the Creative Commons Attribution 4.0 License.
the Creative Commons Attribution 4.0 License.
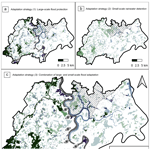
Low-regret climate change adaptation in coastal megacities – evaluating large-scale flood protection and small-scale rainwater detention measures for Ho Chi Minh City, Vietnam
Leon Scheiber
Christoph Gabriel David
Mazen Hoballah Jalloul
Jan Visscher
Hong Quan Nguyen
Roxana Leitold
Javier Revilla Diez
Torsten Schlurmann
Urban flooding is a major challenge for many megacities in low-elevation coastal zones (LECZs), especially in Southeast Asia. In these regions, the effects of environmental stressors overlap with rapid urbanization, which significantly aggravates the hazard potential. Ho Chi Minh City (HCMC) in southern Vietnam is a prime example of this set of problems and therefore a suitable case study to apply the concept of low-regret disaster risk adaptation as defined by the Intergovernmental Panel on Climate Change (IPCC). In order to explore and evaluate potential options of hazard mitigation, a hydro-numerical model was employed to scrutinize the effectiveness of two adaptation strategies: (1) a classic flood protection scheme including a large-scale ring dike as currently constructed in HCMC and (2) the widespread installation of small-scale rainwater detention as envisioned in the framework of the Chinese Sponge City Program (SCP). A third adaptation scenario (3) assesses the combination of both approaches (1) and (2).
From a hydrological point of view, the reduction in various flood intensity proxies that were computed within this study suggests that large-scale flood protection is comparable but slightly more effective than small-scale rainwater storage: for instance, the two adaptation options could reduce the normalized flood severity index (INFS), which is a measure combining flood depth and duration, by 17.9 % and 17.7 %, respectively. The number of flood-prone manufacturing firms that would be protected after adaptation, in turn, is nearly 2 times higher for the ring dike than for the Sponge City approach. However, the numerical results also reveal that both response options can be implemented in parallel, not only without reducing their individual effectiveness but also complementarily with considerable added value. Additionally, from a governance perspective, decentralized rainwater storage conforms ideally to the low-regret paradigm: while the existing large-scale ring dike depends on a binary commitment (to build or not to build), decentralized small- and micro-scale solutions can be implemented gradually (for example through targeted subsidies) and add technical redundancy to the overall system. In the end, both strategies are highly complementary in their spatial and temporal reduction in flood intensity. Local decision-makers may hence specifically seek combined strategies, adding to singular approaches, and design multi-faceted adaptation pathways in order to successfully prepare for a deeply uncertain future.
- Article
(6440 KB) - Full-text XML
- Companion paper
-
Supplement
(9062 KB) - BibTeX
- EndNote
Between 1980 and 2009, floods caused more than half a million deaths and affected another 2.8 billion people worldwide (Doocy et al., 2013). These figures will further increase with global sea levels rising as a consequence of climate change (IPCC, 2019) and more than half of all urban agglomerations (> 100 000 habitants) being closer than 100 km from the coastline (Barragán and de Andrés, 2015). Especially in Southeast Asia, where many megacities are located in low-elevation coastal zones (LECZs), the risk of storm surges will significantly increase (McGranahan et al., 2007; Neumann et al., 2015; Kulp and Strauss, 2019). Not only will these regions be affected by more frequent extreme storm events, but rainfall volumes will also increase as a consequence of the urban heat island (UHI) effect fueled by sustained urban growth (IPCC, 2021). Ranked fifth place in a global assessment of future exposure to climate extremes, Ho Chi Minh City (HCMC; Fig. 1a, b) is a prime example of the complex physical and social interactions that exacerbate the flood risk in many Southeast Asian metropolises (Hanson et al., 2011; Hallegatte et al., 2013; Abidin et al., 2015). Being the largest city in Vietnam and its major economical hub, HCMC is subject to uncontrolled urban sprawl, which in turn deepens the exposure to flooding for a growing and oftentimes highly vulnerable population (Huong and Pathirana, 2013; Duy et al., 2018). Flood exposure, in this context, results from the increasing number of settlements in low-lying areas (cf. Fig. 1c) which continuously expand due to the humanmade problem of land subsidence resulting from soil compaction and groundwater exploitation (Kaneko and Toyota, 2011; Erkens et al., 2015; Duffy et al., 2020).
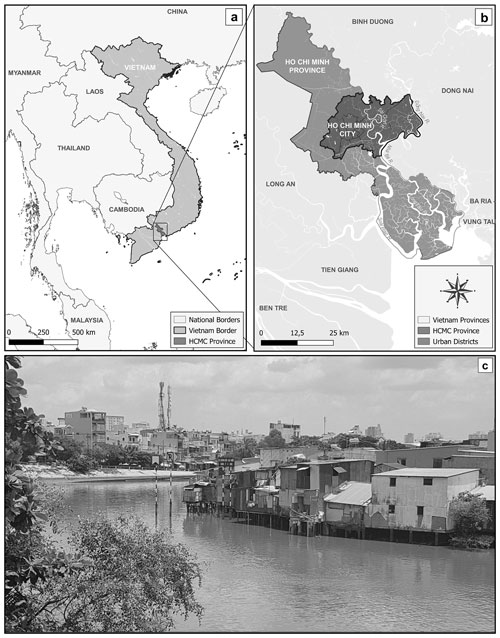
Figure 1Study area. (a, b) Located in proximity to the Mekong Delta, Ho Chi Minh City (HCMC) is the largest city in Vietnam and a major economical hub of Southeast Asia. Administrative boundaries and waterbodies derived from © OpenStreetMap contributors 2022, distributed under the Open Data Commons Open Database License (ODbL) v1.0. (c) Informal settlements are the epitome of the uncontrolled urban sprawl that exacerbates flood risk in this region due to an interplay of increased natural hazards, exposure and vulnerability. Picture taken by the corresponding author in September 2019.
In conjunction with globally rising sea levels and amplified tidal ranges in the adjacent Saigon River, HCMC regularly experiences backwater effects in the antiquated and, in many regards, deficient drainage system causing widespread inundations (Phi, 2007; Downes and Storch, 2014; Tran Ngoc et al., 2016; MONRE, 2016). Apart from the immanent social implications, urban flood events cause major and frequent impediments to the local economy, which is estimated to cause losses of USD 48.3 billion of the gross domestic product (GDP) in the period from 2006 to 2050 (ADB, 2010). Especially manufacturing firms (owning, among others, lots of immovable machinery and facilities) are expected to bear the brunt of damage and loss due to environmental hazards (Neise et al., 2018). Already in 2015, studies suggested that more than 15 % of the manufacturing firms in HCMC were located in current or future inundation areas. Many of these were small and medium-sized enterprises (SMEs) that engage about 37 % of all national employees (Leitold and Revilla Diez, 2019). Even if the city has prospered for several decades and flood control is no longer a question of preventing casualties due to extreme events, suitable adaptation strategies are required to reduce risks and associated financial losses from those recurring floods in particular.
According to the Intergovernmental Panel on Climate Change (IPCC), there are, in general, five categories of possible adaptation options to future risks: retreat, protection, accommodation, advance and ecosystem-based adaptation (https://www.ipcc.ch/srocc/chapter/chapter-4-sea-level-rise-and-implications-for-low-lying-islands-coasts-and-communities/4-1synthesis/4-1-2future-sea-level-rise-and-implications-for-responses/ipcc-srocc-ch_4_box_4_3_figure_1/, last access: 12 June 2023); IPCC, 2019). These strategies can also be differentiated into classic “grey” infrastructure and nature-based “green” solutions (Dong et al., 2017; Morris et al., 2018). One recent example of the latter approach is the Chinese Sponge City Program (SCP), a framework which refines established concepts like water-sensitive urban drainage (WSUD) and low-impact development (LID) (Jia et al., 2017; Qi et al., 2020; Sun et al., 2020; Li and Zhang, 2022). To address the predicament of increasing natural hazards in expanding urbanized areas, Sponge Cities make use of the natural hydrological cycle to effectively reduce urban flooding, harvest rainwater and improve water quality as well as restore ecological values (Köster, 2021; Jia et al., 2022). In practice, this can be accomplished by medium- and small-scale elements that allow for water storage (detention basins and rain tanks), infiltration (permeable pavements and infiltration wells) or both (public parks and rooftop/rain gardens) (Nguyen et al., 2019; Kumar et al., 2021). Since its introduction to the public in 2013, the Sponge City Program has proved its feasibility for multiple real-world examples across China (Hawken et al., 2021; Yin et al., 2022). Yet, especially its adaptability and possible small-scale implementation make it highly attractive to other rapidly growing megacities in Southeast Asia. Nevertheless, the aim of both grey and green adaptation strategies should be to offer a solution that is of low regret. In the context of the IPCC, the term “low-regret” does not necessarily refer to financial aspects but rather aims at solutions that allow for coping with current challenges and hazards without impairing future options to deal with climate change effects (IPCC, 2012). In contrast, actions that alleviate current adaptation needs but have a negative effect on future adaptation pathways are “maladaptive” (IPCC, 2014). From a sociopolitical perspective, adverse or deliberately false decisions leading to (recurrent) maladaptive actions are called maldevelopment (David et al., 2021). Maldevelopment is followed by continuously degrading conditions with the risk of reaching and exceeding anthropogenic tipping points – critical thresholds, from which changes to a system cannot be reversed (Duvat and Magnan, 2019). Given these significant consequences of actions and the wide range of climate change projections, suitable means of adaptation are preceded by robust decisions. With regard to low-regret adaptation, robust decision-making does not aim at providing solutions for all future scenarios but rather lays the foundation and does not neglect to account for a variety of possible impacts (Marchau et al., 2019). In this context, climate adaptation builds on a more flexible strategy, including scenario-based pathways and an “agree-on-decisions” approach rather than the more traditional “predict-then-act” approach (Lempert, 2019). With regard to flooding in Southeast Asian metropolises, low-regret flood adaptation should be based on multiple options which consider the trajectories of natural hazards, like sea level rise and the concentration of extreme rainfall, on the one hand, and anthropogenic factors, such as uncontrolled urban growth and humanmade land subsidence, on the other.
For the particular case of HCMC, few authors investigated the potential of available, grey or green, flood adaptation options within the last years. Most prominently, the Vietnam Climate Adaptation Partnership (VCAPS) consortium presented an extensive flood protection scheme that comprises various, mostly classic, adaptation measures (VCAPS, 2013). Supported by multiple flood gates and six major pumping stations, a large-scale ring dike prevents water from the Saigon River from entering the inner-city canal system and, thus, literally decouples the city center from the natural hydrological regime of the Saigon River. On this basis, annual damage for five different extreme events under the aforementioned adaptation strategy could be estimated by implementing the individual infrastructural elements into a hydro-numerical model of one particularly flood-prone area (Lasage et al., 2014). A comprehensive evaluation of the suggested adaptation measures and their combination was subsequently published modeling present and future storm surges for the complete city area and providing a detailed cost–benefit analysis (Scussolini et al., 2017). The study concludes with a map of proposed adaptation pathways for Ho Chi Minh City. Construction of the ring dike was, in the meantime, ordered by the Vietnamese Ministry of Agriculture and Rural Development (MARD) in 2016. Besides infrastructural flood responses, the importance of governance decisions such as reasonable land use planning was emphasized to minimize the exposure and allow for the vulnerability of the ever-growing urban population (Downes and Storch, 2014). Another recent study focused on surveying and discussing examples of ecosystem-based flood adaptation in terms of sustainable urban drainage systems (SUDSs) (Loc et al., 2015).
In summary, nearly all suggested flood adaptations for HCMC originate from recent work of the VCAPS consortium, and their effectiveness has been thoroughly tested for the case of extreme storm surges with return periods of up to 1000 years. However, hardly any research has been conducted to evaluate the performance of the proposed grey solutions, such as the ring dike, or any ecosystem-based approaches with respect to typical “every-year” events, which is in stark contrast to their 100-fold estimated GDP loss expected by the Asian Development Bank (ADB, 2010). Moreover, heavy rainfall has been completely disregarded in the majority of studies, although precipitation-based damage should demonstrably be an essential part of all urban flood risk assessments (Rözer et al., 2019). Finally, only a few working groups have scrutinized the combination of grey and green flood adaptation measures (Hamel and Tan, 2022).
To address this gap in current research, the presented study scrutinizes the hydraulic effectiveness of grey and green flood adaptation measures under the influence of a representative flood event, i.e., tidal water levels between −1.6 and +1.2 m above sea level in combination with the official design storm with a 1-year return period and 3 h duration (T = 1 year; D = 180 min). By employing a simple yet robust surface runoff model, the focus is set on the following objectives:
-
to explore the feasibility and juxtapose the hydraulic effects of the two aforementioned adaptation concepts given the case of HCMC;
-
to quantify the potential reduction in flood intensity, expressed through conventional and integrated proxies, that is achievable by each adaptation strategy and their combined implementation;
-
to evaluate their low-regret character (including tentative co-benefits) and discuss the implications for decision-makers.
Classic, grey adaptation measures in this study follow the concept of the large-scale ring dike as envisioned by VCAPS. Decentralized, green flood adaptation, on the other hand, is represented by small-scale rainwater detention as realized in the context of the Chinese Sponge City Program. Not only do these two approaches differ in terms of their size and hydraulic working principles, but the large-scale ring dike is also characterized by its binary realization, while the Sponge City concept is inherently modular. It is the aim of this study to juxtapose these two strategies both from a hydrological and a governance point of view, elucidating the practical validity of the IPCC's low-regret paradigm for flood protection and disaster risk reduction in associated rainfall events.
To assess the hydraulic effectiveness of various adaptation strategies, a simplified 2D flow model, designed in HEC-RAS (Hydrologic Engineering Center River Analysis System) version 6.0 by the U.S. Army Corps of Engineers (USACE, 2021), was used to simulate rainwater-induced inundations in HCMC. The key principle behind the employed modeling scheme is that precipitation volumes typically accumulate across the urban topography and finally discharge into the local canal system (physically based rainfall runoff modeling). HEC-RAS was first introduced in 1995 and, since then, has been validated in numerous case studies in comparable settings (Patel et al., 2017; Muthusamy et al., 2019; Rangari et al., 2019; Yalcin, 2020). As a consequence of the availability of local data, the complete setup of the utilized model is based on open-access input data in the form of freely available topography and hydro-meteorological conditions. The computational grid comprises about 1.6 million rectangular cells of ca. 35 m × 35 m resolution with combined elevation data from the Shuttle Radar Topography Mission (Farr et al., 2007) and the CoastalDEM (digital elevation model; Kulp and Strauss, 2018). On this basis, the model domain is defined by the local terrain as it contains all catchments that potentially contribute to surface runoff within the city boundaries (cf. Fig. 2a). Upstream influxes are given by the long-term mean discharges of the Saigon (MQ = 54 m3 s−1) and Dong Nai (MQ = 890 m3 s−1) rivers, respectively (Tran Ngoc et al., 2016). The downstream boundary condition in the south of the city was obtained by extrapolating coastal water levels from a global database (Caldwell et al., 2015) in accordance with hydrological reports for this catchment (Gugliotta et al., 2019). The model boundaries are complemented by uniformly distributed rainfall with a hyetograph that follows an official 3 h design storm and can be readily adapted for arbitrary return periods (cf. Fig. 2b). As there is hardly any public information about the quality and condition of the existing drainage system in HCMC – except that its capacity is regularly overloaded during extreme events – all rainfall is assumed to become gradient-controlled surface runoff as is a common conservative estimate (e.g., Scussolini et al., 2017). The resulting flow paths resemble the course of the physical drainage system, including site-specific backwater effects, so that flooding hotspots can be reproduced reliably. The runoff model was validated against locally reported inundations from 25 different locations across HCMC (documenting a storm event in June 2010). Although verification data from 2010 might seem outdated in the meantime, the practical choice for this event had to be based on the co-occurrence of all boundary conditions (including discharge, tidal water levels and precipitation) with credible reports of urban inundations at a considerable number of locations. In combination with the goal of using open-access data alone, suitable events were limited to a handful of options. While three of these data sets (from 2010 and 2013) served as calibration data, the remaining event with the most data points (2010) was used for validation. In this context, the comparison of simulated and observed flood depths yielded a root mean square error of RMSE = 0.03 m and a correlation coefficient of R2 = 0.75, respectively, making the modeling approach trustworthy enough for all intended tasks, i.e., the direct comparison of flood intensities under different adaptation scenarios. For a more detailed explanation regarding the processing of input data as well as the calibration and validation of the employed model, please refer to the independent publication by Scheiber et al. (2023), which discusses the general validity of open-access data in numerical analyses more profoundly.
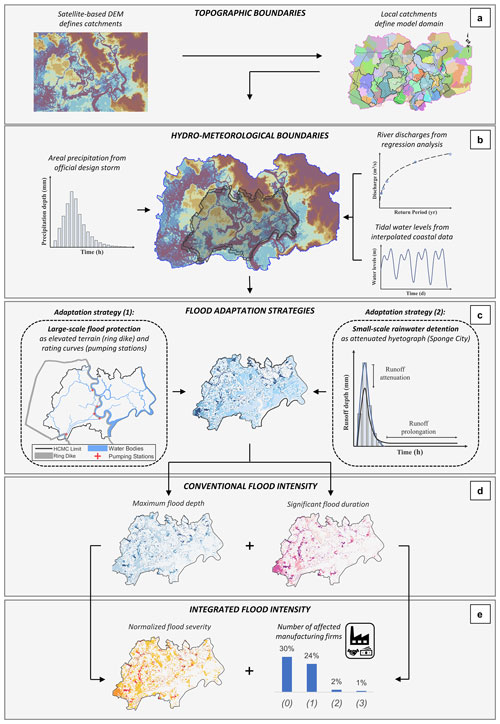
Figure 2Model setup. A bias-corrected digital elevation model was derived from the combination of SRTM and CoastalDEM; the local catchments that result from the local topography define the modeling domain (a, topographic boundaries). While tidal water levels were extrapolated from coastal data, average river discharges were taken from the literature and precipitation was forced on the model boundary in accordance with the valid design storm focusing on a return period of 1 year (b, hydro-meteorological boundaries; all elevation data displayed using scientific color maps; Crameri, 2021). Two adaptation strategies were investigated by either (1) implementing changed elevations due to a large-scale ring dike in combination with six parameterized pumping stations or (2) reproducing the hydraulic effects of decentralized water detention according to a Sponge City approach in the form of an attenuated hyetograph, respectively (c, flood adaptation strategies). The flood simulations resulted in spatiotemporal data on water surface elevations revealing local maximum flood depths and durations of significant flooding (d, conventional flood intensity). Finally, the local flood severity was estimated via the normalized flood severity index (INFS) and in terms of manufacturing firms that were still affected by significant flooding after implementation of the individual adaptation strategies (e, integrated flood intensity; all numerical results displayed in monochromatic colors for illustration purposes).
The described surface runoff model was employed to assess four different adaptation scenarios:
- (0)
a base case scenario without any technical adaptations to mitigate urban flooding;
- (1)
a large-scale protection project including a ring dike, multiple sluice gates and pumping stations protecting the central districts of HCMC from storm surges (and, in parts, pluvial flooding);
- (2)
a small-scale rainwater detention scheme as implemented in the Sponge City Program, mitigating the peak of rain-induced surface runoff; and
- (3)
a combined application of strategies (1) and (2).
Apart from a reference simulation without any adaption options (0), the first strategy (1) originates from the large-scale development project envisioned by VCAPS that was initiated by the Vietnamese Ministry of Agriculture and Rural Development (MARD) in 2016. In the framework of the numerical model, the central ring dike was implemented by locally fitting digital elevations to a designated height of 2.3 m above sea level (see Figs. 2c, left, and 3c), thus, directly affecting the simulated surface runoff in this area. The pumping stations, on the other hand, were realized by a customary HEC-RAS feature (since version 6.0), which allows for cell-specific discharges according to a user-defined rating curve. The six pumping stations were placed vis-à-vis the flood gates, i.e., at all crossings between the ring dike and a major inner-city canal (see Figs. 2c, left, and 3c). In contrast, the six large-scale sluice gates (and multiple smaller ones) that are also part of the underlying “MARD plan variant” (Phi et al., 2015) were not implemented in this study as these facilities would be locked and therefore without hydraulic effect in the considered flood events. While the structural solutions of the first adaptation strategy were integrated directly into the hydraulic model, the decentralized approach of the second strategy (2) had to be implemented in a parameterized manner. All rainwater detention measures – be it public basins, green roofs or private rain barrels – generally procure a similar alteration of surface runoff: while the effective volume stays the same, the local maxima in the hydrograph are attenuated and converted into (throttled) discharge of manageable magnitude and time. According to this volumetric analysis, the comprehensive application of small-scale rainwater detention as a realization of the Sponge City concept was represented by directly changing the hyetograph of the regular design storm, thereby translating the hydraulic effect of decentralized water storage into attenuated and prolonged rainfall (see Fig. 2c, right). In this connection, an approximate assessment based on Sentinel-2 images revealed that about one-third of the included area is covered by roofs. Optimistically estimating the potential of household level rain detention, it was assumed that a maximum of one-half of this roof-area could be utilized for water storage (i.e., connected to rainwater detention measures), leaving about 15 % of the city's total area to be considered in the parametrization scheme. The remaining scenario (3) finally combines the large-scale ring dike project with small-scale rainwater detention in order to assess the complementarity of these seemingly adverse strategies. All simulations yield spatiotemporal output data describing the water surface elevation at every cell of the modeling domain over time. To understand the resulting risks, flood intensity was further assessed through four proxies in two steps: the flood height (Fig. 2d, left), which quite intuitively represents time-independent maximum inundation depths at every point, and the flood duration (Fig. 2d, right) defined by the time span a certain point experiences a significant inundation, i.e., an inundation depth greater than 10 cm. These two parameters are the most common proxies for flood intensity and can directly be derived from the model outputs. Secondly, a more integrated view on the potential reduction in flood risk is provided by the normalized flood severity index (INFS) (Fig. 2e, left) and the number of affected manufacturing firms (Fig. 2e, right). The INFS combines flood depth and duration into one dimensionless parameter. For a given pair of coordinates, the INFS is calculated as the product of these two conventional flood intensity proxies divided by the product of the 95th percentiles of the same proxies as follows:
where refers to the maximum simulated flood depth over time at coordinates x and y and refers to the inundation duration over the predefined threshold of 10 cm at the same coordinates. Using the 95th spatial percentiles in the denominator eliminates the distorting effects of potential numerical artifacts. As a qualitative first estimate, this easy-to-apply index emphasizes those areas which are exposed to significant flooding over a significant time and, thus, are expected to experience the most severe damage across a given study area. A detailed explanation of the rationale behind the INFS and its validation can be found in an independent publication by Scheiber et al. (2023). The location of manufacturing firms in HCMC is based on information from the Vietnamese 2017 Economic Census collected by the General Statistics Office of Vietnam. These data were previously utilized in a study which investigated the constraints that affect firm decisions in undertaking flood adaptation measures based on SRTM elevation data (Leitold et al., 2021). In contrast to traditional cost–benefit analyses that require sophisticated damage–loss models, the avail of assessing only the number of affected firms is that all companies are valued equally, thereby avoiding an underestimation of (non-monetary) SME values (Kind et al., 2020; Hino and Nance, 2021). On this basis, the hydraulic effectiveness of each adaptation strategy was expressed through difference maps highlighting the local reduction in each of the described proxies in comparison with the base case, allowing for a qualitative estimation of the flood reduction that each of the hypothetical strategies offers and, what is more, facilitating a direct juxtaposition of their hydraulic effectiveness.
Allowing for the highly dynamic behavior of surface runoff volumes across the HCMC model, all spatiotemporal output data were converted into heat maps for the first three flood intensity proxies. The potential flood reduction in a given adaptation strategy was then illustrated by subtracting the respective base case heat map. The benefits of this methodology become clearer when studying the distribution of flood severity (INFS) reductions as depicted in Fig. 3. Analogous maps of the potential changes in maximum flood depth and duration can be found in the Supplement. Figure 3a and b, corresponding to strategies (1) and (2), visualize the reduction in flood severity due to the implementation of the VCAPS ring dike (including sluice gates and pumping stations) and the comprehensive application of water detention, respectively. When comparing the two strategies, areas with reduced flood severity (highlighted in green) significantly exceed after implementation of the first adaptation strategy (Fig. 3a), although they form certain clusters along the low-lying areas close to the major canals. This seems obvious given that the dike eradicates any tidal influence and the heavy-duty pumps are capable of emptying these crucial elements of urban drainage within hours. Then again, there are several areas (highlighted in blue) that experience increased flood severity, especially across the western, riverine parts of the municipal city of Thu Duc (hatched area), which lies east of the northernmost pumping station. Given their proximity to the Saigon River, these “new” flooding hotspots are very likely a consequence of excess water being pumped into the river from inside the ring dike. Flood reduction for the second adaptation strategy (Fig. 3b), on the other hand, is less pronounced but more uniformly distributed, likewise addressing low- and high-lying areas. In addition, this strategy hardly affects flood severity except for the exact spots that are alleviated by the ring dike strategy, which already hints at the benefits of using both strategies in combination. Changes in flood severity that can be expected for a combination of both strategies are depicted in the lower panel (Fig. 3c). At first sight, most flood severity reductions visible in Fig. 3a can also be found for the combined approach in Fig. 3c. When looking at the increases in flood severity, however, several of the inundations, which are induced by the implementation of a ring dike, diminish in the case of additional rainwater detention. This applies particularly to the areas east of the Saigon River, suggesting that certain detrimental effects of excessive pumping could be mitigated by a combined adaptation strategy. Complementing Fig. 3, the Supplement includes a more detailed frequency analysis further quantifying the changes in specific value ranges.
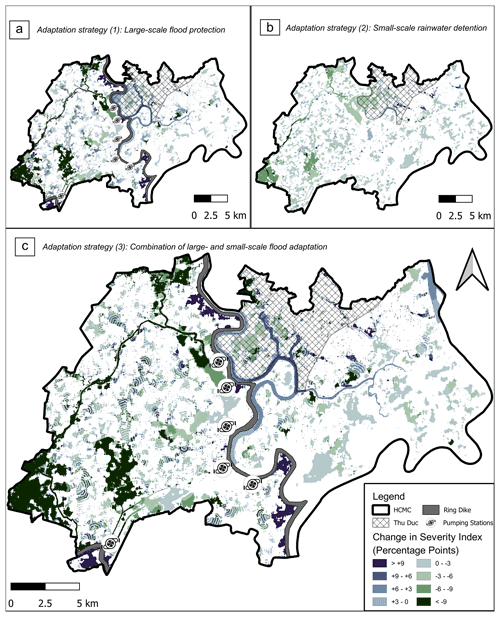
Figure 3Simulation results. Spatial changes in flood intensity in terms of decreases (green) and increases (blue) in normalized flood severity (INFS). (a) While the large-scale ring dike strategy improves the flooding situation in the low-lying areas along the major canals but worsens the situation for the western parts of the municipal city of Thu Duc (hatched area), (b) the decentralized water detention scheme shows more uniformly distributed improvements. (c) The combination of both approaches yields even better results as increases and decreases in flood severity overlap for the former two strategies in a highly complementary way. All INFS reductions displayed using scientific color maps (Crameri, 2021).
Adding to these findings, the following quantitative results also underline that both grey and green adaptation strategies are, in general, capable of alleviating the average intensity of local flooding in HCMC. This can be concluded, for instance, from average values of maximum flood depths and flood durations inside the ring dike, which are significantly reduced in relation to the reference case without adaptations (Table 1). In direct comparison, it becomes evident that (1) the currently constructed ring dike (including flood gates and pumping stations) would reduce average flood depths nearly as effectively as (2) the implementation of widespread water detention, whereas the potential improvements from (3) a combined approach suggest reductions in flood depth are even higher than the sum of the single components of strategies (1) and (2). The numerical simulations suggest not only that the considered strategies can be implemented without limiting their individual benefits but also that they are highly complementary with respect to their hydraulic effects. This is corroborated by the integrated flood intensity proxies (Table 2). For example, the INFS is reduced by −17.9 % for strategy 1 and −17.7 % for strategy 2, respectively, in comparison to the base case without adaptation. The combination of both, however, would yield a −38.3 % reduction in normalized flood severity (INFS). Further evidence for this complementarity can be found when assessing how the different adaptation strategies influence the number of manufacturing firms affected by significant flooding (with a depth of more than 10 cm). While the classic strategy (1) protects 33.8 % of the listed companies, decentralized detention measures alone (2) could also reduce the current number by −18.0 % after all. But again, the combined approach (3) would protect more manufacturing firms than the individual measures, yielding a 58 % higher effectiveness compared to the ring dike scheme alone. Even though these numerical results already indicate that small-scale measures can support future flood adaptation concepts in an effective and straightforward manner, they do not (yet) allow for the strategic low-regret qualities of the assessed options and the implications that arise for local decision-makers.
Table 1Potential reduction in flood intensity for three adaptation strategies: (1) large-scale ring dike scheme including pumping stations, (2) comprehensive application of decentralized rainwater detention and (3) the combination of both approaches. Improvements are expressed through decreasing mean values (inside the ring dike) of the conventional flood intensity proxies, maximum flood depths in centimeters (left) and significant flood duration with depths higher than 10 cm in hours (right), in comparison with respective values for (0) the base case without adaptation measures.
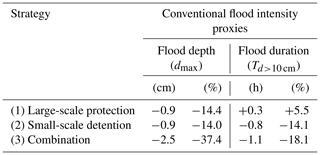
Table 2Potential reduction in flood intensity for three adaptation strategies: (1) large-scale ring dike scheme including pumping stations, (2) comprehensive application of decentralized rainwater detention and (3) the combination of both approaches. Improvements are expressed through decreasing values (inside the ring dike) of two integrated flood intensity proxies, namely the average normalized flood severity index (INFS) (left) and the total number of manufacturing firms that are affected by a flood depth greater than 10 cm (right), in comparison with respective values for (0) the base case without adaptation measures.
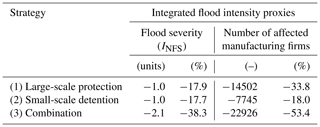
Several studies have recently assessed the available flood adaptation options of HCMC, with a special focus on resistance against extreme storm surges and such hazards under future sea level rise scenarios (VCAPS, 2013; Lasage et al., 2014; Scussolini et al., 2017). However, no attention was paid to the more frequent, especially pluvial, events. But these incidents demonstrably cause financial losses that are up to 10 times higher than singular extremes (ADB, 2010) and affect small and medium-sized manufacturing firms, which are the backbone of the national economy, to a disproportionately high degree (Leitold and Revilla Diez, 2019). To address this knowledge gap, a numerical model was employed to evaluate possible responses to urban inundations resulting from heavy precipitation of an annual return period. Two exemplary adaptation measures, (1) a classic (grey) protection scheme in the form of a large-scale ring dike and (2) a (green) Sponge City approach in terms of decentralized rainwater detention, were implemented in a process-based hydrological model.
The fact that this model was exclusively built on freely accessible boundary conditions introduces some uncertainties to the numerical setup: first of all, the elevation data from SRTM and CoastalDEM typically come with a site-specific vertical bias (Schumann and Bates, 2018; Kulp and Strauss, 2019; Vernimmen et al., 2020; Mukul et al., 2015). However, a comparison with three major lidar samples suggests that, given the specific case of HCMC, errors on the order of 0.5 m are to be expected (A = 165 km2, ME = −0.45 m, SD = 0.81 m, RMSE = 0.93 m; Scheiber et al., 2023). Yet, although the results of the here-employed model are highly dependent on the topographic conditions across the city as well, the influence of a vertical bias diminishes when simulation runs with the same systematic error are directly compared. The same applies to the (conservative) assumption that the local drainage system malfunctions from the beginning of each model run. Furthermore, the impact of local land subsidence was not considered in the assessed simulations of present-day adaptation responses, but the extrapolation of such trends should be a requisite for simulations that address options under future climate change projections given their significant share in relative sea level rise (Nicholls et al., 2021). Additional limitations arise from the definition of a design storm and from the parametrization of rainwater detention in the form of an attenuated hyetograph (cf. Sect. 2, “Material and methods”). The precipitation boundary was determined in conjunction with a systematic mapping of local policies and adaptation guidelines. It follows a 180 min design storm specified by an official decree enforced in decision 752/QD-TTg of the HCMC government. This duration may be seen as a balance point: while the large-scale ring dike and pumping stations are constructed to cope with extraordinary precipitation volumes (mostly in combination with spring tides), the suggested detention measures aim at complementing the local drainage system during shorter cloudburst events. The same applies to the return period, which certainly has a considerable impact on the effectiveness and technical limitation of the compared strategies. Nevertheless, the commitment to this specific design storm has to be seen in a row of conceptual assumptions that were necessary to undertake a direct comparison of these hydraulically unlike adaptation options. Furthermore, even if a comparison of different technical solutions for this type of decentralized rainwater storage is generally possible on an analytical basis – investigating what detention measure would theoretically cause which attenuation – their parameterized implementation in the model would nearly be identical, as is their general working principle. As a consequence, the experienced flooding would also be very similar in its pattern of depth and duration for all realizations of the Sponge City concept. Even if presumable heterogeneities in the realization of rainwater detention measures are neglected by this uniform parametrization, it still allows for the fact that subsidized micro-scale solutions may be implemented in a people-centered, i.e., bottom-up, approach. In the first place, this new paradigm in adaptation can complicate official monitoring but may render supervision by local authorities unnecessary in the end. The estimation of the roofed area from satellite imagery and corresponding detention capacities entails additional uncertainties with respect to the assumed runoff attenuation. In reality, the actual shape of this hyetograph depends on a multitude of factors including technical details about the individual solutions (how much storage volume per unit) as well as the degree of implementation (how many units per area). Nevertheless, the presented approach is sufficiently descriptive for a conceptual juxtaposition of the effects and performance of the two mitigation strategies under consideration and demonstrates the general working principles despite the underlying simplifications: the large-scale pumping stations comprised in the classic protection scheme reduce flood volumes along the inner-city canals and thus represent a line or even point sink within the numerical model; the implementation of the Sponge City concept, in contrast, is characterized by spatially uniform runoff attenuation, which translates to an area sink for flood volumes across the whole model domain. The aim of this study, i.e., to compare the working principles behind these two seemingly adverse adaptation options, is thoroughly accomplished by the employed conceptual approach. Although the employed surface runoff model is based on these assumptions, its implications still go beyond earlier DEM-based analyses (Dang and Kumar, 2017; Leitold and Revilla Diez, 2019) and its limitations are comparable to other process-based models of this region (Scussolini et al., 2017). Even though simulated urban inundations should not be mistaken for quantitative forecasts (as needed by, for instance, insurance companies), the reduction in flood intensity and especially the spatiotemporal differences between the assessed strategies can help both researchers and decision-makers to critically compare the hydraulic effects of available adaptation options. In this context, the combination of conventional and integrated flood intensity proxies allows for a holistic view of their potential in physical flood risk reduction and avoids a singular focus on economic assets, which would underestimate the social relevance of small and medium-sized enterprises (Kind et al., 2020; Hino and Nance, 2021).
The obtained simulation results suggest that both adaptation measures are generally capable of alleviating the flooding situation in HCMC, albeit on very different spatial and temporal scales. The classic protection scheme has a slightly better reduction rate than the projected rainwater storage regarding flood depth but increases the average flood duration inside the ring dike. Positive effects of the ring dike are also limited to the low-lying areas in the vicinity of the inner-city canals, which are easily drained in contrast to the more remote and high-lying districts. In addition, the grey adaptation measure relies on sufficient capacities and smooth operation of a few central pumping stations, which makes this concept highly vulnerable in the case of technical or operational failure. The importance of technical robustness and reliability becomes particularly clear when considering the role of the existing drainage system. Outdated and in many regards deficient, this system is one of the main drivers of growing flood risk in HCMC and the epitome of critical dependence on grey infrastructure and susceptible technical solutions. A Sponge City approach, in contrast, is characterized by its modularity comprising a large number of decentralized elements. This is a major advantage as it disperses the risk of failure through redundancy and therefore increases the resilience of the overall system. However, in urban settings, the implementation of medium-scale solutions for rainwater storage, like dedicated detention basins, may entail conflicts with other (mostly commercial) interests in land use management due to the required consumption of limited public space. Multiple administrative authorities, private land owners and developers typically need to be involved to make decisions. Although arising conflicts can be addressed through active participation by representatives of the local citizens and businesses as well as experts from the administration in a joint co-design process (Chen et al., 2021), their complexity can prolong and in some cases even prevent implementation plans. Hence, the most promising approach for integrating the Sponge City concept into a highly urbanized area, like HCMC, is promoting small- and even micro-scale solutions on a neighborhood and household or firm level. These are inherently easier to realize than medium-scale solutions as they can make use of existing residential spaces or vacant lots (not being subject to commercial interests) and can be governed through incentives more easily. Also, concerning future trends, both strategies differ in their degree of sustainability: because the operation of grey flood protection measures, and specifically the ring dike, is defined by absolute design heights, these structures are constructed for one specific water level projection and constant land subsidence rate. In contrast, these two components of relative sea level rise hardly impair the potential of rainwater detention. Although classic flood protection shows higher effectiveness in almost all assessed flood intensity proxies, this study of HCMC shows that small-scale solutions, as projected in the framework of a Sponge City approach, excel in terms of technical resilience and that they are readily available options for addressing residual flood risk. Especially the combination of both strategies offers much-needed technical redundancy besides the obvious gains in quantitative flood reduction, which, in the present case, are even higher than if the solutions would be implemented individually.
With regard to the low-regret paradigm, flexible approaches and strategic planning for the future are central elements of a successful disaster risk response strategy (IPCC, 2012; Marchau et al., 2019). Typically, an adaptation plan contains multiple adaptation pathways, visualizing the impact and dependence of single adaptation measures on future capacities to deal with climate change effects (Haasnoot et al., 2013; Kwakkel et al., 2015; Haasnoot et al., 2019). Planning adaptation with a low-regret mindset facilitates small-scale measures along the way, with the chance of avoiding more drastic interference within the natural and social setting and circumventing maladaptation or maldevelopment (David et al., 2021). With this in mind, the ring dike in HCMC is a direct protection measure for the low-lying parts of the inner city. Complemented by heavy-duty pumps, it shields the central districts from harmful storm surges and eradicates the tidal influence on the inner-city canals. However, even if parts of the precipitation volumes remain in more highly elevated areas, unable to drain through the deficient drainage system, this surplus of discharge still raises the water levels of the gaining Saigon River. Like the general dependence on a single technical structure, this fact shows a maladaptive tendency as it can be detrimental to the outer reaches of HCMC (outside the ring dike). These are increasingly inhabited by the poorer, more vulnerable portions of the population (Duy et al., 2018), who now experience even higher floods. To alleviate this tendency and prevent maldevelopment, the currently constructed solution can be complemented with incentives for societal and communal flood protection: decentralized rainwater detention verifiably attenuates the runoff peak and allots discharges to a longer period (Jiang et al., 2022). Considering an adaptation pathway plan for HCMC from a governance perspective, the ring dike is a unique project based on a binary decision (to build or not to build). It is designed to protect a given domain from a clearly specified set of natural hazards. Rainwater detention, on the other hand, is a modular category of individual measures following the Sponge City concept which does not need further specification and can be tailored to the specific demands of the local situation. This makes this approach highly flexible as its spatiotemporal implementation can be controlled by targeted subsidies, e.g., for purchasing and installing private rain barrels. Such micro-scale solutions enable both individuals and SMEs, who currently depend on top-down decisions, to actively participate in (bottom-up) climate change adaptation on a community and household level. Finally, this approach tackles flooding at its source and does not interfere with other protection measures on site. These aspects – flexibility, the capacity of local stakeholders to respond gradually and in accordance with near-future precipitation projections, and the ability to mitigate without impairing protection efforts on site – make the Sponge City concept a prime addition to a successful low-regret adaptation strategy.
As mentioned before, the construction of a ring dike, multiple flood gates and pumping stations is currently underway in HCMC. This adaptation pathway is an effective response to the current flooding situation as it protects the central districts of the city from high tides and coastal storm surges. However, frequent rain events cause major socio-economic disruptions and induce significant financial losses to the local economy as well. To address those areas that are not yet protected from pluvial flooding or even adversely affected by the installation of the ring dike, pursuing a Sponge City approach would be a second and highly complementary adaptation pathway. In this context, decentralized rainwater detention in any form – be it green roofs, rain barrels or detention basins – can be considered useful, especially in view of the impending rise in relative sea levels through global warming and local land subsidence. This may be even more beneficial as many of these solutions can be implemented as multi-purpose structures or possess valuable ecosystem services, first and foremost beneficial to mitigating urban-heat-island effects (He et al., 2019). Although further adding to the low-regret character of this concept, an assessment of the co-benefits of individual Sponge City measures would go beyond the scope of this study. In the end, it is highly desirable to consider this vital concept when technically approaching the increasingly severe disaster risk of low-elevation coastal zones in general and of Southeast Asian metropolises in particular to prepare for a deeply uncertain future.
No code was used in this research. Details about the general processing of numerical data are provided in the methods section or can be inquired from the corresponding author.
The presented model setup is completely based on open-access data from third parties, which are clearly referenced in the companion paper and specified in the respective list of references. Modeling outputs will be made available in a decision support tool in the context of the research project DECIDER (https://www.decider-project.org, last access: 21 June 2023). All other data can be requested from the corresponding author.
The supplement related to this article is available online at: https://doi.org/10.5194/nhess-23-2333-2023-supplement.
LS, MHJ and JV designed the hydro-numerical model finally set up and operated by MHJ. JRD acquired the census data, from which RL extracted the geolocation of manufacturing firms. MHJ processed the simulation results, which LS, MHJ and JV interpreted. LS and CGD conceptualized the paper outline. LS and CGD wrote the initial manuscript with input from MHJ, while JV, JRD, HQN and TS reviewed and edited the final text. JV and TS (co-)designed the overarching research project and were responsible for funding resources at the Ludwig Franzius Institute for Hydraulics, Estuarine and Coastal Engineering, as was JRD at the Global South Studies Center. All three provided guidance throughout the entire study.
The contact author has declared that none of the authors has any competing interests.
Publisher's note: Copernicus Publications remains neutral with regard to jurisdictional claims in published maps and institutional affiliations.
This article is part of the special issue “Future risk and adaptation in coastal cities”. It is not associated with a conference.
The list of inundation hotspots, forming the basis for the model validation in this study, was generously provided by Nguyen Quy from the HCMC-based engineering company EPT Ltd. Moreover, the authors wish to express their gratitude to Friedrich Hilgenstock from WTM Engineers GmbH for his expert guidance in technical flood adaptation options. Talia Schoonees provided helpful comments on the writing style, which is highly appreciated. Finally, sincere thanks go to the editor at Natural Hazards and Earth System Sciences for handling the paper and three anonymous reviewers for their helpful comments.
This research has received funding from the DECIDER project sponsored by the German Federal Ministry of Education and Research (BMBF; grant no. 01LZ1703H). C. Gabriel David is funded by the Deutsche
Forschungsgemeinschaft (DFG, German Research Foundation) under Germany's
Excellence Strategy (grant no. UP 8/1).
The publication of this article was funded by the open-access fund of Leibniz Universität Hannover.
This paper was edited by Liang Emlyn Yang and reviewed by three anonymous referees.
Abidin, H. Z., Andreas, H., Gumilar, I., and Wibowo, I. R. R.: On correlation between urban development, land subsidence and flooding phenomena in Jakarta, Proc. IAHS, 370, 15–20, https://doi.org/10.5194/piahs-370-15-2015, 2015.
ADB: Ho Chi Minh City – Adaptation to Climate Change: Summary Report, Asian Development Bank, Manila, the Philippines, ISBN 978-971-561-893-9, 2010.
Barragán, J. M. and de Andrés, M.: Analysis and trends of the world's coastal cities and agglomerations, Ocean Coast. Manage., 114, 11–20, https://doi.org/10.1016/j.ocecoaman.2015.06.004, 2015.
Caldwell, P. C., Merrifield, M. A., and Thompson, P. R.: Sea level measured by tide gauges from global oceans – the Joint Archive for Sea Level holdings (NCEI Accession 0019568), Version 5.5, NOAA National Centers for Environmental Information (NOAA), https://doi.org/10.7289/v5v40s7w, 2015.
Chen, S., van de Ven, F. H. M., Zevenbergen, C., Verbeeck, S., Ye, Q., Zhang, W., and Wei, L.: Revisiting China's Sponge City Planning Approach: Lessons From a Case Study on Qinhuai District, Nanjing, Front. Environ. Sci., 9, 748231, https://doi.org/10.3389/fenvs.2021.748231, 2021.
Crameri, F.: Scientific colour maps (7.0.1), Zenodo [data set], https://doi.org/10.5281/zenodo.5501399, 2021.
Dang, A. T. N. and Kumar, L.: Application of remote sensing and GIS-based hydrological modelling for flood risk analysis: a case study of District 8, Ho Chi Minh city, Vietnam, Geomatics, Natural Hazards and Risk, 8, 1792–1811, https://doi.org/10.1080/19475705.2017.1388853, 2017.
David, C. G., Hennig, A., Ratter, B. M. W., Roeber, V., Zahid, and Schlurmann, T.: Considering socio-political framings when analyzing coastal climate change effects can prevent maldevelopment on small islands, Nat. Commun., 12, 5882, https://doi.org/10.1038/s41467-021-26082-5, 2021.
Dong, X., Guo, H., and Zeng, S.: Enhancing future resilience in urban drainage system: Green versus grey infrastructure, Water Res., 124, 280–289, https://doi.org/10.1016/j.watres.2017.07.038, 2017.
Doocy, S., Daniels, A., Murray, S., and Kirsch, T. D.: The human impact of floods: a historical review of events 1980–2009 and systematic literature review, PLoS Curr., 5, 23857425, https://www.ncbi.nlm.nih.gov/pmc/articles/PMC3644291/ (last access: 21 June 2023), 2013.
Downes, N. K. and Storch, H.: Current Constraints and Future Directions for Risk Adapted Land-Use Planning Practices in the High-Density Asian Setting of Ho Chi Minh City, Planning Practice & Research, 29, 220–237, https://doi.org/10.1080/02697459.2014.929835, 2014.
Duffy, C. E., Braun, A., and Hochschild, V.: Surface Subsidence in Urbanized Coastal Areas: PSI Methods Based on Sentinel-1 for Ho Chi Minh City, Remote Sens., 12, 4130, https://doi.org/10.3390/rs12244130, 2020.
Duvat, V. K. E. and Magnan, A. K.: Rapid human-driven undermining of atoll island capacity to adjust to ocean climate-related pressures, Sci. Rep., 9, 15129, https://doi.org/10.1038/s41598-019-51468-3, 2019.
Duy, P. N., Chapman, L., Tight, M., Linh, P. N., and Le Thuong, V.: Increasing vulnerability to floods in new development areas: evidence from Ho Chi Minh City, Int. J. Clim. Chang. Str., 10, 197–212, https://doi.org/10.1108/IJCCSM-12-2016-0169, 2018.
Erkens, G., Bucx, T., Dam, R., de Lange, G., and Lambert, J.: Sinking coastal cities, Proc. IAHS, 372, 189–198, https://doi.org/10.5194/piahs-372-189-2015, 2015.
Farr, T. G., Rosen, P. A., Caro, E., Crippen, R., Duren, R., Hensley, S., Kobrick, M., Paller, M., Rodriguez, E., Roth, L., Seal, D., Shaffer, S., Shimada, J., Umland, J., Werner, M., Oskin, M., Burbank, D., and Alsdorf, D.: The shuttle radar topography mission, Rev. Geophys., 45, RG2004, https://doi.org/10.1029/2005RG000183, 2007.
Gugliotta, M., Saito, Y., Ta, T. K. O., and van Nguyen, L.: Valley-Confinement and River-Tidal Controls on Channel Morphology Along the Fluvial to Marine Transition Zone of the Ðong Nai River System, Vietnam, Front. Earth Sci., 7, 202, https://doi.org/10.3389/feart.2019.00202, 2019.
Haasnoot, M., Kwakkel, J. H., Walker, W. E., and ter Maat, J.: Dynamic adaptive policy pathways: A method for crafting robust decisions for a deeply uncertain world, Glob. Environ. Change, 23, 485–498, https://doi.org/10.1016/j.gloenvcha.2012.12.006, 2013.
Haasnoot, M., Brown, S., Scussolini, P., Jimenez, J. A., Vafeidis, A. T., and Nicholls, R. J.: Generic adaptation pathways for coastal archetypes under uncertain sea-level rise, Environ. Res. Commun., 1, 71006, https://doi.org/10.1088/2515-7620/ab1871, 2019.
Hallegatte, S., Green, C., Nicholls, R. J., and Corfee-Morlot, J.: Future flood losses in major coastal cities, Nat. Clim. Chang., 3, 802–806, https://doi.org/10.1038/NCLIMATE1979, 2013.
Hamel, P. and Tan, L.: Blue-Green Infrastructure for Flood and Water Quality Management in Southeast Asia: Evidence and Knowledge Gaps, Environ. Manage., 69, 699–718, https://doi.org/10.1007/s00267-021-01467-w, 2022.
Hanson, S., Nicholls, R., Ranger, N., Hallegatte, S., Corfee-Morlot, J., Herweijer, C., and Chateau, J.: A global ranking of port cities with high exposure to climate extremes, Climatic Change, 104, 89–111, https://doi.org/10.1007/s10584-010-9977-4, 2011.
Hawken, S., Sepasgozar, S., Prodanovic, V., Jing, J., Bakelmun, A., Avazpour, B., Che, S., and Zhang, K.: What makes a successful Sponge City project? Expert perceptions of critical factors in integrated urban water management in the Asia-Pacific, Sustain. Cities Soc., 75, 103317, https://doi.org/10.1016/j.scs.2021.103317, 2021.
He, B.-J., Zhu, J., Zhao, D.-X., Gou, Z.-H., Qi, J.-D., and Wang, J.: Co-benefits approach: Opportunities for implementing sponge city and urban heat island mitigation, Land Use Policy, 86, 147–157, https://doi.org/10.1016/j.landusepol.2019.05.003, 2019.
Hino, M. and Nance, E.: Five ways to ensure flood-risk research helps the most vulnerable, Nature, 595, 27–29, https://doi.org/10.1038/d41586-021-01750-0, 2021.
Huong, H. T. L. and Pathirana, A.: Urbanization and climate change impacts on future urban flooding in Can Tho city, Vietnam, Hydrol. Earth Syst. Sci., 17, 379–394, https://doi.org/10.5194/hess-17-379-2013, 2013.
IPCC: Managing the Risks of Extreme Events and Disasters to Advance Climate Change Adaptation. A Special Report of Working Groups I and II of the Intergovernmental Panel on Climate Change, edited by: Field, C. B., Barros, V., Stocker, T. F., Qin, D., Dokken, D. J., Ebi, K. L., Mastrandrea, M. D., Mach, K. J., Plattner, G.-K., Allen, S. K., Tignor, M., and Midgley, P. M., Cambridge University Press, Cambridge, UK, and New York, NY, USA, 582 pp., ISBN 978-1-107-02506-6, 2012.
IPCC: Climate Change 2014: Impacts, Adaptation, and Vulnerability. Part A: Global and Sectoral Aspects. Contribution of Working Group II to the Fifth Assessment Report of the Intergovernmental Panel on Climate Change, edited by: Field, C. B., Barros, V. R., Dokken, D. J., Mach, K. J., Mastrandrea, M. D., Bilir, T. E., Chatterjee, M., Ebi, K. L., Estrada, Y. O., Genova, R. C., Girma, B., Kissel, E. S., Levy, A. N., MacCracken, S., Mastrandrea, P. R., and White, L. L., Cambridge University Press, Cambridge, United Kingdom and New York, NY, USA, 1132 pp., ISBN 978-1-107-05807-1, 2014.
IPCC: IPCC Special Report on the Ocean and Cryosphere in a Changing Climate, edited by: Pörtner, H.-O., Roberts, D. C., Masson-Delmotte, V., Zhai, P., Tignor, M., Poloczanska, E., Mintenbeck, K., Alegría, A., Nicolai, M., Okem, A., Petzold, J., Rama, B., and Weyer, N. M., Cambridge University Press, Cambridge, UK and New York, NY, USA, 755 pp., https://doi.org/10.1017/9781009157964, 2019.
IPCC: Climate Change 2021: The Physical Science Basis. Contribution of Working Group I to the Sixth Assessment Report of the Intergovernmental Panel on Climate Change, edited by: Masson-Delmotte, V., Zhai, P., Pirani, A., Connors, S. L., Péan, C., Berger, S., Caud, N., Chen, Y., Goldfarb, L., Gomis, M. I., Huang, M., Leitzell, K., Lonnoy, E., Matthews, J. B. R., Maycock, T. K., Waterfield, T., Yelekçi, O., Yu, R., and Zhou, B., Cambridge University Press, Cambridge, United Kingdom and New York, NY, USA, 2391 pp. https://www.ipcc.ch/report/ar6/wg1/ (last access: 21 June 2023), 2021.
Jia, H., Wang, Z., Zhen, X., Clar, M., and Yu, S. L.: China's sponge city construction: A discussion on technical approaches, Front. Environ. Sci. Eng., 11, 18, https://doi.org/10.1007/s11783-017-0984-9, 2017.
Jia, H., Hu, J., Huang, T., Chen, A. S., and Ma, Y.: Urban Runoff Control and Sponge City Construction, Water, 14, 1910, https://doi.org/10.3390/w14121910, 2022.
Jiang, Y., Qiu, L., Gao, T., and Zhang, S.: Systematic Application of Sponge City Facilities at Community Scale Based on SWMM, Water, 14, 591, https://doi.org/10.3390/w14040591, 2022.
Kaneko, S. and Toyota, T.: Long-Term Urbanization and Land Subsidence in Asian Megacities: An Indicators System Approach, in: Groundwater and Subsurface Environments, edited by: Taniguchi, M., Springer, Tokyo, 249–270, https://doi.org/10.1007/978-4-431-53904-9_13, 2011.
Kind, J., Botzen, W. J. W., and Aerts, J. C. J. H.: Social vulnerability in cost-benefit analysis for flood risk management, Envir. Dev. Econ., 25, 115–134, https://doi.org/10.1017/S1355770X19000275, 2020.
Köster, S.: How the Sponge City becomes a supplementary water supply infrastructure, Water-Energy Nexus, 4, 35–40, https://doi.org/10.1016/j.wen.2021.02.002, 2021.
Kulp, S. A. and Strauss, B. H.: CoastalDEM: A global coastal digital elevation model improved from SRTM using a neural network, Remote Sens. Environ., 206, 231–239, https://doi.org/10.1016/j.rse.2017.12.026, 2018.
Kulp, S. A. and Strauss, B. H.: New elevation data triple estimates of global vulnerability to sea-level rise and coastal flooding, Nat. Commun., 10, 4844, https://doi.org/10.1038/s41467-019-12808-z, 2019.
Kumar, N., Liu, X., Narayanasamydamodaran, S., and Pandey, K. K.: A Systematic Review Comparing Urban Flood Management Practices in India to China's Sponge City Program, Sustainability, 13, 6346, https://doi.org/10.3390/su13116346, 2021.
Kwakkel, J. H., Haasnoot, M., and Walker, W. E.: Developing dynamic adaptive policy pathways: a computer-assisted approach for developing adaptive strategies for a deeply uncertain world, Climatic Change, 132, 373–386, https://doi.org/10.1007/s10584-014-1210-4, 2015.
Lasage, R., Veldkamp, T. I. E., de Moel, H., Van, T. C., Phi, H. L., Vellinga, P., and Aerts, J. C. J. H.: Assessment of the effectiveness of flood adaptation strategies for HCMC, Nat. Hazards Earth Syst. Sci., 14, 1441–1457, https://doi.org/10.5194/nhess-14-1441-2014, 2014.
Leitold, R. and Revilla Diez, J.: Exposure of manufacturing firms to future sea level rise in Ho Chi Minh City, Vietnam, J. Maps, 15, 13–20, https://doi.org/10.1080/17445647.2018.1548385, 2019.
Leitold, R., Garschagen, M., van Tran, and Revilla Diez, J.: Flood risk reduction and climate change adaptation of manufacturing firms: Global knowledge gaps and lessons from Ho Chi Minh City, Int. J. Disast. Risk Re., 61, 102351, https://doi.org/10.1016/j.ijdrr.2021.102351, 2021.
Lempert, R. J.: Robust Decision Making (RDM), in: Decision Making under Deep Uncertainty, Springer International Publishing, Cham, 23–51, https://doi.org/10.1007/978-3-030-05252-2_2, 2019.
Li, F. and Zhang, J.: A review of the progress in Chinese Sponge City programme: challenges and opportunities for urban stormwater management, Water Supp., 22, 1638–1651, https://doi.org/10.2166/ws.2021.327, 2022.
Loc, H. H., Babel, M. S., Weesakul, S., Irvine, K. N., and Duyen, P. M.: Exploratory assessment of SUDS feasibility in Nhieu Loc-Thi Nghe Basin, Ho Chi Minh City, Vietnam, British Journal of Environment and Climate Change, 5, 91–103, https://doi.org/10.9734/BJECC/2015/11534, 2015.
Marchau, V. A. W. J., Walker, W. E., Bloemen, P. J. T. M., and Popper, S. W.: Decision Making under Deep Uncertainty – From Theory to Practice, Springer, Cham, Switzerland, https://doi.org/10.1007/978-3-030-05252-2, 2019.
McGranahan, G., Balk, D., and Anderson, B.: The rising tide: assessing the risks of climate change and human settlements in low elevation coastal zones, Environ. Urban., 19, 17–37, https://doi.org/10.1177/0956247807076960, 2007.
MONRE: Climate change and sea level rise scenarios for Viet Nam, Ministry of Natural Resources and Environment (MONRE), Technical Report, http://coastal-protection-mekongdelta.com/download/library/135.CC_SLR2016_EN.pdf (last access: 13 June 2023), 2016.
Morris, R. L., Konlechner, T. M., Ghisalberti, M., and Swearer, S. E.: From grey to green: Efficacy of eco-engineering solutions for nature-based coastal defence, Glob. Change Biol., 24, 1827–1842, https://doi.org/10.1111/gcb.14063, 2018.
Mukul, M., Srivastava, V., and Mukul, M.: Analysis of the accuracy of Shuttle Radar Topography Mission (SRTM) height models using International Global Navigation Satellite System Service (IGS) Network, J. Earth Syst. Sci., 124, 1343–1357, https://doi.org/10.1007/s12040-015-0597-2, 2015.
Muthusamy, M., Rivas Casado, M., Salmoral, G., Irvine, T., and Leinster, P.: A Remote Sensing Based Integrated Approach to Quantify the Impact of Fluvial and Pluvial Flooding in an Urban Catchment, Remote Sens., 11, 577, https://doi.org/10.3390/rs11050577, 2019.
Neise, T., Revilla Diez, J., and Garschagen, M.: Firms as drivers of integrative adaptive regional development in the context of environmental hazards in developing countries and emerging economies – A conceptual framework, Environ. Plann. C, 36, 1522–1541, https://doi.org/10.1177/2399654418771079, 2018.
Neumann, B., Vafeidis, A. T., Zimmermann, J., and Nicholls, R. J.: Future coastal population growth and exposure to sea-level rise and coastal flooding–a global assessment, PloS one, 10, e0118571, https://doi.org/10.1371/journal.pone.0118571, 2015.
Nguyen, T. T., Ngo, H. H., Guo, W., Wang, X. C., Ren, N., Li, G., Ding, J., and Liang, H.: Implementation of a specific urban water management – Sponge City, Sci. Total Environ., 652, 147–162, https://doi.org/10.1016/j.scitotenv.2018.10.168, 2019.
Nicholls, R. J., Lincke, D., Hinkel, J., Brown, S., Vafeidis, A. T., Meyssignac, B., Hanson, S. E., Merkens, J.-L., and Fang, J.: A global analysis of subsidence, relative sea-level change and coastal flood exposure, Nat. Clim. Chang., 11, 338–342, https://doi.org/10.1038/s41558-021-00993-z, 2021.
Patel, D. P., Ramirez, J. A., Srivastava, P. K., Bray, M., and Han, D.: Assessment of flood inundation mapping of Surat city by coupled 1D/2D hydrodynamic modeling: a case application of the new HEC-RAS 5, Nat. Hazards, 89, 93–130, https://doi.org/10.1007/s11069-017-2956-6, 2017.
Phi, H. L.: Climate change and urban flooding in Ho Chi Minh City, in: Proceedings of the 3rd International Conference on Climate and Water, 3–6 September 2007, Helsinki, Finland, Finnish Environment Institute, 194–199, ISBN 978-952-11-2790-8194-199, http://hdl.handle.net/10138/233271, 2007.
Phi, H. L., Hermans, L. M., Douven, W. J., van Halsema, G. E., and Khan, M. F.: A framework to assess plan implementation maturity with an application to flood management in Vietnam, Water Int., 40, 984–1003, https://doi.org/10.1080/02508060.2015.1101528, 2015.
Qi, Y., Chan, F. K. S., Thorne, C., O'Donnell, E., Quagliolo, C., Comino, E., Pezzoli, A., Li, L., Griffiths, J., Sang, Y., and Feng, M.: Addressing Challenges of Urban Water Management in Chinese Sponge Cities via Nature-Based Solutions, Water, 12, 2788, https://doi.org/10.3390/w12102788, 2020.
Rangari, V. A., Umamahesh, N. V., and Bhatt, C. M.: Assessment of inundation risk in urban floods using HEC RAS 2D, Model. Earth Syst. Environ., 5, 1839–1851, https://doi.org/10.1007/s40808-019-00641-8, 2019.
Rözer, V., Kreibich, H., Schröter, K., Müller, M., Sairam, N., Doss-Gollin, J., Lall, U., and Merz, B.: Probabilistic Models Significantly Reduce Uncertainty in Hurricane Harvey Pluvial Flood Loss Estimates, Earths Future, 7, 384–394, https://doi.org/10.1029/2018EF001074, 2019.
Scheiber, L., Hoballah Jalloul, M., Jordan, C., Visscher, J., Nguyen, H. Q., and Schlurmann, T.: The potential of open-access data for flood estimations: uncovering inundation hotspots in Ho Chi Minh City, Vietnam, through a normalized flood severity index, Nat. Hazards Earth Syst. Sci., 23, 2313–2332, https://doi.org/10.5194/nhess-23-2313-2023, 2023.
Schumann, G. J.-P. and Bates, P. D.: The Need for a High-Accuracy, Open-Access Global DEM, Front. Earth Sci., 6, 225, https://doi.org/10.3389/feart.2018.00225, 2018.
Scussolini, P., van Tran, T. T., Koks, E., Diaz-Loaiza, A., Ho, P. L., and Lasage, R.: Adaptation to Sea Level Rise: A Multidisciplinary Analysis for Ho Chi Minh City, Vietnam, Water Resour. Res., 53, 10841–10857, https://doi.org/10.1002/2017WR021344, 2017.
Sun, Y., Deng, L., Pan, S.-Y., Chiang, P.-C., Sable, S. S., and Shah, K. J.: Integration of green and gray infrastructures for sponge city: Water and energy nexus, Water-Energy Nexus, 3, 29–40, https://doi.org/10.1016/j.wen.2020.03.003, 2020.
Tran Ngoc, T. D., Perset, M., Strady, E., Phan, T. S. H., Vachaud, G., Quertamp, F., and Gratiot, N.: Ho Chi Minh City growing with water related challenges, in: Water, megacities and global change: portraits of 15 emblematic cities of the world, UNESCO/ARCEAU, Paris, France, 46–49, ISBN 978-92-3-100161-1, 2016.
USACE: HEC-RAS Version 6.0, Hydrologic Engineering Center's River Analysis System, US Army Corps of Engineers Hydrologic Engineering Center, Davis [code], https://www.hec.usace.army.mil/software/hec-ras/ (last access: 13 June 2023), 2021.
VCAPS: Climate Adaptation Strategy Ho Chi Minh City – moving towards the sea with climate change adaptation, Vietnam Climate Adaptation PartnerShip (VCAPS), 2013.
Vernimmen, R., Hooijer, A., and Pronk, M.: New ICESat-2 Satellite LiDAR Data Allow First Global Lowland DTM Suitable for Accurate Coastal Flood Risk Assessment, Remote Sens., 12, 2827, https://doi.org/10.3390/rs12172827, 2020.
Yalcin, E.: Assessing the impact of topography and land cover data resolutions on two-dimensional HEC-RAS hydrodynamic model simulations for urban flood hazard analysis, Nat. Hazards, 101, 995–1017, https://doi.org/10.1007/s11069-020-03906-z, 2020.
Yin, D., Xu, C., Jia, H., Yang, Y., Sun, C., Wang, Q., and Liu, S.: Sponge City Practices in China: From Pilot Exploration to Systemic Demonstration, Water, 14, 1531, https://doi.org/10.3390/w14101531, 2022.