the Creative Commons Attribution 4.0 License.
the Creative Commons Attribution 4.0 License.
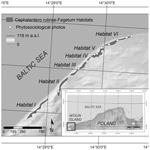
Influence of hydrometeorological hazards and sea coast morphodynamics on development of Cephalanthero rubrae-Fagetum (Wolin island, the southern Baltic Sea)
Jacek Tylkowski
Marcin Winowski
Marcin Hojan
Paweł Czyryca
Mariusz Samołyk
Climate changes, sea transgression and sea coast erosion observed today cause dynamic changes in coastal ecosystems. In the elaboration, cause and effect interrelations between abiotic hazards (hydrometeorological conditions and sea coast morphodynamics) and biotic (Cephalanthero rubrae-Fagetum phytocoenosis) components of natural environment have been defined. An up-to-date phytosociological analysis of a very valuable Cephalanthero rubrae-Fagetum site on cliff tableland was conducted in the context of hitherto temporal variability of climatic conditions and the rate of cliff coast recession. Also, the development prognosis of the researched site in the 21st century is provided, with respect to the expected climate changes and cliff's morphodynamics. The conducted research actions revealed the influence of global hazards (e.g. climate changes, sea transgression and sea coast erosion) on changes in the natural environment on regional scale (with the example of the site of Cephalanthero rubrae-Fagetum on a cliff coast of Wolin island in Poland). It has been established that in the 21st century, a relatively larger hazard to the functioning of the researched site is climate changes (i.e. mostly changes in thermal and precipitation conditions) not the sea coast erosion.
- Article
(4704 KB) - Full-text XML
- BibTeX
- EndNote
Contemporary research confirms dynamic climate changes, which are evidenced mainly by rise of temperatures (Sillmann et al., 2013). The result of thermal climate changes is the rise of the sea level by approximately 2 mm yr−1 (Church et al., 2013). The temporal variability of hydrometeorological conditions is decisive for the sea coast erosion dynamics and causes changes in coastal phytocoenoses (Strandmark et al., 2015). A particular role in this respect is reserved for extreme hydrometeorological events (Tylkowski and Hojan, 2018). Intensification of geomorphological processes, in the majority of cases, results in degradation of coastal vegetation sites (Feagin et al., 2005). Exceptionally rapid and intensive changes of the natural environment are present in moraine cliff coasts of the Baltic Sea that are poorly resistant to erosion (Kostrzewski et al., 2015). That is why empirical research on the influence of abiotic conditions on determination of the current state, threats and development perspectives of all coastal phytocoenoses is particularly important.
Unique in the world are the sites of coastal thermophilous orchid beech wood, Cephalanthero rubrae-Fagetum (Cr-F), which are found only in Poland, on the cliff coast of Wolin island, in Wolin National Park. Cr-F grows on specific soils and is a peculiar type of beech wood, recognised as a separate regional association (Matuszkiewicz, 2001, 2020). The uniqueness of this plant community stems from the endemic and specific character of habitat formation. Cr-F occurs on the top of the cliff (the so-called “cliff top”) and on cliff tableland, where unique soils rich in calcium carbonate in the form of cliff naspa were formed. Naspa's accumulation level consists in interbeddings of fine-grained sand and dust drifted by wind from eroded cliff slopes and dark-grey organic accumulation laminas rich in humus (mainly leaves of Fagus sylvatica). The cliff naspa is a soil with reaction close to neutral, rich in calcium carbonate and characterised by high porosity and efficient humification of organic remains. That is why naspa is a fertile soil. Naspa is deposited on the fossil podzolic soil. Naspa has the following sequence of soil levels: A0 litter level, A1I accumulation level of sand and organic matter layers, A1 (fos) accumulation level of fossil podzolic soil, A2 (fos) eluvial level of fossil podsolic soil, B (fos) illuvial level of fossil podzolic soil, C (fos) parent rock of fossil podzolic soil (Prusinkiewicz, 1971). Therefore, the prerequisite for the development of this phytocoenosis is its non-episodic, aeolian supply of mineral material from clayey and sandy cliff slopes. Moreover, the dynamics of cliff coast recession may not be too extensive, as spatial reach of Cr-F, counted from cliff top, is 150 m at maximum (Piotrowska, 1993). The average rate of aeolian deposition in the Cr-F habitat was 3–5 mm yr−1, and the maximum point value was 8–10 mm yr−1 (2000–2019).
Cephalanthera rubra and Epipactis atrorubens are indicator species for Cr-F (Matuszkiewicz, 2020). Both species were found in the six studied Cr-F habitats, but Cephalanthera rubra was the dominant one. Non-indicator species, e.g. Cephalanthera damasonium and Epipactis helleborine, have been found in Cr-F habitats too. The research on Cr-F conducted up to now (among others, Czubiński and Urbański, 1951; Piotrowska, 1955, 1993) was concentrated mainly on qualitative floristic and phytosociological analysis. On the other hand, the main aim of this elaboration was the up-to-date evaluation of the plant richness and floristic composition of Cr-F, and possible growth of this exceptional association, in the context of climate changes and morphodynamics of cliff coast expected to take place in this century.
The section of cliff coast in which Cr-F occurs was developed as a result of undercutting Wolin end moraine by the transgressing Baltic Sea. Ultimately, orchid beech wood sites have been developed on hinterland of moraine cliffs. Moraine cliffs at Cr-F sites are characterised by high morphological (height of 20–95 m, dominant NW exposition, inclinations up to 1∘ on cliff top and up to 88∘ on clayey slopes) and lithological (sandy sections, clayey or mixed – sandy and clayey) differentiation. The analysed section of cliff coast with the length of merely 3 km features various morphodynamic states (erosion or stagnation). The researched site type is rich in species characteristic for both forest and non-forest phytocoenoses. Forest species, characteristic for Fagetalia and Querco-Fagetea, and meadow species with Molinio-Arrhenatheretea occur in large numbers (Piotrowska, 1993). The high flow of light to the ground from the sea direction favours the occurrence on the top cliff of many heliophilous species, characteristic for meadows and psammophilous short-grass swards. Gramineous species prevail in the herb layer, among others: Brachypodium sylvatica, Dactylis glomerata, Poa nemoralis. The most valuable are orchid species, Cephalanthera damasonium, Cephalanthera rubrae and Epipactis atrorubens, which prefer fertile soils with reaction close to neutral (Piotrowska, 2003). There are, however, none of the numerous species characteristic for the Fagetalia sylvaticae order (Actaea spicata, Daphne mezereum, Lathyrus vernus, Mercurialis perennis) and Querco-Fagetea class (Aegopodium podagraria, Campanula trachelium, Corylus avellana) that feature a considerable share in all other Cephalanthero-Fagenion forests, which evidences the distinction and uniqueness of the Cr-F association (Matuszkiewicz, 2001). The source of Latin names of plant species and plant communities is the publications of Mirek et al. (2002) and Matuszkiewicz (2020).
The current reach and floristic composition of Cr-F has been determined on the basis of phytosociological mapping conducted on six study sites over 2018 and 2019 vegetative seasons. All in all, 10 detailed phytosociological images were taken with the use of the Braun–Blanquet method, and a Cr-F habitat chart on Wolin island was drafted (Fig. 1). An assumption was adopted that Cr-F site reach is determined by soil conditions. The cliff naspa determines the occurrence of Cephalanthera rubra and Epipactis atrorubens, which are species regionally characteristic of Cephalanthero rubrae-Fagetum. The site's reach limits are indicated on the basis of occurrence of Cephalanthera rubra.
Detailed recognition of hydrometeorological conditions and the recession rate of the cliff top are vastly important for the functioning of Cr-F habitats. Thermal and precipitation conditions determine water and heat resources and duration of vegetative season. On the other hand, extreme storm surges may generate intensive cliff erosion and consequently reduce the spatial extent of coastal plant communities. Therefore, unfavourable hydrometeorological conditions may limit the development of the Cr-F habitats. For the purpose of defining the long-term trend for thermal and precipitation conditions and sea level, daily hydrometeorological data in the period of 1960–2019, collected at the measurement station in Świnoujście, were used. The data were provided by the Polish Institute of Meteorology and Water Management. The meteorological and mareographical station in Świnoujście is located 15 km from the research area and provides homogeneous and complete series of actual data.
In the elaboration, a number of especially useful climatic indicators were calculated and their values compared with threshold values adequate for Fagus sylvatica given by Budeanu et al. (2016).
-
De Martonne aridity index . Here P is the amount of the annual precipitation, and T is the average annual temperature (De Martonne, 1926), with optimal thresholds for beech wood in the range of 35–40 (Satmari, 2010); De Martonne aridity index – classification by Tabari et al. (2014): IA<5 extremely arid, 5<IA<10 arid, 10<IA<20 semi-arid, 20<IA<24 Mediterranean, 24<IA<28 semi-humid, 28<IA<35 humid, 35<IA<55 very humid, 55<IA extremely humid.
-
Ellenberg quotient . Here Tw is the temperature of the warmest month of the year, and P is the annual precipitation (Ellenberg, 1988), with an optimal threshold beneficial for beech growth of below 30 and its recession threshold of above 40 (Stojanovic et al., 2013).
-
Forestry aridity index . Here TVII−VIII is the average temperature of the months July and August, PV−VII is the amount of precipitation during May–July and PVII−VIII is the amount of precipitation during July–August, with climatic conditions favouring beeches of below 4.75 (Führer et al., 2011).
-
Mayr tetratherm . Here TV−TVIII represent the mean temperature for the May–August period (Mayr, 1909), with optimal thermal conditions for beech wood of 13–18 ∘C (Satmari, 2010).
The main zone of Cr-F occurrence is the cliff top, which changes its location as a result of, among others, mass movements, water erosion and aeolian erosion. Thus, the cliff's morphodynamics is decisive for spatial reach of Cr-F. Annual measurements of the recession rate of cliff top and evolution of slope forms have been conducted since 1984 on four orchid beech wood sites (Fig. 1) (Kostrzewski et al., 2015; Winowski et al., 2019). Geomorphological changes in the cliff coast were registered a few times over a year, based on geodetic measurements, geomorphological mapping and photographic documentation collected with the use of camera traps and drones.
3.1 Hydrometeorological conditions and hazards
In the researched 60-year period (1960–2019), the mean annual air temperature reached 8.7 ∘C, with a statistically significant rising trend of 0.3 ∘C every 10 years (Fig. 3). A cooler period lasted until the end of the 1980s. Since the 1990s, a considerable warming may be observed, and an especially warm period has been the 2010s. The mean annual precipitation reached 546.7 mm. The annual sum of precipitation has not shown a statistically significant long-term trend (Fig. 3). However, for the mean and maximum annual sea level, statistically significant rising trends in their values have been observed. The mean sea level has been rising by 2 cm every 10 years, which correlates with the results of Church et al. (2013). On the other hand, the dynamics in the maximum level rise is twice as high and amounts to 4 cm every 10 years (Fig. 3). Such positive long-term trends evidence a rising threat of cliff coast abrasion in the future. The mean annual sea level in the period of 1960–2019 amounted to 501 cm, but in the last 10 years it reached 508 cm.
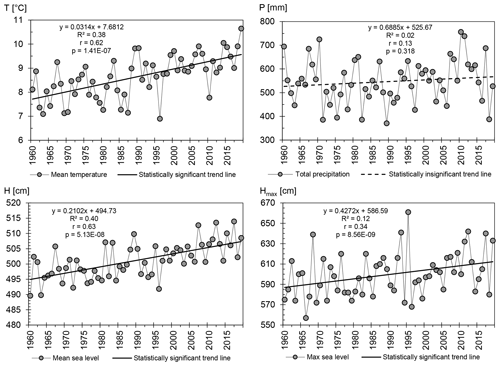
Figure 3Long-term trends in hydrometeorological conditions: annual mean air temperature (T), annual total precipitation (P), annual mean sea level (H), annual maximum sea level (Hmax) (Świnoujście, 1960–2019). (Own study based on raw data from the Institute of Meteorology and Water Management in Warsaw.)
For recognition of thermal conditions of floral growth, a detailed analysis of the thermal conditions trend may be presented with the data on vegetative season and heat resources. In Poland, the vegetative season starts when the main daily air temperature exceeds 5 ∘C. Heat resources in the vegetative season may be presented with the sum of effective temperatures, which are the sum of surpluses of the mean daily temperature exceeding 5 ∘C (Tylkowski, 2015). The vegetative season in the research area lasts, on average, 228 d; it usually starts on 30 March and ends 12 November. A statistically significant trend of extending the vegetative season by +3 d every 10 years has been proven (Fig. 4). The mean annual (1960–2019) sum of effective temperatures reached 1817 ∘C, and annual range of variability amounted to 1500 ∘C in 1967 and up to 2254 ∘C in 2018. The indicator of effective temperature sums featured a positive trend of heat resource rise of 60 ∘C every 10 years for the researched area (Fig. 4), which is a favourable condition for the growth and expansion of stenothermal species. A regularity of a considerable heat resource rise has been confirmed, especially over the last 20 years. The dynamics of increasing the heat resources, especially in the 21st century, is more noticeable than the increase in duration of the vegetative season.
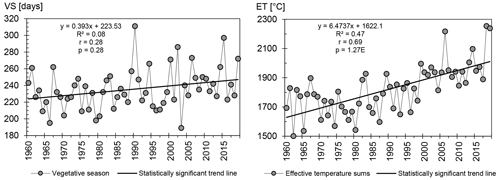
Figure 4Long-term trends in the length of vegetative season (VS) and effective temperature sums (ET) (Świnoujście, 1960–2019). (Own study based on raw data from the Institute of Meteorology and Water Management in Warsaw.)
In the last 60 years, the AI, EQ and MT indicators confirm a long-term trend of worsening climatic conditions for Cr-F (Fig. 5). The aridity index (AI) and forestry aridity index (FAI) indicators point to a statistically insignificant (p>0.05) dropping trend, and the EQ indicator shows an insignificant rising trend. The proven long-term regularities of these indicators suggest worsening thermal and precipitation conditions for the researched forest phytocoenosis in subsequent years of the 21st century. Climatic indicators will probably head towards the threshold values for sub-humid conditions (AI index), which will spur the decay of beech forest (EQ index). Unfavourable thermal conditions will grow especially rapidly in the vegetative season (MT index), for which a statistically significant rising trend (p>0.05) has been established with the value of 0.33 ∘C every 10 years (Fig. 5). Taking into account this trend's continuance in the future, it should be expected that within approximately 50 years, the thermal conditions for occurrence of Cr-F will be too excessive, and as a result, its degeneration will advance. Analysis of agroclimatic indicators (Fig. 5) showed that during phytosociological mappings of Cr-F in 2018 and 2019 highly unfavourable climatic conditions occurred for its functioning.
3.2 Cliff coast morphodynamics hazard
The mean annual rate of cliff top recession in 1984–2019 at Cr-F habitats II, III and V amounted to 0.24 m yr−1. The lowest mean annual value of cliff recession was measured for site V (0.12 m yr−1), where the cliff is built mainly of clayey sediments. The clayey sediments are characterised by relatively high resistance to degradation processes, and the reaction time of cliff top to abrasion undercuttings is extended. A large number of storms is needed for the damages to reach the cliff top. On the other hand, the highest rate of cliff erosion has been established for site III (0.31 m yr−1), where the cliff is built mainly of sandy material that is non-resistant to erosion. Sandy sediments are characterised by very low cohesion and are the subject of rapid degradation. During stormy swellings, the sandy cliffs are undercut in a short time, which favours initiation of aeolian processes (deflation) and mass movements (sheddings, slidings). The processes cause the sediments to move across the entire slope profile, and thus the reaction of cliff top to abrasion undercutting is relatively short. An increased erosion dynamics has also been observed in site II (0.27 m yr−1), on the cliff built of, both, clayey and sandy sediments. Its characteristic feature is the occurrence of underground water effluences, and high humidity of clayey sediments increases the susceptibility to landslide processes. The efficiency of the cliff springs is <1 dm3 min−1. Landslide processes generate the highest cliff's transformations, contributing to movements of its top and causing reduction of Cr-F site area. In total, over the last 35 years, the researched cliffs have recessed by an average of 7.32 m. The rate of recession of cliff top varied spatially. The largest local and pinpoint movements were measured in the western part of site II (28.44 m) (Fig. 6). In this location, owing to high activity of landslide processes, the cliff top recessed with a high rate of 0.81 m yr−1. In turn, the smallest local movements of cliff top were noted for the eastern and western parts of site V (0.30–0.42 m). In these locations, a very small rate of cliff top recession was connected with high resistance of clayey sediments to erosion processes and amounted to merely 0.01 m yr−1.
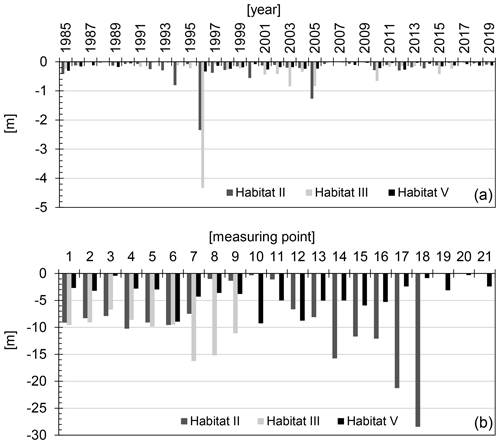
Figure 6Location changes of cliff top at sites II, III and V of Cr-F in the period of 1985–2019: A – annual mean at sites, B – multiannual total measurement points at sites. (Own study based on own measurements and raw data from Kostrzewski et al. (2015) and Winowski et al. (2019).)
Relatively lower sections of cliff coast (<30 m a.s.l.), which are primarily built of sandy formations that are not resistant to erosion, do not favour the occurrence of the Cr-F phytocoenoses. In these sections of cliff coast, the deposition of sediments containing the calcium carbonate required by the orchid beech wood is relatively small (sandy sediments contain 4–5 times less calcium carbonate 2 % than clay sediments), and an increased erosion (sandy sediments are much less resistant to erosion than clay sediments) of the coast additionally results in the reduction of the habitat's area. A different situation is with the high cliff, with a considerable share of clayey sediments. When aeolian processes occur, the dusty material, originating mainly in the clayey slope, rich in calcium carbonate, is accumulated on the cliff top and in the cliff's hinterland, causing soil deacidification. This is the condition that particularly favours the development of Cr-F habitats (e.g. habitat V). Limited occurrence of the orchid beech wood also stems from development cycles of the cliff coast. For the sandy and dusty material – that is the components of the cliff naspa – to be supplied, morphogenetic activity at the cliff's slope is required. Only then are material deflation from the cliff's slope and its subsequent aeolian deposition in the cliff's hinterland possible. Thus, the aeolian deposition is indispensable for the formation and development of the cliff naspa for inland. When the cliff coast, over an extended period of time, is not subject to processes of maritime abrasion and slope erosion, then its slope is covered with vegetation under biocenotic succession. The vegetation considerably hinders, and even renders impossible, the supply of aeolian matter and consequently the formation of cliff naspa, which in the long-term spurs the decay of the Cr-F phytocoenoses (e.g. habitat I). That is, the occurrence of the active morphogenetic processes of small intensity is desirable (e.g. at habitat V; mean annual rate of cliff top recession in the last 35 years amounted to “as little as” 0.12 m yr−1). The dynamics of coast recession may not, however, be too intensive and exceed the natural expansion of the cliff naspa and Cephalanthero rubrae-Fagetum habitat for the inland direction. Then, the decrease in habitat area is spurred (e.g. in habitat III; mean annual rate of cliff top recession in the last 35 years has been considerable and amounted to 0.32 m yr−1). Therefore, the optimal morpholitodynamic conditions for the growth of Cr-F are found mainly in habitat V. Similar conditions are found in habitats II and IV. In the remaining habitats of the Cr-F phytocoenoses, the morpholitodynamic conditions are rather unfavourable – too much (habitat III) or too little (habitat I) cliff erosion.
3.3 Plant species richness and floristic composition of Cr-F
Currently, Cr-F grows along the northern cliff coast of Wolin island, between Biała Góra and Grodno, in six isolated sites with a total area of merely 7.3 ha. The researched phytocoenosis occurs over a short 3 km section of the coast, in the form of a narrow belt of approximately 100 m for inland between the cliff's edge and a complex of lowland acidophilous beech forest, Luzulo pilosae-Fagetum.
The floral richness of Cr-F association consists of 113 species of vascular plants. They represent two divisions – Pteridophyta and Spermatophyta. In Pteridophyta four species have been recorded: Dryopteris carthusiana, Dryopteris filix-mas, Polypodium vulgare and Pteridium aquilinum. In Spermatophyta three classes have been confirmed: Pinopsida (two species: Juniperus communis and Pinus sylvestris), Magnoliopsida (23 orders, 29 families and 81 species) and Liliopsida (respectively 3, 6 and 26). The richest in species have been the families of Poaceae (14 species), Asteraceae (13), Fabaceae (11) and Rosaceae (6). Orchidaceae has been represented by seven species: Cephalanthera damasonium, Cephalanthera rubra, Corallorhiza trifida, Epipactis atrorubens, Epipactis helleborine, Neottia nidus-avis and Platanthera bifolia. The researched site is an example of a coexistence between forest species of fertile and acidic beech woods, acidophilic oak woods and forests, and species of meadows and psammophilous swards. There have observed species from syntaxa: Artemisietea vulgaris, Festuco-Brometea, Molinio-Arrhenatheretea, Querco-Fagetea and Vaccinio-Piceetea.
-
Habitat I. The cliff slope is not subject to erosion processes, and for over 35 years it has been the so-called “dead cliff”. Therefore, aeolian deposition on the cliff top is very limited and the Cr-F habitat decays. The soil profile and the presence of calcium carbonate in surface sediments confirm the presence of cliff naspa and morphodynamic activity of this cliff section in the past. On the cliff top, there are only six Cephalanthera rubra specimens (Table 1), which are relics of a once well-developed habitat. There are no other orchid species found, though. The ground cover was poor (<5 % coverage in the herb layer), and the confirmed species of Luzula pilosa and Trientalis europaea are the distinguishing species of the Luzulo-Fagenion beech forests.
-
Habitat II. In terms of phytosociology, this is a phytocoenosis of Cr-F typicum. The cliff wall is predisposed to aeolian processes as it is exposed and morphogenetically active. The ground cover is rich in species. The highest number (97) of vascular plants species was found in this habitat (Table 1). There is a high concentration of Cephalanthera rubra (44 shoots per hectare) and four orchid species have been found: Cephalanthera damasonium, Cephalanthera rubra, Epipactis helleborine and Epipactis atrorubens. There are also numerous species of the Poaceae family (among others, Brachypodium sylvaticum, Calamagrostis arundinacea, Deschampsia flexuosa, Poa nemoralis). Density of beech heads at this site is low (approximately 50 %), and light conditions are favourable for the development of the ground cover (94 % coverage in the herb layer), rich in species. A large portion (20 %) of the site is covered by beech brushwood, which evidences an intensive renewal of forest.
-
Habitats III and IV. The plant indicators in Table 1 show that the habitats are moderately formed. At habitat III, there are intensive erosion processes taking place. Despite the aeolian deposition on the cliff top (40 m a.s.l.) being high, due to a relatively high rate of cliff recession (0.31 m yr−1) the site's reach in this location decreases. The ground cover is well developed, and there are four species of Orchidaceae: Cephalanthera rubra, Epipactis atrorubens, Epipactis helleborine and Neottia nidus-avis. They are, however, quite diffused and occur in a relatively narrow (Cephalanthera rubra density 31 shoots per hectare) strip along the cliff top (max 40 m). However, habitat IV is a very small (0.1 ha), isolated area, where five shoots of Cephalanthera rubra have been found.
-
Habitat V. The biggest patch of Cr-F typicum is very well developed (Table 1). The cliff's wall is exposed, and high (35–50 m a.s.l.) aeolian deposition on the cliff top is visible. Aeolian material is visible on plants and the ground surface. The increment of aeolian cover in the soil profile is about 4 mm yr−1 in 2000–2019. The ground cover is well developed (57 % coverage in the herb layer) and rich in species (73), although in some areas their number drops due to poorer light conditions (high coverage of forest canopy). There is a high abundance of Cephalanthera rubra (51), as well as other orchid species. This site strongly encroaches inland. Species regionally characteristic for Cr-F have been found even up to 100 m from the cliff's edge. Even in this zone there were orchids, but their numbers were smaller than at the cliff. In total, six species of Orchidaceae have been identified: Cephalanthera damasonium, Cephalanthera rubra, Epipactis atrorubens, Epipactis helleborine, Neottia nidus-avis and Platanthera bifolia.
-
Habitat VI. This habitat may also be considered a patch of Cr-F typicum (Table 1), but a smaller concentration of Cephalanthera rubra (15 shoots per hectare) has been confirmed there. The cliff is mostly clayey and low (25–30 m a.s.l.); thus the intensity of aeolian deposition is relatively smaller (2 mm yr−1 in 2000–2019). The cliff tableland is flat. And the ground cover covers up to 90 % of the phytocoenose area and is rich in species typical for orchid beech wood. There have been five orchid species from Cephalanthero-Fagenion confirmed: Cephalanthera damasonium, Cephalanthera rubra, Corallorhiza trifida, Epipactis atrorubens and Epipactis helleborine.
The most valuable orchid beech wood habitats are II, V and VI. Habitat V is the best developed patch of Cr-F, with optimal habitat conditions: favourable morpholitodynamic conditions (abrasive coast but low rate of cliff recession 0.12 m yr−1, higher share of clay sediments, rich in calcium carbonate 8 %–10 %); favourable light conditions (relatively greater insolation of the forest floor); ground cover of orchid beech wood (developing inland for a dozen or so metres in some points). The relatively poorest condition was confirmed for habitat I, which does not develop due to unfavourable morpholithodynamic conditions (dead non-erosive cliff, stabilised with compact pine wood, no possibility of forming naspa).
The current condition and future development of coastal phytocoenoses depends, primarily, on changes in climatic conditions and morphodynamics of sea coasts. In the 21st century, in the Polish coastal zone of the Baltic Sea, the mean annual air temperature may rise by 2–3 ∘C, with a concurrent rise in total precipitation of 0 %–10 % during summer and 10 %–20 % during winter (Collins et al., 2013). Many research works indicate that in the last half century, as a result of global warming (Sillmann et al., 2013) on increase in activity of cyclones has occurred, and the frequency of western winds in northern Europe (Pinto et al., 2007) and over the Baltic Sea region (Sepp, 2009) has increased. Another of the observed changes is the northward displacement of trajectories of lows, which may cause advection of warm and humid air to northern Europe and decrease in precipitation in central Europe (Bengtsson et al., 2006). The changes are connected with a varied location of the Icelandic Low and the North Atlantic oscillation (NAO) (Omstedt et al., 2004). In the Baltic Sea catchment area, the warming will probably be higher than the mean global value, and the air temperature rise will, probably, be accompanied by higher precipitation, especially in winter. Also, the rise in frequency and duration of droughts (Orlowsky and Seneviratne, 2012) and heat-waves (Nikulin et al., 2011) is also expected. In the 21st century, the forecast climate changes will be accompanied by the rise in sea levels of up to 1 m, and absolute rise of the Baltic Sea level is estimated to reach 80 % of the mean rise of the world ocean level. For the south-west coast of the Baltic Sea, the estimated rise in water level would be high, reaching approximately 60 cm (Grinsted, 2015). The executed hydrodynamic modelling iterations also assume the rise in frequency of stormy swellings for the entire Baltic Sea, in all seasons (Vousdoukas et al., 2016). Changes of the climate and hydrodynamic characteristics of seas will favour the high frequency of extreme hydrometeorological events. In Poland, for the Baltic coasts, over the last half century, a rise in the frequency of extreme hydrometeorological events has been confirmed (Paprotny and Terefenko, 2017; Tylkowski and Hojan, 2018). Extremely high stormy swellings and precipitation intensify hydrological and geomorphological process, e.g. stormy floods or mass movements at cliff coasts. For the Polish coastal zone of the Baltic Sea, the occurrence of such unfavourable geomorphological results of extreme and above-average hydrometeorological events has been confirmed for both cliff and dune coasts (Florek et al., 2009; Furmańczyk et al., 2012; Hojan et al., 2018; Kostrzewski and Zwoliński, 1995; Tylkowski, 2017, 2018).
Climate changes in the 21st century will cause dynamic changes in the reach of forest phytocoenoses, including Fagus sylvatica. The forecast warming and gradual deterioration of water conditions in the coming 50 years will not considerably influence the changes in beech forest sites, yet. But from 2070 onwards, climatic conditions will be too warm and too dry for the growth of Fagus sylvatica, and this species will start to withdraw from the area of research (Falk and Hempelmann, 2013). The above forecast corresponds to the long-term trend of the agroclimatic indicators presented in the elaboration, especially with Mayr tetratherm index. According to the forecast variability of this indicator, in 50 years, climatic conditions will not be suitable for the development of the Cr-F habitat.
In the analysed period (1985–2019), the average annual rate of the cliff crown retraction on the examined sections amounted to 12 up to 31 cm, and it was much lower than the values estimated (80–100 cm) by the mid-20th century by Subotowicz (1982) and Kostrzewski (1984). Whereas the maximum annual point retraction of the cliff crown was almost 10 m. The average annual retraction rate of the Wolin cliffs is approximately 2–4 times lower than other monitored cliff coasts, e.g. in the vicinity of Ustka, Jastrzębia Góra or Gdynia (e.g. Florek et al., 2009; Łęczyński, 1999). Although the Wolin cliffs are much higher and are not subjected to any protective measures, the relatively lowest rate of their retraction results primarily from specific hydrogeological conditions. For example, contrary to the cliff coast in Jastrzębia Góra (Uścinowicz et al., 2017) on the island of Wolin, underground waters practically do not play any role in erosion processes and shore degradation.
Species composition of association's phytocoenoses has not changed extensively over the last half century (Piotrowska, 1993; Prusinkiewicz, 1971), which confirms its relative stability; however, some Orchidaceae habitats do not keep up with the rate of the cliff's recession or they do not develop due to many years of cliff erosive stagnation. No specimens of Malaxis monophyllos were confirmed, which was occurring at the cliff's edge tens of years ago (Piotrowska, 1993). A vast loss for the site is also the lack of current confirmation for the occurrence of Listera ovata. Also, it has been confirmed that the number of Lonicera xylosteum decreased – a species important for the orchid beech wood. In past elaborations, the indicatory species of Cephalanthera rubra featured a larger reach in the area of Wolin National Park, e.g. in the forest divisions of Międzyzdroje 16 and Wisełka 2. Currently, no specimens of Cephalanthera rubra have been found at those sites, which is the confirmation for the decreasing reach of this species in Wolin National Park.
The analysis of Cr-F habitats indicated its small total area of merely 7.3 ha. This valuable site consists of six isolated, single sites with an area of 1.7 ha to just 0.1 ha. Discontinuity of the site stems from many natural factors — mainly due to the spatial variability of the cliff's morpholitodynamics. Phytosociological studies evidenced relatively good condition of Cr-F in majority of sites.
The analysis of temporal variability of hydrometeorological conditions, duration of the vegetative season and heat resources (1960–2019), and cliff coast morphodynamics (1985–2019) has indicated, up to now, rather favourable conditions for the growth of Cr-F. A statistically significant trend of the increase in mean annual air temperature, sea level, duration of the vegetative season and heat resources has been verified. Analysis of climatic indicators AI, EQ and FAI in the last 60 years has not evidenced a trend of unfavourable climatic conditions clustering, and the occurrence of unfavourable thermal and precipitation conditions was of random character. Only the analysis of the MT indicator pointed to an alarming and statistically significant rise in its value. It must be stressed that as of now the regularities in long-term changes of AI and EQ indicators are unfavourable. Climatic conditions at the end of the 21st century may be too warm for Fagetum type forests, which – concurrently with uncertainty of precipitation efficiency and their time distribution – will intensify evapotranspiration and draught. It seems that climatic conditions of the southern Baltic Sea are heading for change in the 21st century from humid to subhumid, and in the long term, to Mediterranean (IA index). Therefore, it is possible that access to water will be limited and may influence a drastic change in the conditions of Cr-F.
As a result of global warming, the sea level rises, and in the future, this may be the cause of intensified coastal erosion. The current cliff erosion rate is 0.3 m yr−1. Thus, in the coming decade, the morphodynamic processes should not cause sudden degradation in the reach of Cr-F site. The long term, the dynamics definition of these processes is very difficult without precise recognition of submarine slope configuration and functioning of the circulatory cell system. Erosion processes of the cliff coast are taking place over various time and spatial scales, and the highest erosion intensity is featured during extreme events that cannot be predicted. But, taking into account the increasing frequency of the maximum level of the Baltic Sea and stormy swellings, the erosion intensification of the sea coast may be expected. The development of the Cr-F site is highly conditioned by the presence of cliff naspa and its formation due to aeolian processes. The cliff's erosive activity is a favourable condition for the development of the analysed site only to a certain degree. High activity of morphodynamic processes influences the high rate of cliff top recession, and this, in turn, contributes to the loss of Cr-F phytocoenoses. On the other hand, the limited influence of the morphogenetic process favours the cliff's stabilisation and sprouting of vegetation, and thus the Cr-F site does not develop. Therefore, the optimal condition for the development of Cr-F is the balanced cliff's dynamics. This notion is, however, difficult to be defined quantitatively due to high morpholithological diversity of cliffs. The simplest assumption is that the optimal condition for the growth of the orchid beech wood is the case in which the cliff top recesses with a small but stable rate of up to, approximately, 0.15 m yr−1.
Future existence of Cr-F depends, primarily, on climatic conditions and, to a lesser extent, on erosive processes on the cliff coast. Taking into account that Cr-F sites are found in the strict nature reserve of Wolin National Park, there is no need to introduce special protection measures. A favourable condition is the lack of cliff coast protection against erosive processes. Full limitation of the cliff's erosion would result in a lack of cliff naspa formation. As evidenced by multiannual field research that has been conducted until now, more favourable conditions for the development of Cr-F are found in the cliff coast zone in the erosion phase, and not stagnation, as the benefits stemming from aeolian accumulation and formation of cliff naspa outweigh the losses in coastline due to cliff top recession.
Data in this paper can be made available for scientific use upon request to the authors.
JT designed the research with participation of all the authors. JT and MW compiled data and conducted hydrometeorological and sea coast morphodynamics analyses. PC compiled data and conducted phytosociological analysis. All other authors contributed with data or conducted a small part of data compilation or analysis. JT drafted the paper with participation from MH and comments from all authors.
The authors declare that they have no conflict of interest.
The authors would like to thank the Polish Institute of Meteorology and Water Management in Warsaw for the provided hydrometeorological data. We would also like to thank the management of Wolin National Park, Marek Dylawerski and Stanisław Felisiak, for their consent and assistance in scientific research. We also thank the Natura company, especially Wojciech Zyska, for his help in drafting this elaboration.
The research was supported mainly by the Forest Fund, within the scope of funding admitted by the Directorate General of State Forests National Forest Holding for Wolin National Park (grant no. EZ.0290.1.21.2019 of 22 July 2019).
This paper was edited by Mauricio Gonzalez and reviewed by Tomasz Wolski and Janina Borysiak.
Bengtsson, L., Hodges, K., and Roeckner, E.: Storm tracks and climate change, J. Climate, 19, 3518–3543, https://doi.org/10.1175/JCLI3815.1, 2006.
Budeanu, M., Petritan, A. M., Popescu, F., Vasile, D., and Tudose, N. C.: The resistance of european beech (Fagus Sylvatica) from the eastern natural limit of species to climate change, Not. Bot. Horti Agrobo., 44, 625–633, https://doi.org/10.15835/nbha44210262, 2016.
Church, J. A., Clark, P. U., Cazenave, A., Gregory, J. M., Jevrejeva, S., Levermann, A., Merrifield, M. A., Milne, G. A., Nerem, R. S., Nunn, P. D., Payne, A. J., Pfeffer, W. T., Stammer, D., and Unnikrishnan, A. S.: Sea Level Change, in: Climate Change 2013: The Physical Science Basis, Contribution of Working Group I to the Fifth Assessment Report of the Intergovernmental Panel on Climate Change, edited by: Stocker, T. F., Qin, D., Plattner, G.-K., Tignor, M., Allen, S. K., Boschung, J., Nauels, A., Xia, Y., Bex, V., and Midgley, P. M., Cambridge University Press, Cambridge, UK and New York, USA, 1137–1216, 2013.
Collins, M., Knutti, R., Arblaster, J., Dufresne, J. L., Fichefet, T., Friedlingstein, P., and Wehner, M.: Long-term climate change: Projections, commitments and irreversibility, in: Climate Change 2013: The Physical Science Basis, Contribution of Working Group I to the Fifth Assessment Report of the Intergovernmental Panel on Climate Change, edited by: Stocker, T. F., Qin, D., Plattner, G.-K., Tignor, M., Allen, S. K., Boschung, J., Nauels, A., Xia, Y., Bex, V., and Midgley, P. M., Cambridge University Press, Cambridge, UK and New York, USA, 1029–1136, 2013.
Czubiński, Z. and Urbański, J.: Park Narodowy na wyspie Wolin, Chrońmy Przyrodę Ojczystą, 7, 1–64, 1951.
De Martonne, E.: Une nouvelle fonction climatologique: L'indice d'aridité, La Meteorologie, 2, 449–458, 1926.
Ellenberg, H.: Vegetation Ecology of Central Europe, Cambridge University Press, Cambridge, UK, 1988.
Falk, W. and Hempelmann, N.: Species Favourability Shift in Europe due to Climate Change: A Case Study for Fagus sylvatica L. and Picea abies (L.) Karst. Based on an Ensemble of Climate Models, J. Climatol., 2013, 787250, doi.org/10.1155/2013/787250, 2013.
Feagin, R. A., Sherman, D. J., and Grant, W. E.: Coastal erosion, global sea-level rise, and the loss of sand dune plant habitats, Front. Ecol. Environ., 3, 359–364, https://doi.org/10.1890/1540-9295(2005)003[0359:CEGSRA]2.0.CO;2, 2005.
Florek, W., Kaczmarzyk, J., and Majewski, M.: Intensity and character of cliff evolution near Ustka, Quaestiones Geographicae, 28, 27–38, 2009.
Führer, E., Horváth, L., Jagodics, A., Machon, A., and Szabados, I.: Application of a new aridity index in Hungarian forestry practice, Idöjárás, 115, 205–216, 2011.
Furmańczyk, K. K., Dudzińska-Nowak, J., Furmańczyk, K. A., Paplińska-Swerpel, B., and Brzezowska, N.: Critical storm thresholds for the generation of significant dune erosion at Dziwnow Spit, Poland, Geomorphology, 143/144, 62–68, https://doi.org/10.1016/j.geomorph.2011.09.007, 2012.
Grinsted, A.: Projected Change-Sea Level, in: Second Assessment of Climate Change for the Baltic Sea Basin, Springer, London, UK, 253–263, https://doi.org/10.1007/978-3-319-16006-1, 2015.
Hojan, M., Tylkowski, J., and Rurek, M.: Hydrometeorological conditions for the occurrence of aeolian processes on the Southern Baltic coast in Poland, Water, 10, 1745, https://doi.org/10.3390/w10121745, 2018.
Kostrzewski, A.: Rozwój wybrzeży klifowych wyspy Wolin w oparciu o materiały archiwalne, Sprawozdania PTPN, 100, 129–132, 1984.
Kostrzewski, A. and Zwoliński, Z.: Present-day morphodynamics of the cliff coasts of Wolin Island, J. Coastal Res., 22, 293–303, 1995.
Kostrzewski, A., Zwoliński, Z., Winowski, M., Tylkowski, J., and Samołyk, M.: Cliff top recession rate and cliff hazards for the sea coast of Wolin Island (Southern Baltic), Baltica, 28, 109–120, https://doi.org/10.5200/baltica.2015.28.10, 2015.
Łęczyński, L.: Morpholitodynamics of the shoreface on the cliff coast at Jastrzębia Góra, Peribalticum, 7, 9–20, 1999.
Matuszkiewicz, W.: The systematic position of termophilous beechwoods (Cephalanthero-Fagenion) in Poland, Fragmenta Floristica et Geobotanica, 45, 1–2, 2001.
Matuszkiewicz, W.: Przewodnik do oznaczania zbiorowisk leśnych Polski, Wydawnictwo Naukowe PWN, Warszawa, 1-540, ISBN 978-83-01-16707-3, 2020.
Mayr, H.: Waldbau auf naturgesetzlicher Grundlage, Verlag Paul Perey, Berlin, Germany, 1909.
Mirek, Z., Piękoś-Mirkowa, H., Zając, A., and Zając, M.: Flowering plants and pteridophytes of Poland. A checklist, W. Szafer Institute of Botany, Polish Academy of Sciences, Kraków, 1-442, ISBN 83-85444-83-1, 2002.
Nikulin, G., Kjellström, E., Hansson, U., Jones, C., Strandberg, G., and Ullerstig, A.: Evaluation and future projections of temperature, precipitation and wind extremes over Europe in an ensemble of regional climate simulations, Tellus A, 63, 41–55, https://doi.org/10.1111/j.1600-0870.2010.00466.x, 2011.
Omstedt, A., Pettersen, C., Rohde, J., and Winsor, P.: Baltic Sea climate: 200 yr of data on air temperature, sea level variation, ice cover and atmospheric circulation, Clim. Res., 25, 205–216, 2004.
Orlowsky, B. and Seneviratne, S. I.: Global changes in extreme events: Regional and seasonal dimension, Climate Change, 116, 669–696, https://doi.org/10.1007/s10584-011-0122-9, 2012.
Paprotny, D. and Terefenko, P.: New estimates of potential impacts of sea level rise and coastal foods in Poland, Nat. Hazards, 85, 1249–1277, https://doi.org/10.1007/s11069-016-2619-z, 2017.
Pinto, J. G., Ulbrich, U., Leckebusch, G. C., Spangehl, T., Reyers, M., and Zacharias, S.: Changes in storm track and cyclone activity in three SRES ensemble experiments with the ECHAM5/MPI-OM1 GCM, Clim. Dynam., 29, 195–210, https://doi.org/10.1007/s00382-007-0230-4, 2007.
Piotrowska, H.: Zespoły leśne wyspy Wolin, Prace Komisji Biologii PTPN, 16, 3–169, 1955.
Piotrowska, H.: Buczyna storczykowa wzdłuż nadmorskiego klifu na wyspie Wolin (północno-zachodnia Polska), Zeszyty Naukowe UG, Biologia, 10, 5–29, 1993.
Piotrowska, H.: Zróżnicowanie i dynamika nadmorskich lasów i zarośli w Polsce, Bogucki Wydawnictwo Naukowe, Poznań, Poland, 1–104, ISBN 83-89290-17-0, 2003.
Prusinkiewicz, Z.: Naspy przyklifowe – nowy typ gleb morskiego pobrzeża, Zeszyty Naukowe UMK w Toruniu, Geografia, 26, 133–157, 1971.
Satmari, A.: Lucrari practice de biogeografie (Practical applications of biogeography), available at: http://www.academia.edu/9909429/05_indici_ecometrici (last access: 26 November 2019), 2010.
Sepp, M.: Changes in frequency of Baltic Sea cyclones and their relationships with NAO and climate in Estonia, Boreal Environ. Res., 14, 143–151, 2009.
Sillmann, J., Kharin, V. V., Zhang, X., Zwiers, F. W., and Bronaugh, D.: Climate extremes indices in the CMIP5 multimodel ensemble: Part 2. Future climate projections, J. Geophys. Res.-Atmos., 118, 2473–2493, https://doi.org/10.1002/jgrd.50188, 2013.
Stojanović, D., Kržič, A., Matović, B., Orlović, S., Duputie, A., Djurdjević, V., Galić, Z., and Stojnić, S.: Prediction of the European beech (Fagus sylvatica) xeric limit using a regional climate model: An example from southeast Europe, Agr. Forest Meteorol., 176, 94–103, https://doi.org/10.1016/j.agrformet.2013.03.009, 2013.
Strandmark, A., Bring, A., Cousins, S. A. O., Destouni, G., Kautsky, H., Kolb, G., de la Torre-Castro, M., and Hambäck, P. A.: Climate change effects on the Baltic Sea borderland between land and sea, AMBIO, 44, 28–38, https://doi.org/10.1007/s13280-014-0586-8, 2015.
Subotowicz, W.: Litodynamika brzegów klifowych wybrzeża Polski, Gdańskie Towarzystwo Naukowe, Ossolineum, Wrocław, ISBN 83-04-01301-0, 150, 1982.
Tabari, H., Talaee, H., Nadoushani, M., Wilems, P., and Marchetto, A.: A survey of temperature and precipitation based aridity indices in Iran, Quatern. Int., 345, 158–166, https://doi.org/10.1016/j.quaint.2014.03.061, 2014.
Tylkowski, J.: The variability of climatic vegetative seasons and thermal resources at the Polish Baltic Sea coastline in the context of potential composition of coastal forest communities, Balt. For., 21, 73–82, 2015.
Tylkowski, J.: The temporal and spatial variability of coastal dune erosion in the Polish Baltic coastal zone, Baltica, 30, 97–106, https://doi.org/10.5200/baltica.2017.30.11, 2017.
Tylkowski, J.: Hydrometeorologiczne uwarunkowania erozji wybrzeża klifowego wyspy Wolin, Przegląd Geograficzny, 90, 111–135, https://doi.org/10.7163/PrzG.2018.1.6, 2018.
Tylkowski, J. and Hojan, M.: Threshold values of extreme hydrometeorological events on the Polish Baltic coast, Water, 10, 1337, https://doi.org/10.3390/w10101337, 2018.
Uścinowicz, G., Jurys, L., and Szarafin, T.: The development of unconsolidated sedimentary coastal cliffs (Pobrzeże Kaszubskie, Northern Poland), Geol. Q., 61, 491–501, 2017.
Vousdoukas, M. I., Voukouvalas, E., Annunziato, A., Giardino, A., and Feyen, L.: Projections of extreme storm surge levels along Europe, Clim. Dynam., 47, 3171–3190, https://doi.org/10.1007/s00382-016-3019-5, 2016.
Winowski, M., Kostrzewski, A., Tylkowski, J., and Zwoliński, Z.: The importance of extreme processes in the development of the Wolin Island cliffs coast (Pomeranian Bay-Southern Baltic), in: Proceedings of the International Scientific Symposium New Trends In Geography, Ohrid, Republic of North Macedonia, 3–4 October 2019, 99–108, 2019.