 1994; Buonocore et al., 2003; Saviano et al., 2019).
Considering the seasonal surface circulation, during winter, cyclonic and anticyclonic circulation systems alternate in the gulf due to the interaction between the local wind forcing and the large-scale circulation of the Tyrrhenian Sea. In spring, when a shallow and sharp seasonal thermocline is present, coastal upwelling is recorded and generates internal waves that propagate along the coast, causing relevant mixing processes (de Ruggiero et al., 2018). In summer, the breeze forcing induces a relatively regular diurnal current oscillation (de Ruggiero et al., 2016). In this last season, surface currents typically rotated clockwise under the effect of land and sea breeze over an entire day (Uttieri et al., 2011). In autumn, the circulation is similar to the one recorded in winter.
The fast procedure proposed in this paper aims to analyze marine and atmospheric parameters (i.e., wind intensity and duration) during a marine storm to establish a threshold beyond which an event can produce destructive coastal effects (Fig. 2), according to the following steps:
-
detecting the most exposed coastal sectors during the peak of a meteo-marine event by calculating the total effect of the meteo-marine agents;
-
calculation of the wind setup in the coastal sketches where the total effect was highest;
-
calculation of the coastal setup only along with the urbanized coastal sketches with maximum values of wind setup.
The storm intensity was analyzed based on historical marine weather data coupled with the spatial modeling of barometric pressure, wind speed, and wave height and direction. This severe event strongly impacted the coast of the city of Naples, flooded wide areas, and greatly damaged the promenade and restaurants, although the significant wave height measured by the wave buoy in the gulf did not exceed 3.5 m. The examination of the duration, degree of severity, assessment of damages, and losses, reported in the local press, were compared with those of local historical chronicles to demonstrate the high return period of the event analyzed in this study.
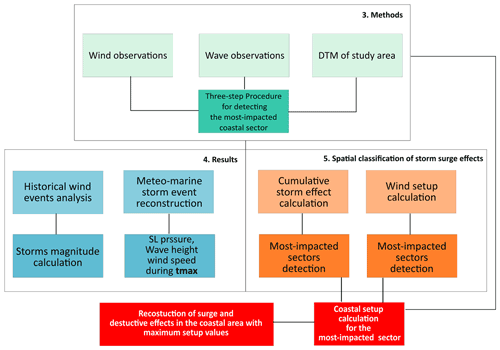
Figure 2Workflow of the procedure applied in this study to evaluate the most impacted coastal sectors in the Gulf of Naples during the December 2020 storm.
Table 1Classification in five classes of the three marine weather parameters – WSs, Hss, and SLPs – and classification of their cumulative coastal effects.
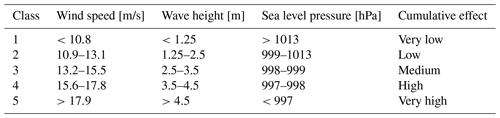
3.1 Wind observations
Data from different facilities, devoted to meteorological in situ observations, were consulted to provide a historical analysis of the wind event that affected the study area in the last 10 years and to evaluate the accuracy of the simulation results. The following weather stations located in the city of Naples were considered (see Fig. 1):
-
p01 (40∘49′55.16′′ N–14∘14′41.78′′ E) is managed by the University of Naples “Parthenope” (Fig. 1a). Since 2015, this Vaisala weather transmitter (WXT520) has measured barometric pressure, humidity, precipitation, temperature, and wind speed and direction. The Vaisala WINDCAP sensor uses ultrasound to determine horizontal wind speed and direction with an accuracy of ±0.3 m/s and ±3∘, respectively.
-
p02 (40∘50′24.46′′ N–14∘16′30.63′′ E) is part of the National Tide Gauge Network (https://www.mareografico.it/ last access: 1 February 2021), and it is managed by the Italian Institute for Environmental Protection and Research (ISPRA). The weather station is located in the Port of Naples at the Diaz pier.
Considering such databases, an analysis of wind events was applied to 2010–2020 wind records to evaluate and classify the storms that approached the southwest in the last 10 years. Therefore, the dataset was filtered for events coming from 202–242∘ directions, highlighting those with a velocity higher than 13.9 m/s (the lower limit of the “near-gale” class in the Beaufort scale) and duration >6 h (according to Allen, 1981). Subsequently, for each of the selected events, the magnitude (M) was evaluated according to the following equation:
where h is the event duration in hours, and v is the wind speed in meters per second (modified from Allen, 1981).
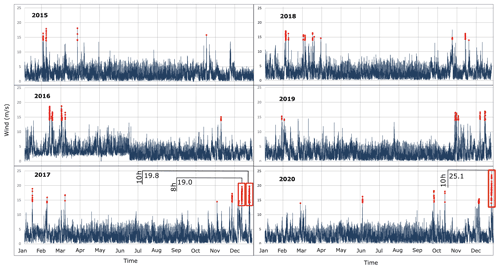
Figure 3Graph of wind speed (m/s) between 2015 and 2020 measured by the P01 weather station; red points highlight storms from 202–242∘ with velocity >13.9 m/s.
3.2 Wave observations
The in situ sea wave observation was carried out with the wave recorder b01 (40∘37′07.82′′ N–14∘19′24.60′′ E), managed by the University of Naples “Parthenope” (Fig. 1a and c). The buoy is located in the Gulf of Naples near the Vervece islet, and it has been operative since July 2020. It is equipped with a BRIZO-X directional GNSS wave height sensor to record wave statistics such as significant wave height (Hs), maximum wave height (Hm), peak wave period (Tp), mean zero upcrossing period (Tm), mean wave direction (Dm), wave spread (Ds), and wave spectra.
A subset of the dataset was used to evaluate the accuracy of the offshore wave simulations during the considered December 2020 storm event.
3.3 Atmospheric–marine numerical workflow
To characterize the meteo-marine scenario during the December 2020 storm event, an high-spatial-resolution model chain (Sánchez-Gallegos et al., 2021; Di Luccio et al., 2020b; Sánchez-Gallegos et al., 2019b, a) was configured using the workflow orchestrator DagOnStar (Montella et al., 2018) to manage and run the community numerical models Weather Research and Forecasting (WRF) (Skamarock et al., 2001; Powers et al., 2017) and Wavewatch III (WW3) (Tolman, 2009).
The first workflow component is the atmospheric model WRF, which computes the 10 m wind fields and other atmospheric parameters needed to drive the WW3 offshore wave model.
The weather pattern (e.g., 10 m wind field and sea level pressure) during the December 2020 storm was reconstructed with the WRF model with the following two-way nested computational domains: a coarser domain (25 km) covering the whole of Europe, an intermediate domain (5 km) on the Italian peninsula, and a finer domain (1 km) in the southern Tyrrhenian Sea. The WRF model initial conditions were provided by the Global Forecast System (GFS), owned by the National Centers for Environmental Prediction (NCEP).
A similar telescopic configuration was used in the case of the WW3 wave model, with a ground resolution of 0.09∘ in the whole Mediterranean Sea, an intermediate resolution of 0.03∘ for seas surrounding Italy, and a finer resolution of 0.01∘ for the southern Tyrrhenian Sea. In this case, the weather conditions provided by WRF were used to drive the marine dynamic in the Mediterranean Sea closed geographical domain, so no wave boundary condition was necessary. Moreover, an offline coupling approach between the atmospheric model WRF and the wave model WW3 was applied. The simulation results were provided with a 1 h time step in NetCDF format.
This configuration of WRF and WW3 numerical models had been already tested in other applications involving, among others, the beach run-up calculation (Di Luccio et al., 2018a, 2020b), the rip-current identification (Di Luccio et al., 2018b), and the reconstruction of weather (Di Luccio et al., 2020a, 2021; Montella et al., 2019) and marine (Castagno et al., 2020) patterns.
In the present application, the simulated wind speed (WSs) and direction (WDs), the sea level pressure (SLPs), the significant wave height (Hss), and the mean wave period (Tms) and direction (Dms) were analyzed to characterize the December 2020 storm along the whole Gulf of Naples using the maximum-resolution dataset available for the WRF and WW3 models.
3.4 Setup evaluation
The reconstruction of the main erosive effects of the maximum water level time interval (tmax) that occurred during the December 2020 storm in the study area was performed with the following methodological steps.
-
The first step is aimed at detecting the most impacted coastal sectors in the Gulf of Naples during tmax. To do this, each marine weather parameter – WSs, Hss, and SLPs – was classified, taking into account the five classes of intensity and/or impact on the coasts summarized in Table 1. Wind intensity was classified according to the Beaufort scale, also considering the range of wind variability in the gulf in the last 10 years. In particular, class 1 corresponds to a fresh breeze, class 2 is a strong breeze, classes 3 and 4 are near-gale, and class 5 is gale or higher than a gale. The wave height was classified according to the Douglas scale, where class 1 is from calm to slight wave, class 2 is a moderate wave, class 3 is a rough wave, and classes 4 and 5 are from rough to very rough. Atmospheric pressure was classified in equal intervals, starting from class 1, corresponding to high-pressure conditions, up to class 5, corresponding to low-pressure ones.
The total effect of the three classified parameters was evaluated by calculating the average values between the abovementioned classes and, consequently, classified in five classes according to Table 1.
-
The second step was the calculation of the wind setup in the coastal sketches where the total effect was high, according to the following equations (Reeve et al., 2018):
where Uw is the wind speed (m/s), h is the water depth, ρ is the density of air (“a”) or water (“w”), and Cw is the air or water friction coefficient.
The maximum setup at the downwind coast is
where F is the fetch length in meters, and ηw is the wind setup in meters.
-
The last step provided the calculation of the coastal setup as the sum of wind, wave, and barometric setup, along with the urbanized coastal sketches with maximum values of wind setup. The aim was to evaluate the flooding during tmax only where the storm had destructive effects on anthropic structures. In the surf zone, the rise in the mean water level at mean depth dx was calculated as follows (Dean and Dalrymple, 1991):
where is the wave setdown at the breaking depth, given by
where Hb is breaking wave height, and γb is the breaker index.
For spilling type breakers on dissipative beaches the assumption commonly employed is that γb remains a fixed ratio throughout the entire surf zone:
where db is the mean depth at breaking. The breaking index was calculated by various authors. According to Kamphuis (1991), the significant breaking index is given by
where m is the slope of the seabed.
The barometric setup was calculated as follows:
where ΔPa is the pressure variation during the event.
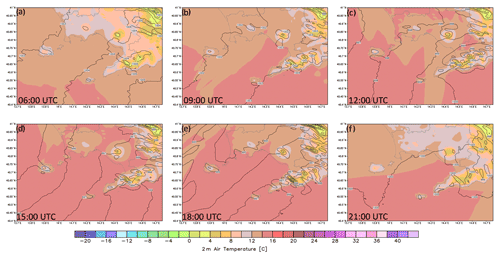
Figure 6Sea level pressure and 2 m air temperature simulated with the WRF model on 28 December 2020 at 06:00 UTC (a), 09:00 UTC (b), 12:00 UTC (c), 15:00 UTC (d), 18:00 UTC (e), and 21:00 UTC (f).
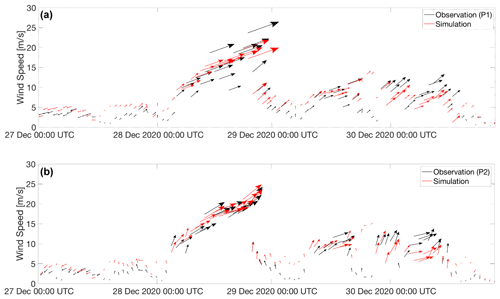
Figure 7Comparison of in situ observations of wind speed and direction at p01 (a) and p02 (b) with the simulation results.
The December 2020 storm came from the southwest, between 202 and 242∘ N. As described in the following sections, the event was characterized by anomalous wind conditions that strongly influenced effects on the coast.
4.1 Historical wind events analysis
A historical analysis of wind events coming from the southwest between 2010 and 2020 was applied to data recorded at p01 and p02 weather stations to classify the event investigated in this paper. The results reported in Figs. 3 and 4 show that the most intense events with a duration higher than 6 h (red points in the figures) come from the NW sector during winter months, confirming previous studies (Menna et al., 2007; Saviano et al., 2019, 2020).
The calculation of the storm magnitude (Fig. 5) shows that these events frequently occurred in December (7 out of 10). Moreover, the December 2020 storm is the most intense event that occurred in the observed period, in terms of both maximum wind speed measured by p01 station (25.1 m/s) and maximum duration (11 h) of wind speed >13.9 m/s (“near-gale” in Beaufort scale).
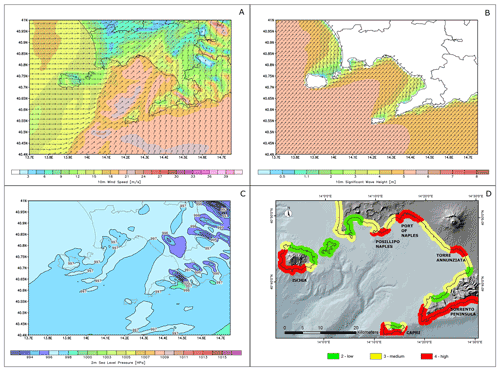
Figure 9Maps during tmax of (a) wind speed, (b) significant wave height, (c) sea level pressure, (d) the cumulative effect of the three modeled parameters. The DTM of the emerged area was downloaded from ISPRA webGIS (http://www.sinanet.isprambiente.it, last access: 1 February 2021); the DTM of the emerged area was downloaded from the GEBCO website (http://www.gebco.net/data_and_products/gridded_bathymetry_data, last access: 1 February 2021).
4.2 Meteo-marine storm event reconstruction
The scientific workflow described in Sect. 3.3 supported the reconstruction of the December 2020 storm event. As shown in Fig. 6, the study area was characterized by a low-pressure front with a minimum SLPs value equal to 995 hPa at tmax±1 h during 28 December 2020.
On the same day, this intense atmospheric low-pressure system was accompanied by widespread rainfall and strong winds coming from the southwest (WSs>20 m/s) with gusts >25 m/s, as shown in Fig. 7.
Moreover, the sea state at tmax±1 h was characterized by high waves (about 4 m as recorded by the wave recorder b01).
The combination of these and other (e.g., tide level) coastal dynamic agents caused a violent storm surge in Naples that strongly flooded the city's waterfront overnight.
The validation of the accuracy of the WRF and WW3 models was done through the comparison between the hourly numerical results in the time interval 27–30 December 2020 and the data recorded by the weather and wave recording stations (p01, p02, and b01). The results evidenced that the numerical models were in good agreement with the observations. In particular, the root mean square error (RMSE) was about 0.23 m for the significant wave height in b01 (Fig. 8) and about 3.06 and 3.35 m/s in the case of wind speed at p01 and p02, respectively.
The results of the spatial classification of the cumulative storm surge effects show that it was highest (red class) along with three cliffed urbanized sectors (Ischia, Capri, and Sorrento Peninsula) and three strongly anthropized, urban, low coastal areas (Posillipo, Port of Naples, and Torre Annunziata), as shown in Fig. 9d.
This result can be explained through the analysis of the wind and wave spatial distribution during the 28 December event in the Gulf of Naples. The wind speed in the western part of the gulf (Fig. 9a) is lower than that in the central-eastern part. In contrast, the significant wave height was maximum in the proximity of the islands (Capri and Ischia), with values higher than 5 m and between 4 and 5 m along with the other urban exposed sectors. Due to the combined effect of the spatial distribution of the fetch and the wind speed variation along the coastal areas, the cumulative effect of the 28 December storm surge was maximum among the urbanized areas most exposed to the southwest, namely the Naples coast (Posillipo and Port sectors) and Torre Annunziata.
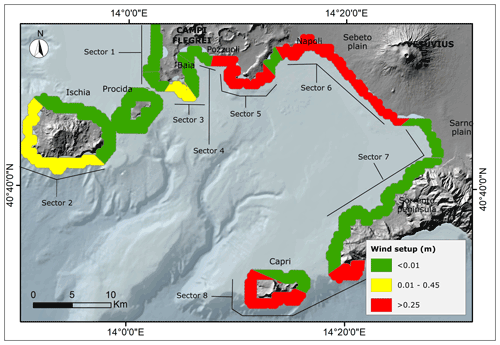
Figure 10Wind setup along the coast of Gulf of Naples during tmax. The DTM of the emerged area was downloaded from ISPRA webGIS (http://www.sinanet.isprambiente.it, last access: 1 February 2021); the DTM of the emerged area was downloaded from the GEBCO website (http://www.gebco.net, last access: 1 February 2021).
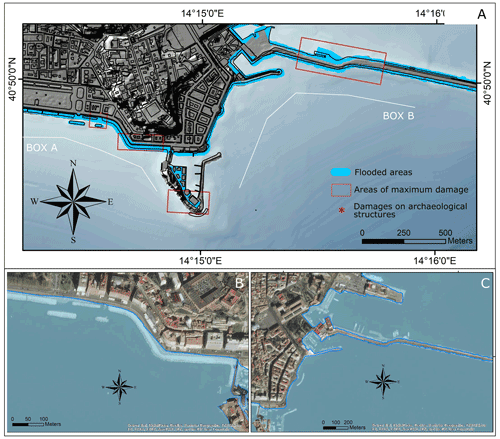
Figure 11(a) The surge in the coastal area with maximum setup values (Naples city center) during the storm event (the onshore DTM from lidar data was provided by the Ministero dell'Ambiente; the offshore DTM was derived from bathymetric data provided by Regione Campania), close-up of flooded areas in the (b) W sector and (c) E sector (Esri World Imagery basemap. Scale not given. Source: Esri, DigitalGlobe, GeoEye, Earthstar Geographics, CNES/Airbus DS, USDA, USGS, AeroGRID, IGN, and the GIS user community, 14 February 2021).
However, the Naples coast (sectors 5 and 6 in Fig. 10) was the most exposed to the wind effects, as the wind setup calculation demonstrated. Consequently, the mean coastal setup was evaluated only in this sector, according to the procedure described before. In particular, the wave setup was calculated (Eq. 2) by using the high-precision submerged bathymetry, obtained from a multibeam survey of the Neapolitan area, from which the slope in shallow waters was measured.
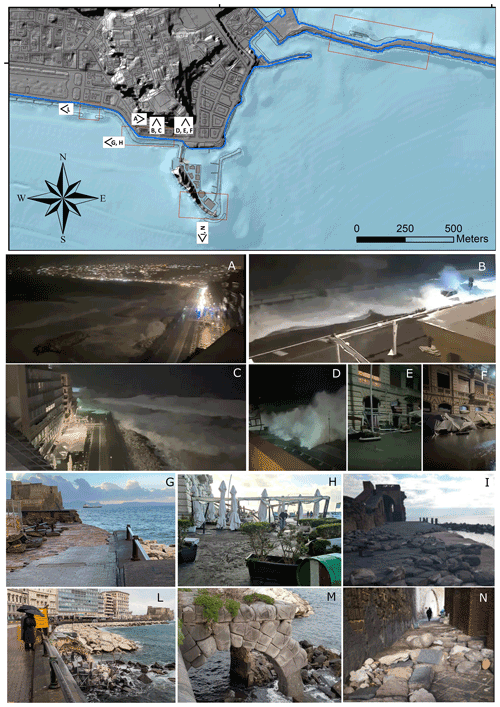
Figure 12(a–d) Photos of storm surge in the coastal area with maximum setup values (Naples city center), (e, f) damages to structures during the event, (g) promenade destroyed with collapsed parapet, (h) shattered shop windows, (i, n) Castel dell'Ovo after the event, (l, m) Bourbon pier after the event, (h) Bourbon pier before the event. On the map, the onshore DTM from lidar data was provided by the Ministero dell'Ambiente; the offshore DTM was derived from bathymetric data provided by Regione Campania.
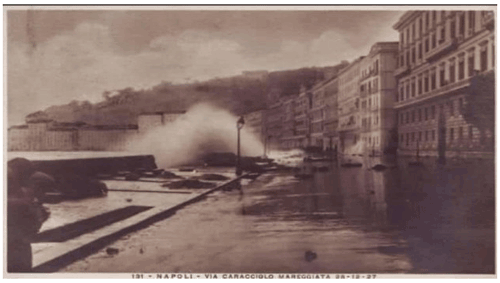
Figure 13Historical photo of the 1927 storm that impacted and destroyed via Caracciolo, Naples (from the Il Mattino newspaper).
As a result of the calculation (step 3 in Sect. 3.4), the time-averaged water level elevation at the coastline (coastal setup) during tmax in the most exposed area of the Naples waterfront was 1.6 m, resulting from the sum of wind setup (0.8 m), wave setup (0.3 m), barometric setup (0.2 m), and water level (0.3 m).
The fast water level increase during tmax produced a twofold effect on the coast, as evidenced by video and photo reports (Fig. 12). On one hand, total flooding of the coastal area took place (Fig. 11); on the other, wave breaking processes took place on the coastal promenade (Fig. 12).
The flooding produced several destructive effects that concerned the whole coastal sector, as shown in Fig. 12, including a 14th-century castle (Castel dell'Ovo; Fig. 12i and n). In the touristic city area between via Caracciolo and Castel dell’Ovo, the main damages concerned both commercial activities and archeological structures. In particular, a part of the promenade was dismantled, together with the bungalows of some restaurants and some shop windows (Fig. 12h). Regarding the cultural heritage, the destruction of the Bourbonic pier occurred (Fig. 12m), and the 19th-century pavement on the outside of Castel dell'Ovo was dismantled. The main damages to the port were related to the military infrastructures located on the San Vincenzo pier.
The return period of this event was evaluated through the analysis of historical archives of local newspapers. According to the last 100-year chronicles, based on similar damage records, the December 2020 storm surge is a marine weather event with a very high return period. The only past event with similar destructive effects occurred on 28 December 1927, as described by local newspapers (Fig. 13).
The marine storm of 28 December 2020 that affected the Gulf of Naples represented an outstanding storm associated with the persistence of wind speed up to 90 km/h and low-pressure conditions (997 hPa). This caused a significant surge with a local increase of about 1.6 m during the peak of the event. The anomalous water level rise provoked the flooding of wide coastal areas of the city of Naples, with catastrophic effects on port infrastructures, urban facilities, and cultural heritage. The very high intensity of this event was testified also by the analysis of historical chronicles that identified only one storm with similar destructive effects in December 1927. The numerical characterization of this SW storm, based on the high-spatial-resolution model, allowed the detection of the most exposed coastal sectors in the gulf, according to the first step of the proposed procedure. On the other hand, this expedited procedure resulted in an efficient way to measure the effects of this event on the urbanized areas among the most exposed sectors. The main result was the definition of threshold values for wind speed and duration, coupled with atmospheric pressure and medium wave height, which could be used as an alert for future similar events coming from the fourth quadrant. This fast procedure applied to data from the marine weather forecasting networks can become an operative tool for local authorities asked to apply protective actions to human activities. Consequently, the approach proposed in this study has the aim to limit the damages of extreme events through the two following actions: the preliminary detection of the destructive storms in time and space and the implementation of fast procedures for alerting. In the context of global warming, this issue is highly topical due to the recent and continuous increase in sea surface temperature (SST), which has the effect of exacerbating the intensity and frequency of extreme marine events on both a global and Mediterranean scale. Considering also the recent acceleration of sea level rise, urbanized and natural coastal areas are likely to be increasingly flooded. In the framework of reducing the flood risk in the coastal area, two alternative strategies can be assessed. On one hand, it is mandatory to enhance the currently poor information on the way in which local stakeholders counteract flood risks, for which local shop owners are held responsible. On the other hand, community disaster management is currently limited to closing off the waterfront at high water levels. The creation of high-resolution databases of the main marine weather characteristics of extreme events sounds strategic for improving information and taking precautionary measures. The second strategy is to improve the resilience of the urban waterfront. This can be achieved by enhancing flood resilience into existing shops, e.g., by raising the topographic level of the shop entrances and incorporating wet-proof technologies and infrastructures into the open-air utilities.
The weather and marine numerical model results are available at https://data.meteo.uniparthenope.it/opendap/opendap/ (last access: 7 October 2021; OPeNDAP, 2021).
PA and GB coordinated the paper. Specific contributions to the sections were managed as follows: data curation and analysis – GM, DDL, and GuB; methodological approach – GA, GuB, GM, and DDL; software: DDL; supervision: PA, GA, and GiB; data visualization – DDL and GM; writing of original draft and reviewed versions – GM, GA, GuB, DDL, GiB, and PA.
The contact author has declared that neither they nor their co-authors have any competing interests.
Publisher's note: Copernicus Publications remains neutral with regard to jurisdictional claims in published maps and institutional affiliations.
This work is a contribution to the Andalusia PAI research group RNM-328. It was in the framework of the project “Distretto ad alta tecnologia per i beni culturali DATABENC, PON 03PE-00164 Rete Intelligente dei Parchi Archeologici (RIPA-PAUN)” and also benefited from the discussion at the Neptune-INQUA meetings (INQUA CMP project 2003P). The activities are in the framework of the project “Use of innovative technologies, materials and models in the aeronautic field (AEROMAT)”, project identification code ARS01-01147, PON R&I 2014–2020. The authors are grateful to the Meteo@Uniparthenope (http://meteo.uniparthenope.it, last access: 7 October 2021), which is the forecast service of the University of Naples “Parthenope” and provided the hardware and software resources for the offshore numerical simulations. The authors thank Gianluigi Di Paola and the other anonymous reviewers for their critical reviews, which greatly improved the manuscript.
This paper was edited by Oded Katz and reviewed by three anonymous referees.
Allen, J. R.: Beach erosion as a function of variations in the sediment budget, Sandy Hook, New Jersey, USA, Earth Surf. Process. Landf., 6, 139–150, 1981. a, b
Amarouche, K., Akpınar, A., Cakmak, R. E., Houma, F., and Bachari, N. E. I.: Assessment of storm events along the Algiers coast and their potential impacts, Ocean Eng., 210, 107432, https://doi.org/10.1016/j.oceaneng.2020.107432, 2020. a
Amores, A., Marcos, M., Carrió, D. S., and Gómez-Pujol, L.: Coastal impacts of Storm Gloria (January 2020) over the north-western Mediterranean, Nat. Hazards Earth Syst. Sci., 20, 1955–1968, https://doi.org/10.5194/nhess-20-1955-2020, 2020. a
Anfuso, G., Rangel-Buitrago, N., Cortés-Useche, C., Iglesias Castillo, B., and Gracia, F.: Characterization of storm events along the Gulf of Cadiz (eastern central Atlantic Ocean), Int. J. Climatol., 36, 3690–3707, 2016. a
Anfuso, G., Postacchini, M., Di Luccio, D., and Benassai, G.: Coastal sensitivity/vulnerability characterization and adaptation strategies: a review, J. Marine Sci. Eng., 9, 72, https://doi.org/10.3390/jmse9010072, 2021. a
Antonioli, F., De Falco, G., Lo Presti, V., Moretti, L., Scardino, G.,Anzidei, M., Bonaldo, D., Carniel, S., Leoni, G., Furlani, S., Marsico, A., Petitta, M., Randazzo, G., Scicchitano, G., and Mastronuzzi, G.: Relative Sea-Level Rise and Potential Submersion Risk for 2100 on 16 Coastal Plains of the Mediterranean Sea, Water, 12, 2173, https://doi.org/10.3390/w12082173, 2020. a
Ascione, A., Aucelli, P. P., Cinque, A., Di Paola, G., Mattei, G., Ruello, M., Russo Ermolli, E., Santangelo, N., and Valente, E.: Geomorphology of Naples and the Campi Flegrei: human and natural landscapes in a restless land, Journal of Maps, pp. 1–11, 2020. a, b
Aucelli, P., Cinque, A., Giordano, F., and Mattei, G.: A geoarchaeological survey of the marine extension of the Roman archaeological site Villa del Pezzolo, Vico Equense, on the Sorrento Peninsula, Italy, Geoarchaeology, 31, 244–252, 2016a. a
Aucelli, P., Cinque, A., Mattei, G., and Pappone, G.: Historical sea level changes and effects on the coasts of Sorrento Peninsula (Gulf of Naples): New constrains from recent geoarchaeological investigations, Palaeogeography, Palaeoclimatology, Palaeoecology, 463, 112–125, 2016b. a
Aucelli, P., Cinque, A., Mattei, G., Pappone, G., and Stefanile, M.: Coastal landscape evolution of Naples (Southern Italy) since the Roman period from archaeological and geomorphological data at Palazzo degli Spiriti site, Quaternary Int., 483, 23–38, 2018a. a
Aucelli, P., Cinque, A., Mattei, G., Pappone, G., and Rizzo, A.: Studying relative sea level change and correlative adaptation of coastal structures on submerged Roman time ruins nearby Naples (southern Italy), Quaternary Int., 501, 328–348, 2019. a, b
Aucelli, P. P., Cinque, A., Mattei, G., and Pappone, G.: Late Holocene landscape evolution of the gulf of Naples (Italy) inferred from geoarchaeological data, J. Maps, 13, 300–310, 2017a. a
Aucelli, P. P., Cinque, A., Mattei, G., Pappone, G., and Stefanile, M.: First results on the coastal changes related to local sea level variations along the Puteoli sector (Campi Flegrei, Italy) during the historical times, Alp. Mediterr. Quat, 31, 3–16, 2018b. a
Aucelli, P. P., Mattei, G., Caporizzo, C., Cinque, A., Troisi, S., Peluso, F., Stefanile, M., and Pappone, G.: Ancient coastal changes due to ground movements and human interventions in the Roman Portus Julius (Pozzuoli Gulf, Italy): Results from photogrammetric and direct surveys, Water, 12, 658, https://doi.org/10.3390/w12030658, 2020. a
Aucelli, P. P., Mattei, G., Caporizzo, C., Cinque, A., Amato, L., Stefanile, M., and Pappone, G.: Multi-proxy analysis of relative sea-level and paleoshoreline changes during the last 2300 years in the Campi Flegrei caldera, Southern Italy, Quaternary Int., 602, 110–130, https://doi.org/10.1016/j.quaint.2021.03.03, 2021. a
Aucelli, P. P. C., Di Paola, G., Incontri, P., Rizzo, A., Vilardo, G., Benassai, G., Buonocore, B., and Pappone, G.: Coastal inundation risk assessment due to subsidence and sea level rise in a Mediterranean alluvial plain (Volturno coastal plain–southern Italy), Estuar. Coast. Shelf Sci., 198, 597–609, 2017b. a
Bakkensen, L. A.: Mediterranean hurricanes and associated damage estimates, J. Extreme Events, 4, 1750008, https://doi.org/10.1142/S2345737617500087, 2017. a
Bamber, J. L., Oppenheimer, M., Kopp, R. E., Aspinall, W. P., and Cooke, R. M.: Ice sheet contributions to future sea-level rise from structured expert judgment, P. Natl. Acad. Sci. USA, 116, 11195–11200, 2019. a
Benassai, G. and Ascione, I.: Implementation and validation of wave watch III model offshore the coastlines of Southern Italy, in: International Conference on Offshore Mechanics and Arctic Engineering, Hamburg, Germany, 47470, 553–560, 2006. a
Benassai, G., De Maio, A., and Sansone, E.: Altezze e periodi delle onde significative nel Golfo di Napoli dall’aprile 1986 al giugno 1987, Ann. IUN, 61, 3–9, 1994. a
Benassai, G., Migliaccio, M., Montuori, A., and Ricchi, A.: Wave simulations through SAR COSMO-SkyMed wind retrieval and verification with buoy data, in: The Twenty-second International Offshore and Polar Engineering Conference, OnePetro, Rhodes, Greece, 2012. a
Benassai, G., Migliaccio, M., and Montuori, A.: Sea wave numerical simulations with COSMO-SkyMed© SAR data, J. Coast. Res., 65, 660–665, 2013. a
Benassai, G., Migliaccio, M., and Nunziata, F.: The use of COSMO-SkyMed© SAR data for coastal management, J. Marine Sci. Technol., 20, 542–550, 2015. a
Biolchi, S., Denamiel, C., Devoto, S., Korbar, T., Macovaz, V., Scicchitano, G., Vilibić, I., and Furlani, S.: Impact of the October 2018 storm Vaia on coastal boulders in the northern Adriatic Sea, Water, 11, 2229, https://doi.org/10.3390/w11112229, 2019. a
Buccino, M., Di Paola, G., Ciccaglione, M. C., Del Giudice, G., and Rosskopf, C. M.: A medium-term study of Molise coast evolution based on the one-line equation and “equivalent wave” concept, Water, 12, 2831, https://doi.org/10.3390/w12102831, 2020. a
Buonocore, B., Sansone, E., and Zambardino, G.: Rilievi ondametrici nel Golfo di Napoli, Ann. IUN, 67, 203–211, 2003. a
Castagno, P., de Ruggiero, P., Pierini, S., Zambianchi, E., De Alteris, A., De Stefano, M., and Budillon, G.: Hydrographic and dynamical characterisation of the Bagnoli-Coroglio Bay (Gulf of Naples, Tyrrhenian Sea), Chem. Ecol., 36, 598–618, 2020. a
Cavaleri, L., Bajo, M., Barbariol, F., Bastianini, M., Benetazzo, A., Bertotti, L., Chiggiato, J., Davolio, S., Ferrarin, C., Magnusson, L., Papa, A., Pezzutto, P., Pomaro, A., and Umgiesser, G.: The October 29, 2018 storm in Northern Italy–an exceptional event and its modeling, Prog. Oceanogr., 178, 102178, https://doi.org/10.1016/j.pocean.2019.102178, 2019. a
Cinque, A.: La trasgressione versiliana nella Piana del Sarno (Campania), Geografia Fisica e Dinamica Quaternaria, 14, 63–71, 1991. a
Cinque, A., Irollo, G., Romano, P., Ruello, M. R., Amato, L., and Giampaola, D.: Ground movements and sea level changes in urban areas: 5000 years of geological and archaeological record from Naples (Southern Italy), Quaternary Int., 232, 45–55, 2011. a
Costas, S., Ferreira, O., and Martinez, G.: Why do we decide to live with risk at the coast?, Ocean Coast. Manage., 118, 1–11, 2015. a
de Ruggiero, P., Napolitano, E., Iacono, R., and Pierini, S.: A high-resolution modelling study of the circulation along the Campania coastal system, with a special focus on the Gulf of Naples, Cont. Shelf Res., 122, 85–101, 2016. a
de Ruggiero, P., Napolitano, E., Iacono, R., Pierini, S., and Spezie, G.: A baroclinic coastal trapped wave event in the Gulf of Naples (Tyrrhenian Sea), Ocean Dynam., 68, 1683–1694, 2018. a
Dean, R. G. and Dalrymple, R. A.: Water wave mechanics for engineers and scientists, vol. 2, World Scientific Publishing Company, Singapore, 1991. a
Di Luccio, D., Benassai, G., Budillon, G., Mucerino, L., Montella, R., and Pugliese Carratelli, E.: Wave run-up prediction and observation in a micro-tidal beach, Nat. Hazards Earth Syst. Sci., 18, 2841–2857, https://doi.org/10.5194/nhess-18-2841-2018, 2018.a. a
Di Luccio, D., Benassai, G., Di Paola, G., Rosskopf, C. M., Mucerino, L., Montella, R., and Contestabile, P.: Monitoring and modelling coastal vulnerability and mitigation proposal for an archaeological site (Kaulonia, Southern Italy), Sustainability, 10, 2017, https://doi.org/10.3390/su10062017, 2018b. a
Di Luccio, D., Benassai, G., Di Paola, G., Mucerino, L., Buono, A., Rosskopf, C. M., Nunziata, F., Migliaccio, M., Urciuoli, A., and Montella, R.: Shoreline rotation analysis of embayed beaches by means of in situ and remote surveys, Sustainability, 11, 725, https://doi.org/10.3390/su11030725, 2019. a
Di Luccio, D., Benassai, G., de Stefano, M., and Montella, R.: Evidences of atmospheric pressure drop and sea level alteration in the Ligurian Sea, in: 2019 IMEKO TC19 International Workshop on Metrology for the Sea: Learning to Measure Sea Health Parameters, MetroSea 2019, 22–27, 2020a. a
Di Luccio, D., Benassai, G., Mucerino, L., Montella, R., Conversano, F., Pugliano, G., Robustelli, U., and Budillon, G.: Characterization of beach run-up patterns in Bagnoli bay during ABBACO project, Chem. Ecol., 36, 619–636, 2020b. a, b
Di Luccio, D., Buono, A., Corcione, V., Migliaccio, M., and Benassai, G.: An integrated approach of in-situ data, remote sensing measurements and numerical simulations to study storm events in the Ligurian Sea, 2020 IMEKO TC-19 International Workshop on Metrology for the Sea, 5–7 October 2020, Naples, Italy, 28–33, 2021. a
Di Paola, G., Ciccaglione, M., Buccino, M., and Rosskopf, C.: Influence of hard defence structures on shoreline erosion along Molise coast (southern Italy): A preliminary investigation, Rendiconti Online Societa Geologica Italiana, 52, 2–11, 2020. a
Dobrynin, M., Murawsky, J., and Yang, S.: Evolution of the global wind wave climate in CMIP5 experiments, Geophys. Res. Lett., 39, 2012. a
Esnard, A.-M., Brower, D., and Bortz, B.: Coastal Hazards and the Built Environment on Barrier Islands: A Retrospective View of Nags Head n the Late 1990s, Coastal Manage., 29, 53–72, 2001. a
Fedele, L., Morra, V., Perrotta, A., Scarpati, C., Putignano, M., Orrù, P., Schiattarella, M., Aiello, G., D'Argenio, B., and Conforti, A.: Note illustrative della Carta Geologica d'Italia alla scala 1:50.000, foglio 465 Isola di Procida, Istituto Superiore per la Protezione e la Ricerca Ambientale, Servizio Geologico d'Italia, available at: https://www.isprambiente.gov.it/Media/carg/note_illustrative/465_Isola_di_Procida.pdf (last access: 7 October 2021), 1, 204, 2015. a
Ferrando, I., Brandolini, P., Federici, B., Lucarelli, A., Sguerso, D., Morelli, D., and Corradi, N.: Coastal Modification in Relation to Sea Storm Effects: Application of 3D Remote Sensing Survey in Sanremo Marina (Liguria, NW Italy), Water, 13, 1040, https://doi.org/10.3390/w13081040, 2021. a
Godschalk, D. R., Norton, R., Richardson, C., and Salvesen, D.: Avoiding coastal hazard areas: Best state mitigation practices, Environ. Geosci., 7, 13–22, 2000. a
Gulev, S. K. and Grigorieva, V.: Last century changes in ocean wind wave height from global visual wave data, Geophys. Res. Lett., 31, 2004. a
Iannace, A., Merola, D., Perrone, V., Amato, A., Cinque, A., Santacroce, R., Sbrana, A., Sulpizio, R., Budillon, F., Conforti, A., and D'Argenio, B.: Note illustrative della carta geologica d'Italia alla scala 1:50.000 Foglio 466–485 Sorrento-Termini, Servizio Geologico d'Italia, ISPRA, available at: https://www.isprambiente.gov.it/Media/carg/note_illustrative/466_485_Sorrento_Termini.pdf (last access: 7 October 2021), p. 204, 2015. a
IPCC: Climate Change 2021: The Physical Science Basis. Contribution of Working Group I to the Sixth Assessment Report of the Intergovernmental Panel on Climate Change, edited by: Masson-Delmotte, V., Zhai, P., Pirani, A., Connors, S. L., Péan, C., Berger, S., Caud, N., Chen, Y., Goldfarb, L., Gomis, M. I., Huang, M., Leitzell, K., Lonnoy, E., Matthews, J. B. R., Maycock, T. K., Waterfield, T., Yelekçi, O., Yu, R., and Zhou, B., Cambridge University Press, in press, 2021.
Isaia, R., Iannuzzi, E., Sbrana, A., and Marianelli, P.: Note Illustrative della Carta Geologica d’Italia alla scala 1:50.000, Foglio 446-447 Napoli (aree emerse), available at: https://www.isprambiente.gov.it/Media/carg/note_illustrative/446_447_Napoli.pdf (last access: 7 October 2021), 2018. a
Jiménez, J., Sanuy, M., Ballesteros, C., and Valdemoro, H.: The Tordera Delta, a hotspot to storm impacts in the coast northwards of Barcelona (NW Mediterranean), Coastal Eng., 134, 148–158, 2018. a
Jiménez, J. A., Sancho-García, A., Bosom, E., Valdemoro, H. I., and Guillén, J.: Storm-induced damages along the Catalan coast (NW Mediterranean) during the period 1958–2008, Geomorphology, 143, 24–33, 2012. a
Kamphuis, J.: Wave transformation, Coastal Eng., 15, 173–184, 1991. a
Lionello, P. and Scarascia, L.: The relation of climate extremes with global warming in the Mediterranean region and its north versus south contrast, Reg. Environ. Change, 20, 1–16, 2020. a, b
Lira-Loarca, A., Cobos, M., Losada, M. Á., and Baquerizo, A.: Storm characterization and simulation for damage evolution models of maritime structures, Coastal Eng., 156, 103620, https://doi.org/10.1016/j.coastaleng.2019.103620, 2020. a
Mattei, G., Troisi, S., Aucelli, P. P., Pappone, G., Peluso, F., and Stefanile, M.: Sensing the submerged landscape of Nisida Roman Harbour in the Gulf of Naples from integrated measurements on a USV, Water, 10, 1686, https://doi.org/10.3390/w10111686, 2018. a
Mattei, G., Rizzo, A., Anfuso, G., Aucelli, P., and Gracia, F.: A tool for evaluating the archaeological heritage vulnerability to coastal processes: The case study of Naples Gulf (southern Italy), Ocean Coast. Manage., 179, 104876, https://doi.org/10.1016/j.ocecoaman.2019.104876, 2019. a
Mattei, G., Aucelli, P. P., Caporizzo, C., Rizzo, A., and Pappone, G.: New geomorphological and historical elements on morpho-evolutive trends and relative sea-level changes of naples coast in the last 6000 years, Water, 12, 2651, https://doi.org/10.3390/w12092651, 2020. a, b
Menna, M., Mercatini, A., Uttieri, M., Buonocore, B., and Zambianchi, E.: Wintertime transport processes in the Gulf of Naples investigated by HF radar measurements of surface currents, Il nuovo cimento C, 30, 605–622, 2007. a, b, c
Meucci, A., Young, I. R., Aarnes, O. J., and Breivik, Ø.: Comparison of wind speed and wave height trends from twentieth-century models and satellite altimeters, J. Climate, 33, 611–624, 2020. a
Milia, A.: The stratigraphic signature of volcanism off Campi Flegrei (Bay of Naples, Italy), Geological Society of America Special Papers, 464, 155–170, 2010. a
Milia, A. and Torrente, M.: Tectonics and stratigraphic architecture of a peri-Tyrrhenian half-graben (Bay of Naples, Italy), Tectonophysics, 315, 301–318, 1999. a
Milia, A. and Torrente, M.: Late-Quaternary volcanism and transtensional tectonics in the Bay of Naples, Campanian continental margin, Italy, Mineral. Petrol., 79, 49–65, 2003. a, b
Molina, R., Manno, G., Lo Re, C., Anfuso, G., and Ciraolo, G.: Storm energy flux characterization along the Mediterranean coast of Andalusia (Spain), Water, 11, 509, https://doi.org/10.3390/w11030509, 2019. a
Molina, R., Manno, G., Re, C. L., Anfuso, G., and Ciraolo, G.: A Methodological Approach to Determine Sound Response Modalities to Coastal Erosion Processes in Mediterranean Andalusia (Spain), J. Marine Sci. Eng., 8, 154, https://doi.org/10.3390/jmse8030154, 2020. a
Montella, R., Di Luccio, D., and Kosta, S.: DagOn*: Executing direct acyclic graphs as parallel jobs on anything, in: 2018 IEEE/ACM Workflows in Support of Large-Scale Science (WORKS), IEEE, Dallas, TX, USA, 64–73, 2018. a
Montella, R., Di Luccio, D., Ciaramella, A., and Foster, I.: StormSeeker: A Machine-Learning-Based Mediterranean Storm Tracer, in: International Conference on Internet and Distributed Computing Systems, 444–456, Springer, Naples, Italy, 2019. a
Morucci, S., Picone, M., Nardone, G., and Arena, G.: Tides and waves in the Central Mediterranean Sea, J. Operational Oceanogr., 9, s10–s17, 2016. a
OPeNDAP: OPeNDAP Hyrax (1.13.5), available at: https://data.meteo.uniparthenope.it/opendap/opendap/ (last access: 7 October 2021.
Pappone, G., Aucelli, P. P., Mattei, G., Peluso, F., Stefanile, M., and Carola, A.: A detailed reconstruction of the Roman landscape and the submerged archaeological structure at “Castel dell'Ovo islet” (Naples, Southern Italy), Geosciences, 9, 170, https://doi.org/10.3390/geosciences9040170, 2019. a, b
Portmann, R., González-Alemán, J. J., Sprenger, M., and Wernli, H.: How an uncertain short-wave perturbation on the North Atlantic wave guide affects the forecast of an intense Mediterranean cyclone (Medicane Zorbas), Weather Clim. Dynam., 1, 597–615, https://doi.org/10.5194/wcd-1-597-2020, 2020. a
Powers, J. G., Klemp, J. B., Skamarock, W. C., Davis, C. A., Dudhia, J., Gill, D. O., Coen, J. L., Gochis, D. J., Ahmadov, R., Peckham, S. E., Grell, G. A., Michalakes, J., Trahan, S., Benjamin, S. G., Alexander, C. R., Dimego, G. J., Wang, W., Schwartz, C. S., Romine, G. S., Liu, Z., Snyder, C., Chen, F., Barlage, M. J., Yu, W., and Duda, M. G.: The weather research and forecasting model: Overview, system efforts, and future directions, B. Am. Meteorol. Soc., 98, 1717–1737, 2017. a
Reeve, D., Chadwick, A., and Fleming, C.: Coastal engineering: processes, theory and design practice, CRC Press, Boca Raton, 2018. a
Reguero, B. G., Losada, I. J., and Méndez, F. J.: A recent increase in global wave power as a consequence of oceanic warming, Nat. Commun., 10, 1–14, 2019. a
Romano, P., Di Vito, M., Giampaola, D., Cinque, A., Bartoli, C., Boenzi, G., Detta, F., Di Marco, M., Giglio, M., Iodice, S., et al.: Intersection of exogenous, endogenous and anthropogenic factors in the Holocene landscape: A study of the Naples coastline during the last 6000 years, Quaternary Int., 303, 107–119, 2013. a
Sánchez-Gallegos, D. D., Di Luccio, D., Gonzalez-Compean, J., and Montella, R.: A Microservice-Based Building Block Approach for Scientific Workflow Engines: Processing Large Data Volumes with DagOnStar, in: 2019 15th International Conference on Signal-Image Technology & Internet-Based Systems (SITIS), pp. 368–375, IEEE, 2019a. a
Sánchez-Gallegos, D. D., Di Luccio, D., Gonzalez-Compean, J. L., and Montella, R.: Internet of Things orchestration using DagOn* workflow engine, in: 2019 IEEE 5th World Forum on Internet of Things (WF-IoT), 95–100, IEEE, Limerick, Ireland, 2019b. a
Sánchez-Gallegos, D. D., Di Luccio, D., Kosta, S., Gonzalez-Compean, J., and Montella, R.: An efficient pattern-based approach for workflow supporting large-scale science: The DagOnStar experience, Future Generation Computer Systems, 122, 187–203, 2021. a
Santacroce, R., Sbrana, A., Sulpizio, R., Zanchetta, G., Perrone, V., and Bravi, S.: Carta geologica del Vesuvio, 1:15 000 Progetto CARG, Servizio Geologico Nazionale, CNR, available at: https://www.isprambiente.gov.it/Media/carg/note_illustrative/448_Ercolano.pdf (last access: 7 October 2021), 2003. a
Sanuy, M., Duo, E., Jäger, W. S., Ciavola, P., and Jiménez, J. A.: Linking source with consequences of coastal storm impacts for climate change and risk reduction scenarios for Mediterranean sandy beaches, Nat. Hazards Earth Syst. Sci., 18, 1825–1847, https://doi.org/10.5194/nhess-18-1825-2018, 2018. a
Saviano, S., Kalampokis, A., Zambianchi, E., and Uttieri, M.: A year-long assessment of wave measurements retrieved from an HF radar network in the Gulf of Naples (Tyrrhenian Sea, Western Mediterranean Sea), J. Operational Oceanogr., 12, 1–15, 2019. a, b, c
Saviano, S., Cianelli, D., Zambianchi, E., Conversano, F., and Uttieri, M.: An integrated reconstruction of the multiannual wave pattern in the Gulf of Naples (South-Eastern Tyrrhenian Sea, Western Mediterranean Sea), J. Marine Sci. Eng., 8, 372, https://doi.org/10.3390/jmse8050372, 2020. a, b
Scicchitano, G., Scardino, G., Monaco, C., Piscitelli, A., Milella, M., De Giosa, F., and Mastronuzzi, G.: Comparing impact effects of common storms and Medicanes along the coast of south-eastern Sicily, Marine Geol., 439, 106 556, 2021. a
Skamarock, W. C., Klemp, J. B., and Dudhia, J.: Prototypes for the WRF (Weather Research and Forecasting) model, in: Preprints, Ninth Conf. Mesoscale Processes, J11–J15, Amer. Meteorol. Soc., Fort Lauderdale, FL, 2001. a
Tolman, H. L.: User manual and system documentation of WAVEWATCH III TM version 3.14, Technical note, MMAB Contribution, 276, 220, 2009. a
Tsai, C.-H. and Chen, C.-W.: The establishment of a rapid natural disaster risk assessment model for the tourism industry, Tourism management, 32, 158–171, 2011. a
Uttieri, M., Cianelli, D., Nardelli, B. B., Buonocore, B., Falco, P., Colella, S., and Zambianchi, E.: Multiplatform observation of the surface circulation in the Gulf of Naples (Southern Tyrrhenian Sea), Ocean Dynam., 61, 779–796, 2011. a
Vacchi, M., Marriner, N., Morhange, C., Spada, G., Fontana, A., and Rovere, A.: Multiproxy assessment of Holocene relative sea-level changes in the western Mediterranean: Sea-level variability and improvements in the definition of the isostatic signal, Earth-Sci. Rev., 155, 172–197, 2016. a
Van Westen, C. J.: Remote sensing and GIS for natural hazards assessment and disaster risk management, Treatise on geomorphology, 3, 259–298, 2013. a
Vieira, F., Cavalcante, G., and Campos, E.: Analysis of wave climate and trends in a semi-enclosed basin (Persian Gulf) using a validated SWAN model, Ocean Eng., 196, 106821, https://doi.org/10.1016/j.oceaneng.2019.106821, 2020. a
Williams, A., Rangel-Buitrago, N., Pranzini, E., and Anfuso, G.: The management of coastal erosion, Ocean Coast. Manage., 156, 4–20, 2018. a
Young, I. R. and Ribal, A.: Multiplatform evaluation of global trends in wind speed and wave height, Science, 364, 548–552, 2019. a