the Creative Commons Attribution 4.0 License.
the Creative Commons Attribution 4.0 License.
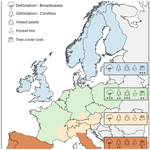
Impacts on and damage to European forests from the 2018–2022 heat and drought events
Florian Knutzen
Paul Averbeck
Caterina Barrasso
Laurens M. Bouwer
Barry Gardiner
José M. Grünzweig
Sabine Hänel
Karsten Haustein
Marius Rohde Johannessen
Stefan Kollet
Mortimer M. Müller
Joni-Pekka Pietikäinen
Karolina Pietras-Couffignal
Joaquim G. Pinto
Diana Rechid
Efi Rousi
Ana Russo
Laura Suarez-Gutierrez
Sarah Veit
Julian Wendler
Elena Xoplaki
Daniel Gliksman
Drought and heat events in Europe are becoming increasingly frequent due to human-induced climate change, impacting both human well-being and ecosystem functioning. The intensity and effects of these events vary across the continent, making it crucial for decision-makers to understand spatial variability in drought impacts. Data on drought-related damage are currently dispersed across scientific publications, government reports, and media outlets. This study consolidates data on drought and heat damage in European forests from 2018 to 2022, using Europe-wide datasets including those related to crown defoliation, insect damage, burnt forest areas, and tree cover loss. The data, covering 16 European countries, were analysed across four regions, northern, central, Alpine, and southern, and compared with a reference period from 2010 to 2014.
Findings reveal that forests in all zones experienced reduced vitality due to drought and elevated temperatures, with varying severity. Central Europe showed the highest vulnerability, impacting both coniferous and deciduous trees. The southern zone, while affected by tree cover loss, demonstrated greater resilience, likely due to historical drought exposure. The northern zone is experiencing emerging impacts less severely, possibly due to site-adapted boreal species, while the Alpine zone showed minimal impact, suggesting a protective effect of altitude.
Key trends include (1) significant tree cover loss in the northern, central, and southern zones; (2) high damage levels despite 2021 being an average year, indicating lasting effects from previous years; (3) notable challenges in the central zone and in Sweden due to bark beetle infestations; and (4) no increase in wildfire severity in southern Europe despite ongoing challenges.
Based on this assessment, we conclude that (i) European forests are highly vulnerable to drought and heat, with even resilient ecosystems at risk of severe damage; (ii) tailored strategies are essential to mitigate climate change impacts on European forests, incorporating regional differences in forest damage and resilience; and (iii) effective management requires harmonised data collection and enhanced monitoring to address future challenges comprehensively.
- Article
(1744 KB) - Full-text XML
-
Supplement
(379 KB) - BibTeX
- EndNote
1.1 General introduction
The global temperature rise due to the accumulation of anthropogenic greenhouse gases in the atmosphere causes extreme drought and heat events to become more likely and extreme (Seneviratne et al., 2021). Even if we manage to stay below the 2 °C global warming threshold by the end of the 21st century (relative to pre-industrial levels), in Europe one out of every two summer months is projected to be as warm as or warmer than the summer of 2010, which was one of the warmest across Europe to date (Suarez-Gutierrez et al., 2018). Furthermore, the likelihood of such extremely warm summers co-occurring with extreme drought conditions over Europe is increasing rapidly (Suarez-Gutierrez et al., 2023). When extreme heat occurs jointly with severe drought conditions, it can lead to devastating ecological and socioeconomic impacts (Feller et al., 2017; Zscheischler et al., 2020; Bastos et al., 2021), such as economic losses (García-León et al., 2021), increased risk of wildfires (Ruffault et al., 2020), crop loss (Brás et al., 2021; Bento et al., 2021), and unprecedented forest mortality events (Schuldt et al., 2020). Extreme drought is often closely linked with extreme heat, which in turn increases heat-related mortality and morbidity (Watts et al., 2021). Vicedo-Cabrera et al. (2021) found that up to 30 % of heat-related deaths globally in the last 30 years can be attributed to anthropogenic climate change. Mitchell et al. (2018) found that the risk of heat-related human mortality during the intense 2003 summer heat wave increased in central Paris by ∼70 % and by ∼20 % in London, both attributable to human factors having exacerbated the likelihood for such events. As such, the recent period of drought and heat between 2018 and 2022 is especially concerning, as it could be the beginning of a new climatic era in Europe.
The recent hot and dry extremes are part of a long-term trend being observed in Europe over the last 42 years, making it a kind of hot spot for heatwaves in comparison to other regions of the Northern Hemisphere midlatitudes (Rousi et al., 2022). Central and southern Europe are affected by a longer-term drying trend, in line with expectations from theory and climate model simulations (Ionita et al., 2022). This trend also includes consecutive multiyear meteorological summer droughts, such as those of 2018 to 2022 in central and western Europe, which are characterised by two or more summers of lower-than-normal precipitation and higher-than-normal evaporative demand, resulting in a larger reduction in soil moisture content in the second year of the drought and therefore in potentially more-extreme drought impacts (Van Der Wiel et al., 2022). Worryingly, climate models project a strong increase in dry spells (Rousi et al., 2021) and multiyear droughts in western Europe in response to further global warming (Van Der Wiel et al., 2022; Suarez-Gutierrez et al., 2023).
1.2 Scope, aims, and research approach
In this study, we present the impacts documented in European forests during the years 2018–2022, among the warmest and driest on record over Europe (Fig. 1). We focus on forest ecosystems to reduce the risk of bias that could arise from variations in irrigation practices, allowing us to better observe the effects of climate extremes. Furthermore, forests are essential to our livelihoods; they provide wood as a renewable raw material and offer a range of vital ecosystem services. For example, forests contribute significantly to maintaining biodiversity, sequestering carbon, mitigating climate change, preventing land degradation, and offering recreational value (e.g. Jenkins and Schaap, 2018).
We partitioned the forest environment of Europe into four main geographical zones with distinct climatic and environmental conditions: (1) northern Europe, (2) central Europe, (3) the Alpine zone, and (4) southern Europe. The four geographical zones do not overlap in all cases with the international borders. Thus, since some of the information sources (e.g. government reports) used for this study refer to political boundaries (at the country level), we assigned those sources to the most appropriate geographical zone. An exception was made for countries that fall within two zones, as they partly overlap with the Alpine zone (see Table 1). The Alpine zone is defined based on the 28 Nomenclature of territorial Units for Statistics (NUTS) 2 regions outlined in the area covered by the Alpine Convention (Alpconv, 2018).
The evaluation of the extraordinarily intense drought and heat events between 2018 and 2022, along with the impacts, was derived using an interdisciplinary approach integrating different information sources that allow for the assessment of temporal and spatial heterogeneity impacts. We start with the description of the climatic conditions in 2018–2022, with a focus on drought and high temperatures. We describe droughts in the years prior to 2018 to provide a better context for our focal period, 2018–2022. Following this, we focus on the heat and drought impacts on forests and their legacy effects. We collected the damage estimates from research papers, reports, and even media coverage when no other source was available. We focus our assessment on damage caused by drought and heat that induced (i) physiological stress, (ii) insect pest incidents, and (iii) fire events, as these are the three most-well-documented impacts in our sources.
The data sources often posed issues and challenges. Concerning fire events, we focus on forest fires, which are defined as uncontrolled fires occurring in areas that are at least partly forested. However, for some countries, only statistics on all-vegetation and uncontrolled wildfires were available. Additionally, the online data from the European Forest Fire Information System (EFFIS) provide information on the number of wildfires and the total affected vegetation area. To resolve these issues, we used data on forest fires (when available) and clearly indicated when the information pertains to wildfires. Although this study examines forest damage spanning 2018–2022, the exceptional forest fire damage in 2017 in southern Europe was also included to provide context for subsequent damage. Post-2017, significant management measures were implemented in southern Europe to mitigate forest fires, affecting subsequent damage trends (e.g. REA, 2024). Forest damage in other zones is not discussed for 2017 as it was comparatively minimal.
In order to evaluate and provide attribution for the impacts of heat and drought during the years 2018 to 2022, we considered a reference period spanning 5 years from 2010 to 2014. The year 2015 was regarded as an extraordinary drought year in Europe (e.g. Hoy et al., 2017; Laaha et al., 2017), and thus it was not included in our reference period. Compared to other periods in the current millennium, the years between 2010 and 2014 were characterised by fewer climate extremes, large-scale droughts, or severe floods. For example, in Germany, the water balance levels show only small deviations from the climatological mean during that period (see DWD Dokumentation SPEI). The period of 2010–2014 experienced below-average to average annual mean temperatures across Europe relative to the 1991–2020 average, particularly in the years 2010, 2012, and 2013 (IMKTRO, 2023a; IMKTRO, 2023b; EC-JRC Drought Reports, 2024). Moreover, damage data availability was sufficient for the period of 2010–2014.
Countries were selected based on their exposure to heat and drought during 2018–2022, as well as on data availability and language barriers (Table 1). Therefore, out of the 44 European countries (UN, 2024), 28 countries could not be included in this study (i.e. Albania, Andorra, Belarus, Bosnia and Herzegovina, Cyprus, Denmark, Estonia, Georgia, Greece, Hungary, Iceland, Latvia, Liechtenstein, Lithuania, Luxembourg, Malta, Moldova, Monaco, Montenegro, North Macedonia, Romania, Russia, San Marino, Serbia, Slovakia, Slovenia, Türkiye, Ukraine, and Vatican City). Data collection was conducted extensively across Europe over several months by a working group from the ClimXtreme project (https://www.climxtreme.net/index.php/en/, last access: 30 October 2024), with additional experts beyond the project contributing their expertise.
Table 1The four climate zones and 16 countries associated with the zones in this study. The countries of the Alpine zone were also assigned to other zones.
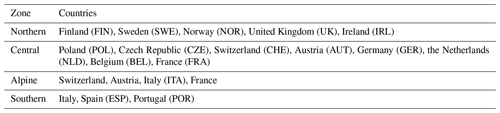
Physiological stress indicators, specifically crown defoliation data segregated into conifers and broadleaves, were sourced from ICP Forests Technical Reports (http://icp-forests.net/page/icp-forests-technical-report, last access: 30 October 2024). Insect pest data were gathered by analysing wood damage from reliable sources, including statistics, government reports, and scientific publications. Forest fire data were derived from the EC-JRC Technical Reports (https://forest-fire.emergency.copernicus.eu/reports-and-publications/annual-fire-reports, last access: 30 October 2024). To broaden our understanding, we incorporated tree cover loss (TCL) data from Global Forest Watch (https://www.globalforestwatch.org/, last access: 30 October 2024). Significant differences between the study period (2018–2022) and the reference period (2010–2014) were discerned utilising a t test conducted with RStudio 2022.12.0 (Table S1 in the Supplement). In the following sections, we take a closer look at the climatic situation during the 5 critical years, 2018–2022, in the four European zones (northern, central, Alpine, and southern).
2.1 Occurrence of drought and heat in Europe during 2018–2022
Persistent drought conditions characterised the spring and summer seasons during 2018–2022 across Europe, as shown by the Standardised Precipitation Evapotranspiration Index (SPEI; Vicente-Serrano et al., 2012, 2013; Beguería et al., 2013; Fig. 1). These prolonged droughts co-occurred with hot conditions across large parts of Europe (Xoplaki et al., 2023) and were linked to strong atmospheric blocking conditions over Europe characterised by persistent high-pressure anticyclonic systems. A persistent positive North Atlantic Oscillation (NAO) was found before the heatwave (Drouard et al., 2019; Li et al., 2020). This pattern was further associated with a double jet stream configuration and two high-speed wind currents in the upper atmosphere that influenced the intensity and persistence of atmospheric conditions in the inter-jet region (Rousi et al., 2023).
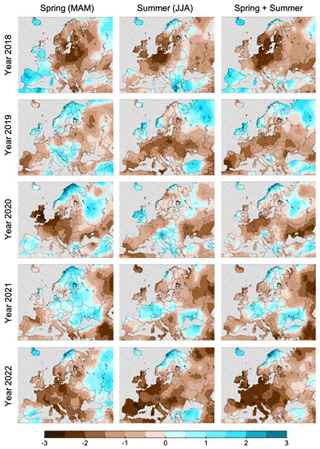
Figure 1SPEI (Standardised Precipitation Evapotranspiration Index) for spring (MAM – March to May), summer (JJA – June to August) and the entire growing season (March to August) during the 2018 (top row) to 2022 (bottom row) period. SPEI results are shown in units of standard deviation from the long-term mean of the standardised distribution. The window length for MAM and JJA is 3 months, and it is 6 months for spring and summer.
Furthermore, sea surface temperature anomalies exhibited a tripolar pattern in the North Atlantic, which has previously been identified as a precursor for European heatwaves (Beobide-Arsuaga et al., 2023) such as the one in 2015 (Duchez et al., 2016), as well as being a precursor for increased drought risk in central Europe via changes in the large-scale atmospheric circulation (Haarsma et al., 2015; Rousi et al., 2021; Ionita et al., 2022).
Hari et al. (2020) used pattern climatology data for Europe and long-term observations to claim that the consecutive droughts in 2018 and 2019 were unprecedented in the last 250 years. Adding the year 2020 to the analysis, Rakovec et al. (2022) found that the 2018–2020 drought was not only unprecedented in intensity, but also what made it truly exceptional was its average near-surface air temperature anomaly of +2.8 °C above the pre-industrial period. The authors identified the 2018–2020 drought event as having an unprecedented intensity that persisted for more than 2 years, exhibiting a mean aerial coverage of 35.6 % of Europe. Following the 2018–2020 extreme drought years, 2021 was a rather normal to wet year. However, persistent hot and dry conditions prevailed during spring and summer 2022, which led to depleted soil water levels (similar to 2018) and regionally critical drought conditions (Fig. 1). Throughout the summer of 2022, heat waves and exceptionally low rainfall led to very dry conditions in central Europe. Observed runoff anomalies highlighted the 2022 European drought as potentially the worst in 500 years (Henley, 2022; Schumacher et al., 2024). Between May and July 2022, many areas in Europe experienced the strongest 500 hPa geopotential height anomalies since 1950 (Toreti et al., 2022a).
2.2 Drought and heat in the northern zone, 2018–2022
From 2018 to 2022, Finland experienced a series of unusually warm and dry years. In 2018, prolonged heat and record-low rainfall caused significant groundwater depletion, algal blooms, fish and mussel deaths, and a 20 % crop yield reduction (Veijalainen et al., 2019; Winland-project Policy Brief VII, 2019). Groundwater levels remained low in 2019, with central and eastern Finland experiencing the worst dryness since 1955 (Ilmastokatsaus, 2019). The year 2020 saw record-breaking warmth and rainfall, particularly in southern and central Finland (Ilmastokatsaus, 2020). June and July of 2021 were exceptionally hot and dry (Ilmastokatsaus, 2021). In 2022, summer temperatures were nearly 2 °C above normal, with varying rainfall patterns across the country (Ilmastokatsaus, 2022).
Sweden experienced dry years in 2016 and 2017, particularly in southern Sweden where streamflow was 28 % below normal, prompting local water use restrictions (Geological Survey of Sweden, 2017). This drought persisted and peaked in 2018, leading to the most-severe wildfires in modern Swedish history (Swedish Board of Agriculture, 2019; Teutschbein et al., 2022a, b). Current climate conditions made such fires approximately 10 % more likely compared to pre-industrial times (Krikken et al., 2021). Drought conditions eased in subsequent years, with slightly drier conditions returning in 2022 (SMHI, 2023).
Norway experienced significant droughts from 2018 to 2022. In spring and summer 2018, temperatures were up to 4.7 °C above normal, and precipitation from May to September was only 18 %–46 % of the 1991–2020 average (Norwegian Center for Climate Services, 2023). This year had the longest drought period in 5 years of study. The years 2021 and 2022 were also dry, with 83 % and 84 % of average annual precipitation, respectively, and August 2021 being the driest month (Norwegian Center for Climate Services, 2023). Groundwater (GW) levels below the treeline dropped to 75 % of average in southeastern Norway in August 2018 and 2022, impacting agricultural production (NVE, 2023). As for the future, climate models predict more-intense precipitation and less snowmelt. Accordingly, floods induced by rainfall will increase in terms of frequency and magnitude, while floods induced by snowmelt will decrease, potentially leading to drought in late spring and summer (Hanssen-Bauer et al., 2017).
In 2018, the United Kingdom (UK) experienced combined heatwaves and droughts (Holman et al., 2021). This extended into late 2018 and 2019 in some areas (Turner et al., 2021). From June 2019 to February 2020, humid conditions caused harmful floods (Sefton et al., 2021). The year 2020 saw a hot, dry spring followed by a wet summer (Kendon et al., 2021). In 2021, temperatures and rainfall were slightly below the long-term average (Kendon et al., 2022). In 2022, the UK recorded its first annual average temperature exceeding 10 °C, while total rainfall remained below average (Kendon et al., 2023). The areas of Coningsby and Lincolnshire recorded temperatures over 40 °C for the first time in UK history (Kendon et al., 2023).
2.3 Drought and heat in the central zone, 2018–2022
Due to its geographical location and unfavourable hydrological conditions, Poland has relatively few natural water resources compared to the rest of central Europe (Susza, 2023). Almost 40 % of Poland's arable and forested land is permanently threatened by drought (Polish Supreme Chamber of Control, 2021). Drought impacts Polish agriculture approximately every 5 years, but recently it has affected large areas nearly annually, including the years 2015, 2016, 2018, 2019, and 2020. In 2018, severe soil drought resulted in over 50 d of no plant-available water in regions like Wielkopolska and Kujawy (Wawrzoniak et al., 2019). Soil droughts have also been observed in extensive forested areas in recent years (Lech et al., 2021).
The 2018 severe drought centred over southwest Germany, the Benelux countries, and northeastern France. The 2019 drought shifted east, impacting eastern Germany and neighbouring countries. Although the 2019 drought was not exceptionally severe, consecutive drought years exacerbated the water deficits in Germany (soil moisture impacts; Xoplaki et al., 2023) and France. Gravity Recovery and Climate Experiment (GRACE) data indicated severe drought conditions in western Germany in autumn 2018, shifting to eastern Germany and Poland in summer 2019 (Boergens et al., 2020). With the exception of southern Germany, Germany and France experienced continued drought until late summer 2020. Summer 2021 saw heavy precipitation and a severe flooding event in central Europe (Mohr et al., 2023). In 2022, extreme drought conditions affected Germany and France due to low precipitation amounts and the occurrence of early heatwaves in May and June. Drought-affected areas in Germany reached 40 % of the country in 2022, followed by 30 % in 2019, 19 % in 2018, and 16 % in 2020.
2.4 Drought and heat in the Alpine zone, 2018–2022
In 2018, Switzerland experienced the fourth-warmest spring and third-warmest summer since instrumental measurements began in 1864 (Bader et al., 2019). Summer 2018 received only 70 % of the long-term mean precipitation (1981–2010), although above-normal winter rainfall helped mitigate the summer's worst impacts. From 2019 to 2021, frequent summer heat episodes occurred with normal winter precipitation. However, winter 2021–2022 saw anomalously warm and dry conditions, especially in southern Switzerland and northern Italy. Summer 2022 had record-breaking temperatures, with July being one of the hottest months since 1864, and low rainfall led to record-low levels in many lakes in eastern and central Switzerland.
Austria, despite its generally water-rich Alpine areas, faced exceptional heat and drought in 2018 and 2022, raising concerns about water availability (Stelzl et al., 2021). A significant decline in snow depth in the Alpine region that may have balanced the increased summer evaporative demand exacerbated these impacts (Matiu et al., 2021). The summer of 2019 was less dry but broke heat records (Olefs et al., 2021). Summer 2022, the fourth-warmest on record, followed a dry and mild winter, and while rainfall events occurred, they were too heavy to alleviate drought conditions due to the high runoff (GeoSphere Austria, 2024).
2.5 Drought and heat in the southern zone, 2018–2022
Italy experienced less impact from the 2018 drought compared to central and northern Europe, with no significant soil moisture anomalies or forest disturbances reported (Senf and Seidl, 2021a).
However, drought conditions persisted into the summers of 2021 and 2022 (Toreti et al., 2022a), exacerbated by a winter rainfall deficit (Toreti et al., 2022b; Bonaldo et al., 2023). The year 2022 was particularly extreme, characterised by 9 consecutive months almost without precipitation, leading to the drying out of the Po River (Montanari et al., 2023). The winter of 2022–2023 remained relatively dry (Toreti et al., 2023).
In Spain, precipitation between 2020 and 2021 was 5 % below normal. From October 2021 to early March 2022, accumulated rainfall was 38.2 % below average (BOE, 2022). In early March 2022, the peninsular water reserve was 40.5 %, markedly below the 5-year average of 52.5 % and the 10-year average of 60.8 %. The water reservoir system, designed to manage demand during dry periods using reserves from wetter years, has been strained by consecutive years of below-average precipitation since 2012–2013, except during 2017–2018. The 2021–2022 hydrological year was among the three driest on record, with precipitation 25 % below average and reservoir levels at 35 %, the lowest in 27 years (Greenpeace, 2022).
Over the past 20 years, mainland Portugal has experienced significant drought, with 6 of the 10 driest years occurring post-2000, including 2017–2018, 2019, and 2021–2022. The 2021–2022 hydrological year recorded 488.3 mm of precipitation; a deficit of 393.8 mm compared to the 1971–2000 average. It ranks as the third-driest year since 1944–1945, following 2004–2005 (APA, 2023). From 2018 to 2020, drought in Portugal was less severe, predominantly affecting the southern regions (Fig. 1). During 2019–2020, drought conditions were more pronounced, with 5 of 11 hydrographic basins showing negative monthly storage deviations throughout the year. The 2020–2021 year saw four basins with below-average storage levels (APA, 2023).
2.6 European droughts from past to future: an attribution challenge
A long-term drying trend has been observed in central and southern Europe in recent years, with climate simulations projecting these trends to continue (Stagge et al., 2017; Ukkola et al., 2020; Bakke et al., 2023). There is high confidence that temperature increases and precipitation decreases have already led to increased aridity in the Mediterranean region (IPCC, 2021a). According to the latest Intergovernmental Panel on Climate Change (IPCC) report (IPCC, 2021b), the combined warming and drying trend is attributable to human causes. This trend is less clear in western and central Europe, but there is high confidence in decreased aridity in response to increased precipitation in northern Europe (IPCC, 2021a). However, Christidis and Stott (2021) found increased drought risk in France and Germany based on summer SPEI trends between 1950 and 2018. Southeastern Europe is also affected, following an analysis based on rainfall and precipitation minus potential evapotranspiration (P-PET) reanalysis data (Christidis and Stott, 2021). Longer-term SPEI trends (1902–2020) indicate drying hotspots in Spain, Portugal, southern France, Italy, eastern Germany, the Czech Republic, Poland, Hungary, Slovenia, and Croatia (Ionita et al., 2021a). Changes in large-scale atmospheric circulation in the North Atlantic region may be linked to these drying conditions (Ionita et al., 2022) and possibly to the slowdown of the Atlantic Meridional Overturning Circulation (AMOC; Caesar et al., 2018). The extent to which these trends are attributable to anthropogenic impact remains a question.
Two approaches have been widely used to address this question: (i) the paleo-climatic perspective based on proxy data and (ii) longer-term climate model projections. Büntgen et al. (2021) found that recent drought extremes (2015–2018) are unprecedented over the past 2000 years in the Czech Republic and neighbouring regions, while Ionita et al. (2021b) suggest that mega-droughts during the 15th, the late-18th, and the early-19th centuries were longer and more severe in Europe. Despite differences in the methods and regions studied, these findings highlight the challenge of drawing definitive conclusions about current drought intensity in a historical context.
Climate model projections based on the latest CMIP6 assessment confirm historical trends observed in drought conditions. According to the IPCC (2021b), rainfall deficits are expected to be most pronounced during summers by the end of the 21st century in central and southern Europe. While increased winter and spring precipitation may offset some summer water deficits (i.e. a negative hydrological balance), this is unlikely for France, Germany, and the Mediterranean region. Trends in evapotranspiration, already negative annually, are projected to worsen in summer. Annual mean rainfall changes are not informative for drought attribution, as drought and heavy precipitation events can occur in the same season, creating adverse conditions for agriculture and forestry despite balanced mean rainfall.
Meteorological drought conditions are likely to become more frequent under current climate projections (Moemken et al., 2022). The current series of extreme drought years is likely a precursor to a new normal in Europe. These projections apply to transient warming conditions; if carbon emissions cease, the climate will transition to an equilibrium warming phase, reducing the land–ocean temperature contrast and potentially altering the drying trend (Byrne and O'Gorman, 2013; Dittus et al., 2024).
Attribution studies of individual extreme drought events are complex due to the low signal-to-noise ratio. While heat waves are easily attributed to anthropogenic climate change (Vogel et al., 2019; IPCC, 2021a), drought events remain challenging to attribute robustly. Van der Wiel et al. (2022) found that droughts of a similar magnitude to those from 2018 to 2020 are within the realm of current climate possibilities, with the signal emerging from natural variability over time, impacting biodiversity and human health.
As it is difficult to reconcile the existing lines of evidence, only a few drought attribution studies have tried to quantify the role of humans thus far. A prominent rapid-event attribution of the intense 2022 drought in central and western Europe showed that human-induced climate change made the root zone soil moisture drought about 3–4 times more likely and the surface soil moisture drought about 5–6 times more likely (Schumacher et al., 2022). The authors concluded that while the magnitudes of historical trends vary between different observation-based soil moisture products, they all agree that the dry conditions observed in 2022 would have been less likely to occur at the beginning of the 20th century. One study on the 2015 European summer drought concluded that the attribution results depend on the methodology used (Hauser et al., 2017). Human influence on the increased likelihood of central-European droughts could only be detected when using the largest-possible forcing difference in CMIP5 models. García-Herrera et al. (2019) analysed the drought that affected France and western Germany from July 2016 to June 2017, stating that recent trends, including those in human-induced higher temperature, have exacerbated the severity of the drought event. Finally, Philipp et al. (2020) investigated the hydrological drought of 2018, stating that the trend is driven by strong trends in temperature and global radiation rather than a trend in precipitation, resulting in an overall trend in potential evapotranspiration. Given that these trends match results from climate model simulations, the authors conclude that the observed trend in agricultural drought can at least in part be attributed to human-induced climate change.
Drought events compounded by heat waves can fundamentally transform the composition, structure, and biogeography of forested ecosystems (Allen et al., 2010, 2015). Overall, the consequences for forests can be summarised in three major impact categories: (i) physiological stress, (ii) insect outbreaks, and (iii) forest fires (e.g. Brodribb et al., 2020; Seidl et al., 2020; Mezei et al., 2022; Salomón et al., 2022). From 1950 to 2019, the number of natural disturbances observed in European forests has increased, with wind being the most important factor (46 % of total damage), followed by fire (24 %) and bark beetles (17 %), although the latter's contribution to the total damage has doubled in the last 20 years (Patacca et al., 2023).
One of the primary impacts of heat and drought on forests is increased tree mortality (Allen et al., 2010; Anderegg et al., 2013; George et al., 2022). Trees can be highly sensitive to drought stress and prolonged periods of high temperatures, and together with low precipitation, these conditions can cause trees to experience water deficits, leading to physiological stress and ultimately death. In general, trees under drought and heat stress may experience carbon starvation and face greater risks of embolism, which can cause a failure in the water transport (Allen et al., 2015; Schuldt et al., 2016). Such physiological stress can lead to mortality but also to milder consequences such as crown defoliation, early leaf shedding, or death of branches, which reduce the vitality and growth of the trees (Schuldt et al., 2016). Soil drying may lead to water repellency (soil hydrophobicity), which slows down the infiltration of rainwater following the end of the drought and produces a heterogeneous soil wetting front (Grünzweig et al., 2022). Soil hydrophobicity has been observed in various temperate forests and diverse soil types in Europe, which may increase drought stress and tree die-off (Gazol et al., 2018; Gimbel et al., 2016; Hewelke et al., 2018; Seaton et al., 2019). As a consequence, reduced forest cover can exert a negative (buffering) feedback on climate change impacts by decreasing the aerodynamic resistance of heat transfer from trees to the surrounding air. The reduced resistance increases sensible heat flux, decreases forest temperature, and enhances water savings because of a reduced need for cooling by transpiration (Rotenberg and Yakir, 2010; Banerjee et al., 2017). For example, during the 2003 extreme heatwave in central and western Europe, surface temperatures rose less in forests than in non-forested areas, allowing forests to conserve water (Teuling et al., 2010). This “canopy convector effect” is an adaptation mechanism, which can prevent long-term amplification of the consequences of extreme heat and drought (Grünzweig et al., 2022). A quantitative understanding of regional and local biophysical effects of such land use changes is required to enable effective land-based mitigation and adaptation measures (e.g. Perugini et al., 2017). However, these effects are complex and strongly depend on local conditions, making their quantification challenging.
At the same time, other processes like outbreaks of forest pests can co-occur with and follow droughts. Resin release plays a pivotal role in the resistance of conifers to bark beetles (Morcillo et al., 2019). However, resin production is very costly in terms of available resources and strongly linked to tree vigour and water availability (Zas et al., 2020). But drought-induced host weakening does not determine beetle outbreaks alone. Dry and warm conditions generally also increase the vitality and reproduction of polythermic insects with consequent shorter generation times, higher fecundity, and survival rates (Jactel et al., 2019; Pettit et al., 2020). It should be noted that heat waves can also negatively affect some insect pest species or pathogens, given their response to the heat stress (Sire et al., 2022).
Heat and drought can create favourable conditions for wildfires to start and spread (Kirchmeier-Young et al., 2019), and drought-stressed trees are more susceptible to ignition and can burn more readily. Although wildfires have decreased on a global scale and across Europe over the last decade (2010–2020), there have also been years with the highest level of fire damage ever recorded in Europe in the past decade (Grünig et al., 2023; Patacca et al., 2023). Several regions (central Europe among others) are likely to face larger and more-frequent wildfires in the future (Feurdean et al., 2020; Milanovic et al., 2021). A study investigating storm and fire disturbances in Europe from 1986 to 2016 identifies storms and fires as the most important abiotic disturbances in the recent past, with wind (i.e. storms) mainly dominating in central and western Europe and fire in the southern part of the continent (Senf and Seidl, 2021b). While in 2018 fire was likely only responsible for about 3 % of the area disturbed in northern and central Europe (Senf and Seidl, 2021a), there is strong evidence that wildfires will increase in a warmer and drier environment (Seidl et al., 2017). This increase can facilitate deforestation, loss of habitat, soil erosion, and long-term changes in forest structure and composition that can have severe environmental, economic and social consequences (Leverkus et al., 2019). Wildfires commonly lead to hydrophobic soils (Davies et al., 2013; Mao et al., 2019), thus reducing water infiltration and causing further damage to trees (Grünzweig et al., 2022).
The forest damage caused by drought leads to significant socioeconomic consequences in European forest ecosystems (Lindner et al., 2010) as forest owners, logging companies, and other stakeholders in the forestry sector experience significant losses due to a reduction in volume and quality of timber (e.g. Brecka et al., 2018; Davies et al., 2020; Knoke et al., 2021). Further impacts to local economies and communities can occur, since the forestry sector is an important employer in many rural areas of Europe, employing about 3.6 million people (EU-27; EUROSTAT, 2023). Furthermore, the value of forest areas is likely to decrease if economically valuable tree species decline (Hanewinkel et al., 2013), and the cultural and recreational qualities of forests can suffer (Winkel et al., 2022).
Heat and drought can also disrupt forest ecosystem dynamics and alter community composition (Hicks et al., 2018), as tree species differ in their vulnerability to drought stress, leading to shifts in species abundance and distribution (Morin et al., 2018). These changes can also have cascading effects on other organisms that depend on forest ecosystems, such as mammals, birds, reptiles, amphibians, or invertebrates (Liebhold et al., 2017), and drought can disrupt these complex ecosystems (Krumm et al., 2020; Vicente-Serrano et al., 2020). Reduced water availability can also strongly affect the carbon cycle by limiting photosynthesis and nutrient uptake and can lead to decreased growth rates and reduced carbon storage in forests. Many recent publications discuss the impact of drought and heat on forest carbon balances, a critical aspect that could not be specifically addressed within this study; relevant information can be found, e.g. in Peters et al. (2020).
The projected increase in frequency and intensity of heat and drought events (Spinoni et al., 2018) will likely increase forest damage. The drought in 2018 alone was probably the largest source of severe forest disturbances in Europe in over 170 years (Senf and Seidl, 2021a). Forest disturbances increased significantly across much of Europe in 2018, particularly in the central and eastern regions, and remained above average in both 2019 and 2020 (Senf and Seidl, 2021a). However, there are opportunities to better understand the damage and to mitigate future harm.
3.1 Europe-wide damage to forests, 2018–2022
The central zone exhibited the most significant impacts and damage between 2018 and 2022, showing high or very high significance for crown thinning, pest outbreaks, and TCL compared to the reference period (Fig. 2). The southern zone, while still affected, had less pronounced damage extents, although significant differences in crown thinning and TCL were observed. The northern zone experienced highly significant TCL. In the Alpine zone, no statistically significant differences were detected. Additionally, across all zones, there were no significant differences in damage caused by wildfires.
A pairwise t test comparing the averages examined whether the observed changes (difference in means) between the two periods (2010–2014 and 2018–2022) are statistically significant. The test results indicate that the difference between the two periods was not statistically significant (p=0.06) for defoliation of both conifers and broadleaves, with a mean difference of 9.4 %. The t-test results for forest fire occurrence clearly showed no significant difference (p=0.34; mean difference – 3400.9 ha). However, for TCL, the mean difference of 0.34 % was highly significant (p=0.004). A similar statistical test for wood damaged by insects was not feasible due to insufficient data.
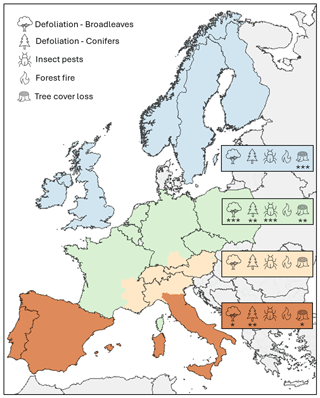
Figure 2Differences in impacts and damage (crown defoliation [%] of broadleaves and conifers, wood damaged by insects [1000 m3], burnt forest area [ha], and tree cover loss [%]) between the study period (2018–2022) and the reference period (2010–2014). * is significant (p<0.05), is highly significant (p<0.01), and is very highly significant (p<0.001). Map created with http://www.mapchart.net (last access: 30 October 2024).
Significant differences were observed across the 16 countries (Fig. 3). In the central zone, coniferous trees showed highly significant crown thinning in the Czech Republic, Belgium, and France, while significant effects were observed in Germany (see Fig. 4 for more detailed results). For deciduous trees, France exhibited highly significant crown thinning, while Germany and the Czech Republic showed significant effects. In the southern zone, deciduous trees in Italy were highly significantly affected, and in Spain conifers were highly significantly and broadleaves significantly affected. In the northern zone, no increased crown thinning was observed during the drought period in individual countries. However, a particularly high incidence of wood damage due to pest infestation was found in the central zone, which was highly significant in the Czech Republic, Switzerland, Austria, and Germany (see Fig. 5 for detailed results). An increase in wildfires during the drought period was only observed in Norway and Germany, where the affected area was significantly higher during 2018–2022 (see Fig. 6 for detailed results). TCL was most significant in Finland, Sweden, and Norway, with Ireland also showing highly significant impacts (see Fig. 7 for detailed results). In the central zone, Poland, the Czech Republic, Switzerland, and Austria were highly significantly affected, with Germany showing significant effects. In the southern zone, Italy and Spain were significantly affected by TCL. No significant differences in impacts were found for the United Kingdom, the Netherlands, and Portugal.
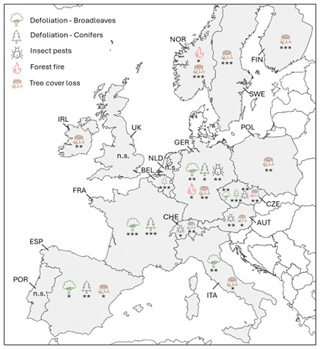
Figure 3Significant differences in the 16 countries regarding impacts and damage (crown defoliation [%] of broadleaves and conifers, wood damaged by insects [1000 m3], burnt forest area [ha], and tree cover loss [%]) between the study period (2018–2022) and a reference period (2010–2014). n.s. is not significant, * is significant (p<0.05), is highly significant (p<0.01), and is very highly significant (p<0.001). Map created with https://www.mapchart.net/ (last access: 30 October 2024).
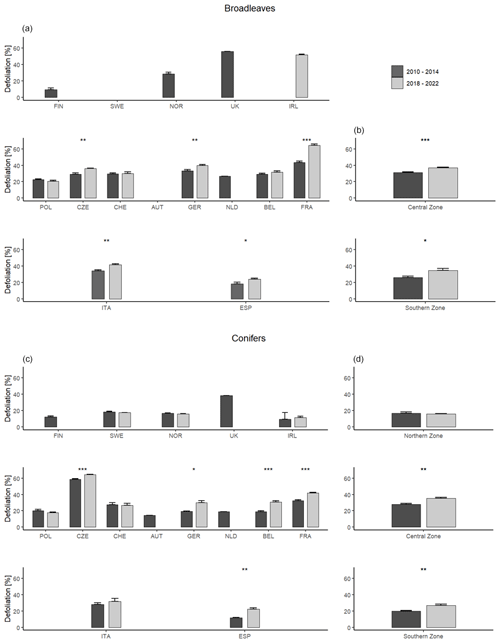
Figure 4Relative crown defoliation of broadleaves (a, b) and conifers (c, d) during the dry period, 2018–2022, and the reference period, 2010–2014 (>25 % needle or leaf loss, i.e. moderate to severe defoliation). Data come from ICP-forests (2022). For broadleaves in the northern zone, sufficient data were not available.
Wood damage caused by insect infestation was significantly higher across central Europe in the study period of 2018–2022 than in the reference period (Fig. 5). Notable is the situation in the Czech Republic, where insect-induced wood damage even surpassed the mean annual roundwood production (2010–2014). Sweden also experienced a degree of roundwood damage attributable to insects during the assessed drought period. While data on roundwood damaged by insects were accessible for select countries, they were not uniformly available across all regions. Notably, acquiring such data was comparatively easier during the more-recent period, indicative of heightened pressures exerted by insect pests within forest ecosystems and a greater interest in monitoring forest damage.
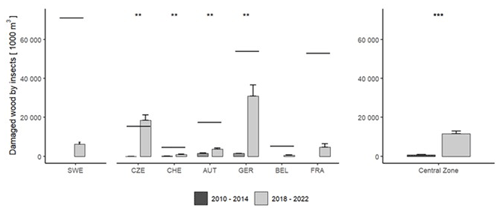
Figure 5Roundwood damaged (1000 m3) by insect pests in Europe in the period of 2018–2022, partly in comparison with the reference period of 2010–2014. The black lines show the total roundwood production average per year during 2010–2014. Wood data were derived from different sources (EUROSTAT, 2016; Wulff and Roberge, 2020; Öhrn et al., 2021; EUWID, 2022; Michel et al., 2022; DESTATIS, 2020, 2023; Waldschutz, 2023; WSL, 2023b; BFW, 2020, 2023, Czech Statistical Office upon request). For the other countries, no data were available.
In our analysis of forest fire occurrences, we did not find significant differences between the dry period in 2018–2022 and the reference period of 2010–2014, except for Norway and Germany (Fig. 6). This lack of significant differences was consistent across the northern, central, Alpine, and southern zones. Generally, countries in the southern zone experienced severe impacts from forest fires. For example, the damage in Sweden and France, which had the highest amounts of burned area in their climatic zones (5000 and 10 000 ha, respectively) during the period of 2018–2022, was only a fraction of that observed in Portugal during 2017.
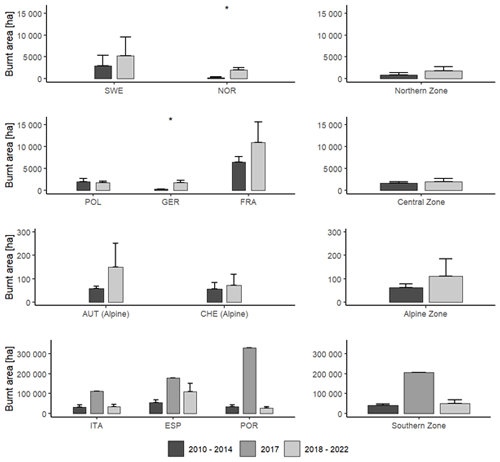
Figure 6Burnt forested area (the mean for the two periods under consideration) in selected European countries. Italy and Portugal had large fires in 2017 (accordingly, the value for 2017 is given for the southern zone). All data are from the JRC Technical Reports of Forest Fires in Europe, the Middle East, and North Africa from the years 2010 to 2022 (https://forest-fire.emergency.copernicus.eu/reports-and-publications/annual-fire-reports, last access: 30 October 2024). The data utilised here stem from the EC-JRC national reports from the years 2010 to 2022, where areas are designated as forested regions. Absolute values were employed instead of relative values due to inconsistent forest area data across all countries within the dataset. Please note the different scales.
The loss of tree cover can stem from various human and natural factors, such as forestry activities (e.g. logging or deforestation), natural occurrences (e.g. diseases or storms), and fire incidents. Notably, very highly significant disparities between the dry period (2018–2022) and the reference period (2010–2014) were observed in the northern zone (Fig. 7).
3.2 Damage to forests in the northern zone, 2018–2022
The total forested area in Finland is 26×106 ha (EFFIS: 24.1×106 ha), of which 20×106 ha is suitable for forest production. Forest damage in Finland was highest in 2018 (21 700 ha) and has been decreasing since then, followed by an increase in 2022 (damage levels in 2019 – 15 800 ha; 2020 – 14 000 ha; 2021 – 12 000 ha; and 2022 – 19 100 ha; Nuorteva, 2019; Nuorteva et al., 2022; Melin et al., 2022; Terhonen et al., 2023). These numbers are high for Finland given that the accumulated forest drought damage in the years 2009–2015 was 8700 ha (Nevalainen and Pouttu, 2017).
The areas influenced by drought and bark beetles were localised and, on an annual scale, quite small when compared, for example, to snow and moose-based damage (Nuorteva, 2019; Nuorteva et al., 2022; Melin et al., 2022; Terhonen et al., 2023). In Finland, the bark beetle population grew slightly between 2018 and 2020, and the damage increased from 12 600 to 21 400 ha but declined slightly to 20 800 ha and 18 000 ha in 2021 and 2022, respectively. In 2021, the bark beetle damage was slightly lower than in 2020, but in 2022 the Finnish Forest Centre received reports of more damage than usual from more-northern areas (South Karelia and North Savo). The reported salvage logging due to insect outbreaks was 3400 ha by November 2022, which is 3 times higher than in 2021 (Metsäkeskus, 2022). Overall, beetle damage in Finland has been increasing during the last decade, and, in the future, the risk of more-intense damage is rising (Neuvonen, 2020). It should be mentioned that the storm damage to forests in Finland has also increased from 2018 to 2022: from 249 000 to 276 300 ha (highest in 2021 at 307 100 ha). Forest damage could be influenced by the overall well-being of the trees. Additionally, the efficiency at collecting the fallen trees influences the bark beetle spread and outbreaks, since deadwood provides prime habitat for the beetle population to grow (Hroššo et al., 2020).
The number of forest fires in Finland in 2018 was the second-highest recorded, but only approximately 1200 ha of forest was damaged (Lehtonen and Venäläinen, 2020). In 2019 the area damaged by forest fires was roughly 500 ha, in 2020–2021 slightly over 1000 ha, and in 2022 only slightly over 265 ha (Aalto and Venäläinen, 2021; Melin et al., 2022; Terhonen et al., 2023). Kosenius et al. (2014) estimated the financial losses due to forest fires in North Karelia and the Republic of Karelia for the years 2009 to 2012. They considered the direct and indirect costs when preparing estimates for the total costs. Venäläinen et al. (2016) used the estimates made by Kosenius et al. (2014) to derive a median estimate for forest fire costs in Finland: EUR 6660 per hectare (estimates ranged from EUR 5381 per hectare in 2009 to EUR 8810 per hectare in 2012). Using the Swedish forest fire cost estimates of Venäläinen et al. (2016) for Finland, between 2018 and 2021 these fires caused roughly EUR 25 million in total damage.
In Sweden, about 90×106 m3 of trees is felled every year (UNECE, 2022), and the total forested area is 27.9×106 ha (SCB, 2020). Physiological damage, expressed as crown defoliation, ranged between 17.1 % and 17.8 % for conifers during 2018–2021 (data for 2022 and for broadleaved trees were unavailable; Michel et al., 2022). In Sweden, bark beetles damaged 3×106–4×106 m3 of spruce in 2018, 7×106 m3 in 2019, and 8×106 m3 in 2020 and 2021, more than 20 times the average of the previous years (Wulff and Roberge 2020; Öhrn et al., 2021; UNECE, 2022). This increase in mortality and damage was caused by the heat and drought in 2018, which allowed for rapid beetle population growth (Öhrn et al., 2021). In Sweden, the dry and warm period of summer 2018 led to the most-severe outbreak of forest fires, estimated at around 25 000 ha and with 3×106 m3 of wood damaged (NordicWoodJournal, 2018). Using the estimate of Venäläinen et al. (2016), the costs for the year 2018 are more than EUR 166 million. This is a similar estimate as though the forest fires in Sweden in 2014 (14 000 ha; costs – SEK 1 billion) were upscaled to 2018 – EUR 160–200 million.
Norway has a total forested area of more than 12×106 ha, of which 8.6×106 ha is suitable for forest production (SSB, 2022). On a national level, the drought did not have a serious impact on Norwegian forestry. In 2017, there was a total of 96.5×106 m3 of standing forest, which increased to 987×106 m3 in 2020 (SSB, 2022). Physiological damage, expressed as moderate-to-severe crown defoliation, ranged from 14.9 % to 17.2 % for conifers in 2018 to 2021 (data for 2022 and for broadleaved trees were not available; Michel et al., 2022).
Norway's annual roundwood production is about 11×106 m3 (ICP, 2022). Numbers from NIBIO's forest portal Kilden (NIBIO, 2023) show an increase in bark beetles in the region, from 8540 per trap in 2017 to 20 600 in 2021, and although concerning, these levels remain below outbreak levels.
The forest area affected by fires in Norway was more than 3000 ha in 2018 and 2019 and decreased to less than 400 ha in 2019 and 2020 (NIBIO, 2023). A record number of 1906 forest fires occurred between January and August 2018. Wells and drinking water resources were almost empty, low water levels in rivers led to fish dying, and electricity production was at times 20 % below normal production levels (−23 TW h), leading to higher electricity costs (MET Norway, 2019). Favourable wind conditions meant that the total area affected was relatively small (2000 ha affected by forest fires), so the consequences were more related to costs and social uncertainty. The Norwegian Directorate for Civil Protection – DSB (2019) estimates that about EUR 8.4 billion (NOK 100 million) was spent on fighting the forest fires; while indirect costs are unknown, they are expected to be high (loss of infrastructure, houses, and cabins). Reports from the county governor of Vestfold and Telemark (Statsforvalteren, 2020, 2021) show some of the consequences for the forests in the region. Vestfold and Telemark county has 6.5×106 ha of productive forest and annual growth of 2.75×106 m3 in timber volume. Damage from forest fires has led to an increase in tree felling: 1.1×106 m3 in 2018, 1.23×106 m3 in 2019, and 1.1×106 m3 in 2020, despite low timber prices, especially in 2020. In comparison, the average felling in the 2010–2014 reference period was 896 000 m3 yr−1. To mitigate the consequences of the 2018 fires, 296 599 saplings were planted in 2019, and another 250 000 in 2020 compared to an average planting of 131 000 in the reference period. While there have been some short-term effects, the drought in Norway has not yet had a lasting negative impact. However, there are indications of increased beetle attacks and more deadwood because of periods with heavy snow in winter, and forest authorities are concerned about the future (e.g. Gunther, 2019; Wataha, 2021).
In the UK, woodlands cover approximately 3.24×106 ha, with an almost equal distribution between conifers (1.65×106 ha) and broadleaves (1.59×106 ha) (Forest Research, 2022a, b). Drought events have significantly impacted these woodlands in recent years. In 2018, early leaf senescence was observed in southern regions due to drought (Michel et al., 2019). Although 2019 was wetter and milder, thus mitigating severe drought impacts (Michel et al., 2020), challenges persisted in subsequent years. The 2020 weather extremes exacerbated ash dieback (caused by Hymenoscyphus fraxineus), with widespread future mortality expected (Michel et al., 2021). By 2021, Phytophthora pluvialis was affecting mature trees, and 2022's severe drought led to widespread defoliation (Michel et al., 2022; Forest Research, 2022c).
Wildfire activity has varied, with significant events in 2018 (17 689 ha burned), 2019 (28 754 ha), 2020 (13 793 ha), and 2022 (20 362 ha). The average annual burned area from 2011 to 2022 was around 10 000 ha (EFFIS Annual Statistics for UK, 2023). In England, woodlands span 1.32×106 ha, of which 26 % constitutes conifers and 74 % constitutes broadleaves (Forest Research, 2022a, b). Notably, 2018 saw severe wildfires in Greater Manchester, which burned 3600 ha and predominantly affected broadleaved woodlands (Telegraph, 2018; BBC, 2018). In 2022, the English fire services managed nearly 25 000 wildfires (BBC, 2022). Wales, with 310 000 ha of woodland equally divided between conifers and broadleaves, faces frequent fires, especially in the south. In the spring of 2020, fires in the Afan Valley and Seven Sisters forests caused over EUR 115 000 in damage and destroyed nearly 140 ha (NRW, 2020).
Scotland has 1.49×106 ha of forest, 74 % of which constitutes conifers and 26 % broadleaves. The region's forests, including Sitka spruce plantations, are particularly vulnerable to drought (Kirkpatrick et al., 2021). Although no insect damage data were available, the great spruce bark beetle (Dendroctonus micans) is now established in southern Scotland (Scottish Forestry, 2023a) and has expanded northwards from 2018 to 2022 (Scottish Forestry, 2023b). Sitka spruce is Scotland's most important commercial tree species, and it is the primary host of this pest. The 2018 drought hindered forest regeneration, while wildfires in April 2018 and 2019 affected significant areas (Copernicus, 2023; The Herald, 2021). In March and April 2022, 95 wildfires were recorded (Highland Council, 2023). Key industries such as whisky production and forestry are heavily reliant on a stable water supply (Kirkpatrick et al., 2021).
Northern Ireland, with 118 000 ha of woodland (54 % conifers, 46 % broadleaves), experienced wildfires in spring 2022, damaging approximately 720 ha (DAERA, 2022). Ireland's forest area ranges from 551 110 ha (EFFIS, 2023) to 770 020 ha (Forest Statistics Ireland, 2020), with a predominance of conifers (three-quarters, including 51 % Sitka spruce) and broadleaves (one-quarter). Forest health remains generally good, with high defoliation rates reported only in 2020 and 2021 (Michel et al., 2022). Ireland's strict pest regulations and island status protect against many forest pests (O'Hanlon et al., 2021). Approximately 3000 ha of forest burned annually from 2018 to 2022, which is moderate compared to record years (EFFIS Annual Statistics for Ireland, 2023).
3.3 Damage to forests in the central zone, 2018–2022
Poland has a forest area of 9 242 000 ha (Central Statistical Office, 2017). In 2018, the drought significantly weakened the condition of the forests over an area of 43 500 ha. In the same year, forest damage was observed on 29 400 ha (Jabłoński et al., 2019a, b). In 2019, the order of species from healthiest to most damaged was determined based on the analysis of three parameters: average defoliation, the proportion of healthy trees (up to 10 % defoliation), and the proportion of damaged trees (more than 25 % defoliation). The order is as follows: Fagus sylvatica, Alnus spp. < Abies < other deciduous, other coniferous < Pinus sylvestris < Betula spp. < Picea abies < Quercus spp. (Wawrzoniak, 2019). In 2020, symptoms of weakened or damaged forest stands caused by disruption of water relations mainly due to drought were reported in 253 out of 430 (i.e. 59 %) forest districts (Lech, 2021).
Pests, which until a few years ago were considered of little concern in Polish forests, today cause the death of many hectares (Sierota et al., 2019). As a result of the drought in the years 2015–2019, secondary factors leading to the death of pine stands (which represent 58.2 % of the Polish forests), have become more active (Sierota et al., 2019). The key role was played by the following pests: the bark beetle (Ips acuminatus), mistletoe (Viscum spec.), Sphaeropsis blight (Sphaeropsis sapinea), the steelblue jewel beetle (Phaenops cyanea), Heterobasidion root disease, and Armillaria spp. (Sierota and Grodzki, 2020). Observations in Poland indicate a significant correlation between drought and engraver beetle (Ips acumintus) outbreaks (Jabłoński et al., 2019a, b; Plewa and Mokrzycki, 2017), a species that until not long ago was not considered a significant forest pest (Głowacka, 2013). The occurrence of mistletoe was also underestimated (Viscum sp.). After prolonged drought periods, the area of the coniferous (mostly pine) forests heavily infested by mistletoe has drastically increased, from 1400 ha in 2017 to almost 23 000 ha in 2018 (Jabłoński et al., 2019a). Mistletoe was found on 14 forest tree species: the most severely infested were fir and pine trees and to a lesser extent birch, as well as a mixture of deciduous species and spruce (Lech et al., 2019). In addition, well-known forest pests such as the European spruce bark beetle (Ips typographus) continue to pose a major threat to Polish forests. The dieback of Norway spruce stands was already increasing in central and eastern Europe in the 1970s and 1980s (Sierota et al., 2019). After the drought in 2015, the Norway spruce decline continues, with new bark beetle outbreaks affecting stands in the Western Carpathian and Sudeten mountains. The ongoing climatic conditions, combined with high bark beetle populations, make the risk of a further outbreak extremely high (Grodzki, 2010).
Recent years have seen significant surface losses on Poland's state forest land due to drought and high temperatures (DGLP; Dyrekcja Generalna Lasów Państwowych). Drought-related losses were 40 852 ha (2018), 60 356 ha (2019), 58 056 ha (2020), 34 673 ha (2021), and 20 258 ha (2022). High temperature losses (burns, wilt, dieback) were reported affecting 80 ha (2018), 340 ha (2019), 2574 ha (2020), 197 ha (2021), and 244 ha (2022). Long-lasting drought in Poland has also led to a lowering of the surface and GW table, as well as to a decrease in tree growth, stand vitality, and resistance to pathogens and pests (Kwiatkowski et al., 2020). Among the species affected by this process are oaks, where the impact of declining GW has been observed since the late 1980s (Przybył, 1989). Current GW fluctuations further weaken oak trees and accelerate their decline (Skrzecz et al., 2022), e.g. on the Krotoszyn Plateau (Danielewicz, 2016).
Furthermore, the prolonged drought has increased the risk of forest fires. Despite the high number of fires, the situation in Poland is relatively good. The average forest fire in the state forests is only 0.25 ha, which indicates a high efficiency of fire protection systems. According to official statistics, between 2011 and 2020, almost 25 000 fires with a total area of 6049 ha occurred in the areas managed by the state forests, causing losses of approximately PLN 39 million. However, the year 2020 was marked by a large fire (6000 ha) in the Biebrza National Park in northeastern Poland.
In the Czech Republic, forest disturbances, mainly by pests, were triggered by drought and high temperature. Near Kostelec and Černými Lesy, studies found that bark beetle outbreaks were related to the duration of April solar radiation in the previous year and the mean in annual air temperature in the current year (Pirtskhalava-Karpova et al., 2024). In the Bohemian Forest, researchers observed that the surface temperature in stands subsequently attacked was higher in the year preceding pest colonisation when compared to intact stands (Kozhoridze et al., 2023). At the beginning of the massive bark beetle attacks, spruce accounted for 50.5 % of stands and pine for 16.4 % (Zahradník and Zahradníková, 2019). This abundance of bark-beetle-sensitive trees led to the suggestion that the Czech Republic may have been the epicentre of bark beetle outbreaks in Europe (Hlásny et al., 2021) since more than 50 % of Czech forests were seriously threatened by this pest, resulting in high ecological and economic losses (Fernandez-Carrillo et al., 2020). The commonly harvested volume of wood per year is about 15×106 m3, of which around 1×106 m3 is designated wood infected by insects (WII). In 2018, 25.6×106 m3 was harvested and 13×106 m3 was WII; in 2019, 32.5×106 m3 was harvested with 22.8×106 m3 of WII; and in 2020, the estimate ranges between 40×106 and 60×106 m3 of WII (Fernandez-Carrillo et al., 2020). The timber damage was almost exclusively caused by European spruce bark beetle infestations (Ips typographus L.). The largest forest fire in Czech history broke out in the Bohemian Switzerland area in the northern Czech Republic and spread to Germany. The fire affected an area of about 1060 ha; over 1000 firefighters, 5 helicopters, and 2 firefighting aircraft were needed to get the fire under control (Worlds Aid, 2022). On the German side of the border, an area of about 150 ha in the Saxon Switzerland National Park was affected (DAV, 2022). During the decade of 2010–2020 in the Czech Republic, almost 100×106 m3 of solid timber was harvested and linked to bark beetle attacks, which led to financial losses in the Czech forestry sector of ca. EUR 1.12 billion (Toth et al., 2020). More than half of this volume has been extracted since 2017, and this amount of unplanned salvage logging represents an increase of about 3-fold from 2017 to 2018 (Moravec et al., 2021). There were also clear signs of loss of vitality during the dry period (2015–2019), with growth reductions in five major species due to drought conditions that were observed when compared to the reference period of 2005–2009 (Jiang et al., 2024).
In the German forestry sector, 2018–2020 and 2022 are considered dry years (e.g. DFWR, 2021; NW-FVA, 2022). Monthly data from the Earth observation satellites Sentinel-2 and Landsat-8 show dramatic canopy losses in Germany, with coniferous forests in the central part of the country particularly affected – from Saxon Switzerland in the east, through Thuringia, to the Harz Mountains, into the Sauerland region, and finally to the Eifel region in the west (Thonfeld et al., 2022). From January 2018 up to and including April 2021, tree losses were recorded on around 501 000 ha in Germany, which corresponds to 5 % of the total forest area. The results of the German forest condition survey show that in 2018, 29 % of the trees investigated showed moderate-to-severe crown defoliation (≥25 %), which is the highest value since records began in 1984, when it was 23 % (BMEL, 2023a). This value increased to about 26 %–37 % during the years 2019 through 2022. On a regional scale results are the same, e.g. the forest condition survey in the German federal state of Lower Saxony shows that defoliation values are at their highest level in the time series since 1984 (NW-FVA, 2022). High water availability enabled trees to maintain growth in the Leipzig floodplain forest during summer 2018, but the subsequent drought in 2019 caused a significant reduction in tree growth, even in a forest ecosystem with a comparably high water supply, demonstrating the cumulative effect of consecutive drought years (Schnabel et al., 2021).
Even if in Germany deciduous forests are not dying off to the same extent as coniferous stands, they are also strongly affected by climate change. In the forest condition survey (BMEL) of 2020, a record-high number of dead trees was documented across all tree species examined. The survey revealed that only 20 % of trees exhibited no crown thinning, with European beech showing an even-more-pronounced decline – only 11 % of these trees were unaffected. Specifically, older trees (exceeding 60 years of age) and those growing in drier sites experienced notably reduced growth rates and increased mortality. These findings were corroborated by additional studies (e.g. Leuschner, 2020; and Weigel et al., 2023), which highlight the ongoing stress and vulnerability faced by European beech. Even tree species that are considered relatively drought-resistant, such as Scots pine (Pinus sylvestris), have experienced massive mortality since 2018 (e.g. Kunert, 2019, 2020). In this case, in addition to the hot and dry summers, the fungus Spaeropsis sapinea (or Diplodia pinea) causes pine dieback (Mette and Kölling, 2020).
In Germany, outbreaks of the European spruce bark beetle (Ips typographus) have caused widespread damage to forests, particularly during periods of heat and drought. In many cases, there was a need for emergency felling and even deforestation to prevent the pest from spreading (e.g. Thonfeld et al., 2022; Bork et al., 2024). In Thuringia, almost 21×106 m3 of deciduous (mainly beech) and coniferous (mainly spruce) deadwood occurred between 2018 and the end of September 2022, of which around 65 % was due to insect infestation and 35 % due to drought and storms (TMIL, 2022). In 2022, around 344 000 m3 of damaged wood (202 000 m3 of hardwood and 142 000 m3 of coniferous wood) was registered due to drought alone, without the primary pests being involved. In the period of 2018–2022, 4.9×106 m3 of damaged wood resulted from heat and drought (TMIL, 2022). It is estimated that around 500 000 ha, or 4.4 % of Germany's forest area, has been damaged by climate impacts, fires, and bark beetles. These areas will need reforestation to mitigate the impacts of the drought from 2018 to 2022 (BMEL, 2023c). For the approximately 13.3×106 m3 of wood damaged by bark beetles, 95.6 % is due to activities of the European spruce bark beetle and 2.8 % to the spruce wood engraver (Pityogenes chalcographus). Although the latter still plays a subordinate role, it could gain increasing importance given that it specialises in younger spruce stands. This is a large-scale threat in the future in terms of reforestation or rejuvenation with conifers (TMIL, 2022).
The years 2018, 2019, and 2022 were also above average for forest fires in Germany (DWD, 2022; UBA, 2023a). The burnt area in 2022 was more than 5 times the annual average of almost 776 ha (since 1991), and damage was estimated at EUR 30 to 40 million (Feuerwehrverband, 2022). In Germany during 2018–2019, damage due to natural disturbances was estimated at EUR 2.5 billion (DW, 2019). It is difficult to disentangle the exact costs of a big disturbance in the German forestry sector, which generates about EUR 170 billion annually and employs directly and indirectly more than 1.1 million people (Popkin, 2021). Möhring et al. (2021) estimated the economic damage caused by the extreme weather events of 2018 to 2020 in the forestry sector at more than EUR 12.7 billion, which is 10 times the annual net profit of the entire German forest industry.
In the Netherlands, there are clear signs that trees suffered from the drought and heat of 2018, with deciduous species in particular experiencing stunted or no growth (Salomón et al., 2022). On a national level, the average volume of living wood and deadwood increased during 2017–2021, although at a slower rate due to the dry summers in 2018–2020 (NBI-7, 2022). There are several indications of tree mortality: the volume of standing deadwood compared to the NBI-6 (2012–2013) shows an increase from 6.1 to 10.0 m3 ha−1 from 2012–2013 (NBI-6) to 2017–2021 (NBI-7), respectively, and lying deadwood increased from 6.6 to 9.2 m3 ha−1 for the same periods. However, there is no information on crown defoliation. The next systematic monitoring of forests in the Netherlands started in 2022 and will be completed in 2026.
In the northern part of Belgium (Flanders), new forest plantations have suffered from the droughts, especially on sandy soils: several have died in 2018 without further quantification available (CIW, 2019). In 2019, besides young trees, widespread mortality of mature deciduous trees, as well as Norway spruce and larch, was observed. Oak and beech trees exhibited dead tops or crowns, and dying juvenile trees of chestnut, sycamore, and silver birch were observed (CIW, 2020). Additionally, in 2020 it was reported that several trees exhibited needle and leaf loss and that Norway spruce trees in particular had died (CIW, 2021). The annual forest vitality inventory for Flanders (Sioen et al., 2022) provides information on the state of the forests for each year by monitoring trees at approximately 70 locations, each with a radius of about 18 m. The annual inventories (Sioen et al., 2019, 2020, 2021, 2022, 2023) provide an indication of trends in vitality (e.g. loss of leaves and needles) but do not provide an overall estimate of the total damage to the complete stock of forests and wood in Flanders. Despite the effects of drought in the years 2019–2020, the year 2021 demonstrated some recovery, with a significant reduction in the loss of leaves and needles (as of the time this text was written, data for 2022 had not yet been published). The inventories also show that the number of damaged trees in the samples increased since 2008 (Fig. 16 in Sioen et al., 2022), with a recent peak in 2020 (30 % of broadleaved trees damaged; 20 % of deciduous trees damaged) and a decline in 2021.
In Wallonia, the southern part of Belgium, nearly one-third of the 550 000 ha forest is covered with spruce. Accordingly, mortality has been high throughout Wallonia since the beginning of the drought years in 2018. In 2018, 500 000 m3 of spruce were infested by bark beetles compared to 5000–10 000 m3 in normal years. This number increased to approximately 1×106 m3 in the years 2019 and 2020 (Saintonge et al., 2021). During the colder and wetter year 2021, the newly infested timber volume dropped again to about 500 000 m3 (Saintonge et al., 2021). Wildfires occur in Belgium but not excessively, and they were highest in 2021, causing 659 ha of area to be burned (EFFIS, 2023).
In France, from 2018 to 2020, 300 000 ha were affected by forest dieback in public forests alone (ONF, 2020). The northeast is particularly affected by bark beetles. In the two most-affected regions, Grand Est and Bourgogne-Franche-Comté, 170 000 ha of forest, equivalent to 58×106 m3 of wood, was covered with spruce at elevations below 800 m before the 2018–2022 drought event (Saintonge et al., 2021). The 2018–2019 drought and associated bark beetle damage was the main reason for the dieback (ONF, 2020). Salvage logging of the damaged public forests led to the harvest of 6.5×106 m3 of low-value wood in the period of 2019–2020 compared to less than 1×106 m3 on average in a normal year, which represents 26 % of the total harvest in public forest (ONF, 2021). If the share of affected spruce stands is extrapolated to private forests, 19×106 m3 of spruce can be considered to have been killed by bark beetles in the two most-affected regions in the period 2018–2021 (Saintonge et al., 2021). Interestingly, the damage increases from year to year, reaching a temporary peak of 9×106 m3 in 2021 (Saintonge et al., 2021), although this year was the only one in the period of 2018–2022 that was not particularly hot and dry. The French government has allocated EUR 150 million between 2021 and 2022 to regenerate and adapt the impacted areas (Gouvernement Français, 2020).
The share of harvested wood of all tree species declared as accidental (often related to storm damage) and sanitary (often related to drought damage or insect pests) products in metropolitan France increased from 0.8 % in 2017 to 1.5 % in 2018 (MAA, 2019a), to 5.5 % in 2019 (MAA, 2021a), to 10.6 % or 3.8×106 m3 in 2020 (MAA, 2022a) and 4.1×106 m3 in 2021 (MAA, 2023). Spruce is particularly impacted, with more than 2×106 m3 in 2020 (MAA, 2022a).
In addition, higher defoliation rates have been observed since 2015, which is probably largely due to the droughts and heat waves and the resulting increase in pests. While in 1997 only 2.2 % of the deciduous trees and 1.8 % of the conifers were affected, in 2019 the figures were 9.6 % and 4.3 %, respectively. In addition to Scots pine (Pinus sylvestris) and Norway spruce (Picea abies), European beech (Fagus sylvatica) is particularly affected (Piton et al., 2021).
In terms of wildfires, the situation in France in the period of 2018–2022 is also exceptional. During this period, researchers observed the 3 years (namely 2019, 2021 and 2022) with the largest cumulative wildfire-burnt area since the start of systematic Copernicus observations in 2006. In 2022, the largest cumulative wildfire-burnt area to date was measured; at 66 393 ha, which was more than 13 times higher than the 2006–2017 average (EFFIS, 2023).
3.4 Damage to forests in the Alpine zone, 2018–2022
In Austria, the regions most affected by drought and heat are primarily in the lowlands, particularly in the east (Vienna, Lower Austria, Burgenland), as well as in the southeast (Styria) and the northern foothills of the Alps (Upper Austria, Northern Salzburg). The country experienced severe bark beetle infestations between 2018 and 2022, resulting in significant timber losses, especially in 2018 (5 210 000 m3), 2019 (4 690 000 m3), and 2022 (3 750 000 m3; see Fig. 3). In 2022, forest damage in Austria, primarily attributed to climate change, was estimated at approximately EUR 28 million (Bundesforste, 2023). Around 940 000 m3 of wood was damaged, representing about 59 % of the total wood harvested in 2021. The primary cause of this damage was a significant increase in bark beetle infestations, with these pests now spreading up to the treeline at approximately 2000 m above sea level due to climate change (Bundesforste, 2023). Additionally, in March 2022, a massive wildfire in Allentsteig, Lower Austria, burned approximately 800 ha, including 400 ha of forest, making it one of the largest forest fires in Austria's history (Müller, 2022).
Due to rainfall in the summer months, the Alps are usually less hot and dry than it is in lower areas (climate monitoring of GeoSphere Austria, 2024). A study based on normalised difference vegetation index (NDVI) data confirms that drought impacts decrease with elevation, especially above 1500 m (Rita et al., 2020).
Damage caused by forest insects in Austria was only sporadically detected, such as in East Tyrol during 2022 (CIPRA, 2022). In March 2022, a significant wildfire occurred in Tyrol, near the German border, where around 35 ha of mountain forest was destroyed in Pinswang (SZ, 2022; Merkur, 2024). The total direct costs for firefighting and necessary measures for burnt areas in the Alpine region, excluding preventive actions, are estimated to be around EUR 75 million per year (Müller et al., 2020).
In Switzerland, in 2018 and 2022, the canopy of numerous beech trees had already changed colour by the end of July, with extensive areas of the forest in the Mendrisiotto region appearing brown by August (WSL, 2022). The volume of spruce wood damaged by bark beetle calamities amounts to approximately 800 000 m3 in 2018, twice as high as in 2017. In 2019, the volume increased further to 1.5×106 m3 before decreasing in 2020 (Dubach et al., 2021) and 2021 to 1.2×106 m3 and 600 000 m3, respectively, given the colder and wetter spring and summer (Saintonge et al., 2021). A study based on Swiss National Forest Inventory (NFI) data (5092 NFI plots) up to 2017 showed that only 14 % of the Swiss forests were classified as naturally disturbed, most of them (59 %) by wind, 16 % by insects (predominantly bark beetle), 1.2 % by fire, and 1.6 % by drought (Scherrer et al., 2022). The interim results of the fifth state forest inventory (NFI5) over the survey years 2018 to 2022 clearly show that there is an increase in dead and damaged trees (WSL, 2023b). Spruce has declined in the Jura, the Mittelland, and the foothills of the Alps, while the sweet chestnut has declined on the southern side of the Alps. The decline in ash trees, attributed to ash dieback caused by the fungus Hymenoscyphus fraxineus, spread rapidly and reached the inner Alpine valleys within a few years, with East Tyrol being affected by 2010 at the latest (Heinze, 2017). In addition, fewer young trees are growing in a quarter of all forests throughout Switzerland. The Alps, and especially the southern side of the Alps, are particularly affected. Besides the interim results of NFI5, only a few reports were found for high altitudes in Switzerland, as, for example, on a regional increase in bark beetles in the Alps in 2020 (e.g. Schreiner Zeitung, 2020; SRF, 2020).
In Italy, following the Vaia windstorm in 2018, pest activity was initially moderate. However, a significant heat wave in early June 2021 triggered a massive swarming of the spruce bark beetle (Agrar- & Forstbericht Südtirol, 2021). By 2022, around 5000 ha of the 350 000 ha of forest in South Tyrol was infested with the bark beetle (Tagesschau, 2022). The pest then rapidly spread in Tyrol from mid-May 2022 (CIPRA, 2022), with approximately 105 000 m3 of wood affected in 2021 and around 1×106 m3 in 2022. The total amount of damaged wood from 2018 to 2022 is roughly equivalent to 15 times the normal annual harvest (Dolomitenstadt, 2023).
Additionally, a prolonged drought during the 2017 growing season led to the most-extensive outbreak of simultaneous fires in the Alpine region in the past 30 years. In the Piemonte region, 11 large fires occurred in the autumn of 2017, burning nearly 10 000 ha of mostly broadleaved forests within a week (Müller et al., 2020). Furthermore, in October 2018, one of Italy's largest forest fires occurred in Monte San Lucano, Veneto, burning 632 ha (Müller et al., 2020).
3.5 Damage to forests in the southern zone, 2018–2022
Italy was not under extreme drought conditions in spring and summer 2018 (Senf and Seidl, 2021b; Rousi et al., 2023), but it suffered from extended forest damage caused by the extratropical windstorm Vaia over northeastern Italy in autumn 2018 (Motta et al., 2018). Vaia damage accounted for more than 70 % of the total roundwood removed in Italy in the year 2018 (Pilli et al., 2021). Although there was no extreme drought in northern Italy in 2018, the precipitation was below normal for the months April, June, and September (Desiato et al., 2018), which might have contributed to the forests being drier than normal and thus more vulnerable to Storm Vaia in October 2018. Italy did suffer from an extreme heatwave and drought in 2017, which contributed to significant wildfire activity and a subsequent burned-forest total of 161 987 ha, the highest annual total since 2007 (European Commission, 2018; RAF Italia 2017–2018, 2019).
In general during 1998–2021, there was an increase in defoliation, forest mortality, and leaf discolouration in Italian forests, especially in montane conifer forests, with peaks reached in 2021 (Bussotti et al., 2022) and leaf discolouration mainly observed in deciduous and evergreen oak forests. These high damage levels in 2021 are a result of a combination of increased summer drought and the lagging effect of Storm Vaia of 2018 that compromised the stability of the trees and increased the probability of insect attacks due to high accumulation of deadwood in the forests (Bussotti et al., 2022).
The summer of 2022 was affected by a severe-to-extreme meteorological drought (Toreti et al., 2022a). Northern Italy was strongly affected, facing the warmest and driest winter on record in the last 30 years (Toreti et al., 2022b). It resulted in strong hydrological drought and unusually low streamflow of the Po River, which was also related to the lack of snow in the Italian Alps that winter (Koehler et al., 2022). A study looking at the impacts of the 2017–2022 drought and heatwaves in forest areas in Tuscany found that the most-severe impacts, including defoliation and mortality, were observed in the tall evergreen Mediterranean woodlands and in the aged coppices (holm oaks; Bussotti et al., 2023). The study suggests that the impact of the 2022 prolonged drought on forests could have been larger, but trees might be responding to current climate change via rapid acclimation based on epigenetic modifications (Rico et al., 2014).
Due to an increase in precipitation during 2018–2019 in Spain, there was some recovery or stabilisation in terms of forest defoliation and discolouration following the drought of 2017 (AIEF, 2019). However, more-recent reports over areas in northeastern Spain reveal an increase in defoliation during 2019–2021 due to more-severe heat and drought conditions and, in particular, due to extreme events occurring during critical vegetation growth periods (GAN-NIK, 2019). In the period of 2018–2020, physical damage such as drought and wind were the main drivers of forest defoliation, followed by insects. Both drivers cause forest damage 3 to 5 times larger than every other driver (fungi, fires, etc.), and their impacts have increased dramatically since 2014 (AIEF, 2020). In this period, physical damage, insects, and forest fires are the three main drivers of tree mortality in Spain (AIEF, 2020). In 2022, the country experienced almost 270 000 ha of burned area, a drastic increase from previous years amounting to up to 3 to 6 times more surface area compared to 2018–2021. In the 2018–2021 period, around 300 fires per year were recorded versus 400 fires in 2022, indicating not only more fires and a larger burned area but also a larger burned area per fire on average (see Fig. 6).
Although Portugal has recently shown increasing frequency of drought conditions coupled with heatwave events (Bezak and Mikoš, 2020; Vogel et al., 2021; Ribeiro et al., 2020), leading to the exacerbation of limiting climatic conditions for plant growth, the situation of Maritime pine (Pinus pinaster, one of the most-common species) is, according to Kurz-Besson et al. (2016), not completely discouraging. Detailed information regarding defoliation and wood damaged by insects in Portugal is unavailable since 2006 (ICP Forests, 2007).
Since 1980, the mean annual burnt area has been around 115 000 ha, with a large interannual variability and with particularly severe years, such as 2003 (∼ 425 000 ha), 2005 (∼ 350 000 ha), or the record value in 2017 (∼ 540 000 ha; EFFIS, 2023). The interannual variability in burned areas in Portugal is attributable to high temperatures and drought, which are influenced by the amount of precipitation during and before the fire season (from May to September). In addition, atmospheric circulation patterns in the summer induce extremely hot and dry spells over western Iberia (Pereira et al., 2005; Russo et al., 2017). Dry conditions contributed extensively to the massive wildfires that took place in Portugal during 2017 (Turco et al., 2019; San-Miguel-Ayanz et al., 2020). The total burned area in Portugal in 2017 corresponds to nearly 60 % of the total burned area in Europe in 2017. The economic losses due to the 2017 wildfires totalled around EUR 1 billion, and the local insurance sector declared it to be the costliest natural disaster in the country's history, with pay-outs exceedingly around EUR 270 million (Global Fire Monitoring Center, 2018; AON, 2018).
According to Global Forest Watch, Portugal experienced significant TCL from 2001 to 2021, totalling approximately 1.13×106 ha. A notable portion of this loss occurred in 2017 alone, with 226 000 ha lost primarily due to wildfires. In comparison, the cumulative tree cover loss from 2018 to 2022 amounted to 188 000 ha. The loss during this period was predominantly driven by deforestation, with permanent deforestation mainly attributed to urbanisation and shifting agriculture.
4.1 Drought legacy effects
Drought and heat impact vegetation not only immediately but can also have long-term effects that persist for years. Short-term damage assessments often underestimate the overall impact on forest ecosystems. Recovery times vary; for instance, carbon cycle recovery and compositional changes may span several years (Müller and Bahn, 2022). Severe droughts in temperate forests have led to growth reductions lasting up to 6 years, depending on tree species (Orwig and Abrams, 1997). Furthermore, long-term damage assessment is complicated by vegetation adaptation to persistent conditions. For example, pre-existing structural changes in tree hydraulic traits can either mitigate or exacerbate damage, influenced by shifts in plasticity (López et al., 2016). Trees in drier environments often show greater drought resistance (Orwig and Abrams, 1997).
Assessing the impact of consecutive drought years involves disentangling the effects of specific events from ongoing conditions that may influence hazard levels. This task includes evaluating long-term changes in water availability due to extreme droughts and understanding the legacy damage to forest ecosystems from the 2018–2022 drought events. While this section focuses on damage, it is important to recognise that long-term positive effects can also arise following extreme climate events (Müller and Bahn, 2022).
4.2 Linking vegetation drought legacy with groundwater drought legacy
GW is a key component of the terrestrial water cycle, contributing dynamics and feedback to vegetation processes on timescales far beyond the weather and seasonal timescales (Aeschbach-Hertig and Gleeson, 2012), which are especially important for the development and persistence of droughts. The vegetation water supply under meteorological and hydrological drought is determined by the redistribution of moisture in the shallow subsurface (soil) and its hydraulic connection with GW (Yu et al., 2017). Thus, the impact and legacy of drought strongly depends on the local and regional distribution of soil moisture, infiltration, and GW recharge (e.g. capillary rise and baseflow along the rivers). These fluxes and their spatiotemporal dynamics are a function of the subsurface heterogeneity, land surface processes, and climatology. The feedback of GW on vegetation is strongly non-linear and occurs via the capillary rise of water from the free water table or direct extraction of water from GW due to root water uptake. Both processes can be especially pronounced under drought conditions and depend on the vegetation type and associated root depth distribution (Fan et al., 2017). In turn, if the free GW table is at the critical depth along, e.g. a hillslope, even small changes on the order of 10−1 m may result in significant feedback on root water uptake and changes in evapotranspiration (Kollet et al., 2008). For example, Rabbel et al. (2018) showed sap flow density data for a Norway spruce stand in the Eifel Mountains, Germany, from observations in a riparian zone and nearby hillslope exhibiting shallow and deeper water table depth. In the riparian zone, the shallow-rooting spruce exhibited generally large evapotranspiration compared to the hillslope. Thus, GW drought legacy that is manifested in increased GW table depths will impact drought legacy effects in forests in all types of vegetation and land surface processes. Because water use by vegetation is consumptive, vegetation constitutes a sink for GW under these conditions. Thus, a positive-feedback loop may arise in which GW drought legacy influences vegetation drought, and in turn, vegetation influences GW drought legacy. Since the timescale of GW drought legacy acts far beyond the weather and seasonal timescale (Van Loon, 2015; Hellwig et al., 2020), one can expect a strong connection to shallow-moisture redistribution and drought legacy over very large timescales in regions of critical GW depths.
To assess the connection of drought legacy with GW drought legacy from observations, the state of GW (including soil water) must be known in space and time. The state of GW is usually observed in boreholes via in situ GW table or piezometric head measurements. These measurements provide information at the point scale in space and commonly at low frequency in time because they are usually performed manually and thus not logged continuously. This leads to discontinuous images of the GW state in space–time, which is commonly interpolated with the help of models, inversion, and data assimilation. Note, however, that no collated GW observational database exists over Europe or for specific countries. Thus, the data remain fragmented and dispersed across many political and private institutions, and they are not publicly available. This renders a formal analysis of the connection infeasible within the scope of this study, and only the general principles can be discussed here.
In northern and central Europe, dispersed borehole observations of GW levels revealed that the 2018 drought was indeed one of the most severe in decades and comparable to the drought in 1976 (Bakke et al., 2020; Hellwig et al., 2020). In 2018 in many observation wells, GW levels were at or close to the lowest levels ever observed by in situ measurements (Bakke et al., 2020), resulting in the cessation of capillary rise, a reduction in root water uptake, and severe drought stress, even beyond the year 2018 (Schuldt et al., 2020). For example, Süßel and Brü (2021) studied tree–water relations in 2018 in mature oak stands in southwest Germany. They found that sites with continuous capillary rise toward the root zone maintained a canopy conductance at 50 % of the maximum, while sites with hydraulic disconnection from the water table showed a collapse of conductance and significant leaf shedding. In these settings, the long-term effect of droughts may be especially pronounced because GW recovery after drought is a slow process leading to strong memory effects and an increased probability of drought at the interannual timescale, which was indeed observed in the ensuing years (2019 and 2020) in addition to precipitation deficits (Hartick et al., 2021). It is important to note that vegetation stress under the 2018 to 2022 drought conditions showed distinct spatial patterns, with limited stress along river corridors and extreme stress in the upper parts of hillslopes along ridges (Cartwright et al., 2020). These patterns are directly related to GW processes, specifically GW discharge and recharge, respectively. Under drought conditions, along river corridors, GW discharges as baseflow toward the stream, constituting essentially an outcrop of the GW table, thus leading to shallow GW tables connected to the land surface via capillary rise and root water uptake. In contrast, along hillslopes and ridges, capillary rise for root water uptake is mainly sustained by shallow soil water without a connection to the GW compartment, leading to tight coupling of root water uptake and plant stress with quite-limited soil moisture storage. In the case of GW, these patterns are well-known and are shown by in situ GW measurements. However, the lack of remote sensing information for the subsurface, data scarcity, and fragmentation lead to much-more-incomplete spatial coverage of information. Preliminary inspection of leaf area index (LAI) products from remote sensing does not show a systematic pattern at the large scale dependent on topography or the potential GW convergence zone. In the future, a merger of in situ, remotely sensed, and model data with ensuing in-depth analyses will be required to identify potential tree and forest legacy effects induced by GW drought legacy. In this context, data from hyperspectral remote sensing regarding photosynthetic activity may be useful.
4.3 Drought legacy effects in forests – the accumulation of long-term damage due to soil moisture deficit
Drought events can leave longer-lasting impacts on forests, depending on which tree demographic processes are most affected (Müller and Bahn, 2022; Rukh et al., 2023). Adult tree mortality can create gaps in forests, altering carbon and water cycles, species composition, and long-term profitability. These gaps also increase understorey solar radiation, temperature, and soil dryness, which can lead to further damage through soil hydrophobicity and nutrient loss (Grünzweig et al., 2022). Similarly, the death or weakened vitality of saplings can hinder forest recovery, leaving trees vulnerable to future droughts, storms, fires, and pests (Gliksman et al., 2023). A study from Matías Resina et al. (2020) showed that the impact of drought on tree level resilience was not strongly dependent on its latitudinal location but rather on the type of sites the trees were growing on and their growth performance (i.e. magnitude and variability in growth) during the pre-drought period. Examples of drought damage during 2018–2022 in European forests highlight these impacts. The most-pronounced legacy effects involved saplings and young trees, with long-term seedling establishment varying by location and species (Salomón et al., 2022). In central eastern Germany, the 2018 drought caused 65 % defoliation in saplings across multiple species, with some species suffering over 85 % defoliation. Despite some recovery, 25 %–32 % of saplings still showed damage in 2020 (Beloiu et al., 2022). In Scotland, the droughts in 2018 and 2020 caused significant losses, including 50 000 seedlings at a Sitka spruce farm in Galloway and notable mortality rates in privately managed young forests (Locatelli et al., 2021). In Poland's Brodnica forest district, 20 % of trees planted died, leading to replanting costs of approximately EUR 33 000 (LASY, 2023). Similar damage was observed in young spruce and beech trees across Germany (BMEL, 2020). Growth reductions occurred in northern Germany following the 2018 drought, exacerbated by insufficient winter water recharge, with similar reductions in 2019 and 2020 (Beloiu et al., 2022). In Switzerland, forest gross primary productivity recovered in about 50 % of forested area by 2019, while 49 % remained at 2018 damage levels, indicating a strong legacy effect (Sturm, 2022).
Overall, the findings of this study emphasise that the central zone of Europe is the most vulnerable to drought years, such as those between 2018 and 2022, due to its specific forest composition, climate conditions, and susceptibility to secondary effects like pest infestations. The southern zone, although significantly affected by TCL, exhibited less-severe impacts compared to the central zone. The relatively smaller impact in the southern zone could be attributed to the region's long-term exposure to drought, which may have fostered adaptive mechanisms and built resilience over time. At the same time, the varying occurrence of drought conditions along the southern zone may also have contributed to the moderate impact. In the northern zone, the first impacts of drought and heat start to emerge, although the severity is not yet pronounced. The presence of site-adapted boreal forest tree species is likely to contribute to the region's overall resistance. The Alpine zone displayed the least impact, which may highlight the potential protective role of altitude in mitigating the effects of climate extremes.
5.1 Central zone
The central European forests experienced severe impacts during the drought years, 2018–2022, with both coniferous and deciduous species suffering significant damage. Notable crown thinning, particularly among broadleaved species, was observed in France, with similar trends observed in the Czech Republic and Germany. These observations indicate that broadleaved trees across central Europe are increasingly vulnerable to climate-change-related stressors. Conifer defoliation was especially pronounced in the Czech Republic, Belgium, France, and Germany. The region also witnessed high levels of forest damage from pest infestation, underscoring the susceptibility of central European forests to secondary drought effects, such as increased pest activity. The significant rise in TCL and bark-beetle-infested wood highlights the profound impact of prolonged water deficits on these ecosystems, suggesting that the resilience of central Europe's forests is being severely tested by climatic stressors.
The intense drought in 2018, characterised by an exceptionally hot summer, led to early wilting in about 11 % of central European forests, with central and east Germany and the Czech Republic being the most affected (Brun et al., 2020; Buras et al., 2021). These drought conditions, combined with above-average water vapour pressure deficits in subsequent years, were the primary drivers of forest disturbances affecting around 4.74×106 ha between 2018 and 2020, particularly in Germany, the Czech Republic, and Austria (Senf et al., 2021). The physiological damage from 2018, marked by reduced greenness in Austria, Germany, and Switzerland, significantly contributed to forest mortality, and the reduced greenness persisted into 2019 (Schuldt et al., 2020; Brun et al., 2020). The record-hot summer of 2022 further exacerbated this trend, with forest greenness decreasing more sharply than in any other summer since 2002, surpassing even the 2018 drought record (Hermann et al., 2023; Buras et al., 2023).
The prevalence of spruce bark beetles in central Europe has increased over recent decades (Fernandez-Carrillo et al., 2020). From 2018 to 2022, drought and heat triggered an unprecedented outbreak, severely affecting standing timber, particularly in the Czech Republic, Germany, and Austria (Hlásny et al., 2019, 2021; Nardi et al., 2023; Kautz et al., 2023). In 2019, over 50 % of timber harvests in Austria and Germany, and over 90 % in the Czech Republic, were associated with salvage logging due to bark beetle damage (Senf and Seidl, 2021a). The vulnerability of Norwegian spruce monocultures significantly contributed to this damage. Projections suggest a potential 7-fold increase in bark beetle disturbances by 2030 compared to 1971–1980 (Seidl et al., 2014), with a possible 2-fold increase throughout the 21st century depending on climate conditions and forest management practices (Dobor et al., 2020a, b). The cumulative growing stock affected by bark beetles is expected to rise significantly under moderate climate change scenarios, with even greater impacts under more-extreme conditions (Sommerfeld et al., 202).
In addition to drought, storm impacts must also be considered. While there is no definitive evidence of a significant increase in storm frequency in Germany, windthrow damage notably increased during 2018–2022 (BMEL, 2023a). The trend towards milder winters and increased precipitation outside the growing season in parts of central Europe may contribute to greater windthrow susceptibility, as heavy rainfall can weaken root systems, and drought-stressed stands are more prone to wind damage (Středová et al., 2020; UBA 2023b).
Economic losses in central Europe's forestry sector during 2018–2022 were substantial, although precise estimates are challenging due to an incomplete understanding of the full economic impacts (Knoke et al., 2021). While direct damage such as the loss of immature trees can be quantified, more-complex factors like stand destabilisation, market price fluctuations, and impacts on forest workers and machinery are difficult to assess.
5.2 Southern zone
In the southern zone, crown thinning in deciduous trees was particularly pronounced in Italy and Spain. This indicates that even regions well-adapted to dry conditions, which have fostered the development of various adaptive mechanisms in both plant species and forest ecosystems, experienced unprecedented stress during these years. Mediterranean vegetation in the southern zone seems to cope better with seasonal droughts through physiological and structural adaptations, such as deep rooting systems and reduced leaf area. Access of roots to deep water reserves enables hydraulic redistribution, whereby plants transport water from moist, deep to dry, shallow soil layers through their root systems along a water potential gradient (Prieto et al., 2012). This mechanism, occurring frequently in the southern zone (Kurz-Besson et al., 2006; Peñuelas and Filella, 2003), improves plant nutrition, extends root lifespan, and preserves hydraulic conductance in the xylem during dry periods.
Observed damage attributed to increasing temperatures and drought conditions in the southern zone shows that forests are encountering significant repercussions. Data on damage caused by wood-boring insects are unavailable, suggesting that insect pests may not have posed a major threat between 2018 to 2022. Nevertheless, a significant increase in TCL compared to the reference period was observed. Assessing the incidence of wildfires during the period of 2018–2022 was not possible. However, the exceptionally severe wildfires in 2017 with staggering losses, particularly in Portugal, necessitated their inclusion in this study. The devastation caused by wildfires presents a continuously growing challenge for southern Europe, despite wildfires generally being a part of the southwestern European ecosystems. Italy was strongly affected by the windstorm Vaia in 2018. We found no increase in insect infestation during the period from 2018 to 2022 nor in the years prior. Up to 2018, 3×106 ha of forest has been reported as having been converted into shrublands or grasslands in the European Union Mediterranean countries. Fire and drought are the main drivers underlying this deforestation (Karavani et al., 2018). In Spain, forest health showed some recovery between 2018 and 2019, contrasting with greater damage in central Europe (AIEF, 2019; Blunden and Arndt, 2019).
The situation for Maritime pine (Pinus pinaster, one of the most-common species) in Iberia is not completely discouraging. According to Kurz-Besson et al. (2016), wood radial growth and density highly benefit from the strong decrease in cold days and the increase in minimum temperature. Yet, the benefits are hindered by long-term water deficit, which results in different levels of impact on wood radial growth and density. Despite the intensification of long-term water deficit, tree ring width appears to benefit from the minimum temperature increase, whereas the effects of long-term droughts significantly affect tree ring density. Since the particularly extreme year 2017, stringent prevention and rapid response measures have been implemented in the area. When comparing the periods 2007–2017 and 2018–2022, the total number of fires has decreased by half, particularly on days of high fire danger. Larger fires have occurred less frequently since 2017. The average number of fires burning more than 1000 ha decreased from 19 events to just 8 in recent years. Although forest losses are decreasing in the latest period, Portugal experienced an increasing trend in forest area loss due to fires between 2001 and 2019 (Tyukavina et al., 2022). Without the unique events in 2017, the decline in fire incidents might not have been as apparent. This highlights the challenges of interpreting long-term fire trends, as exceptional circumstances can significantly impact annual statistics. Furthermore, the effective implementation of prevention strategies and rapid response efforts in the Iberian Peninsula have played a substantial role in mitigating fire damage (e.g. REA, 2024).
5.3 Northern zone
The relatively low impact observed in the northern zone suggests that it has not yet experienced the full extent of climate extremes or that its forests are more resilient. Nonetheless, the data indicate potential vulnerability to future drought impacts, highlighting the need for ongoing monitoring and conservation efforts. It is important to note that the lack of data on crown defoliation for broadleaves limits a comprehensive assessment of the situation.
The northern zone's forests might benefit from a reduced severity of climate extremes i.e. more consistent precipitation patterns and cooler temperatures, which reduce evapotranspiration rates and alleviate drought stress. Several indices based on Europe-wide data support this assumption, showing that the northern zone was still affected during the 2018–2022 drought period. Specifically, evidence from Sweden reveals significant insect damage to coniferous wood, with high levels recorded in 2018 and even higher levels in subsequent years (2019–2021). Additionally, TCL increased markedly, from 0.7 % to over 1 %.
Overall, in Fennoscandia, forest management during the last decades has favoured conifer monocultures, causing Norway spruce and Scots pine to be the dominant species (Huuskonen et al., 2021). This means that the large forest ecosystems may be more vulnerable to climate extremes. For conifers, however, no significant differences in defoliation were observed in the northern zone or within individual countries within this zone. This suggests a relative stability of conifer health in this region despite variations in environmental conditions. Overall, our findings suggest that conifers in the northern zone exhibit a greater resilience to drought and heat stress compared to those in other regions.
The example of Norway may make it clear that Fennoscandia is probably the area where climate change has had fewer consequences thus far for forest ecosystems. In Norway, larger seasonal differences in precipitation/drought and temperature are expected. Periods of drought are replaced by periods of heavy rains and flooding. The consequences are moderate for forests but can be severe for agriculture in particular during dry seasons. So far, the effects seem to cancel each other out (Miljødirektoratet, 2023; Bardalen et al., 2022). For example, while winter, spring, and summer in 2021 were dry, Norway experienced an autumn and winter with more rain than usual, with GW levels that went above normal. Insect attacks after the 2018 drought could have become severe, but cold and wet preceding years probably mitigated the impact. Overall, the major concern in Norway is periods of drought followed by periods of heavy rainfall leading to passing floods.
In the British Isles, the damage during the study period was not exceptional. Indirect signs of previous drought impacts were noted, with 77 % of UK respondents agreeing on the need for protective measures against pests and diseases (Forest Research, 2021). An earlier spatial-modelling study (Forest Research, 2008) even predicted an improvement in tree growth due to a warmer climate in Scotland in the future, particularly in southern and eastern Scotland for high-quality broadleaved trees, on suitable deep fertile soils, and for conifers on sites where water and nutrients are not limiting. However, a breeding population of the European spruce bark beetle (Ips typographus) has now become established in southeast England, likely arriving by flight across the English Channel following a large-scale dispersal from continental Europe due to extreme weather in 2021–2022 (Inward et al., 2024). This poses a future threat to the spruce in the UK, which is the dominant timber species. It should also be noted that when it comes to drought damage recorded in England and Scotland in 2018, wildfires only ranked third, while impacts on freshwater ecosystems and water quality ranked higher (Turner et al., 2021).
5.4 Alpine zone
The Alpine zone exhibited minimal impact, with no statistically significant differences observed in any forest health or damage indicator used in this study. This limited impact may be attributed to the region's higher altitudes, which might provide mitigating effects such as cooler temperatures or reduced evapotranspiration, potentially buffering the area from extreme drought conditions. But it should be noted that mountain forests are particularly under pressure from climate change impacts due to their temperature limitation and high exposure to warming (Albrich et al., 2020). Such impacts can vary greatly with elevation and topography (e.g. Lindner et al., 2010; Thrippleton et al., 2020) and require a careful study addressing the target species and the abiotic conditions. The main tree species in central European mountain forests are Norway spruce, European beech, and silver fir. All of them are late successional, shade tolerant (Dyderski et al., 2023), and sensitive to drought stress. Additionally, drought can also destabilise mountain forests and result in soil erosion, landslides, and rockfalls. Warmer temperatures, reduced precipitation, and shorter cold periods can lead to reduced snow cover and trigger the distribution of harmful organisms or alien and invasive species that have an impact on biodiversity (Eriksen and Hauri, 2021). Since the length of the growing season decreases with altitude, a warmer climate could also lead to more growth as long as there is sufficient access to water, as confirmed by previous studies (e.g. Thom and Seidl, 2022; Dyderski et al., 2023). Treelines will shift upwards over a longer period, and tree species from the lowlands will establish at higher altitudes. A simulation of forest dynamics in the northern Alps predicts, for the first half of the current century, the probability of increasing gains in stem density, structural complexity, and tree species diversity (Thom et al., 2022). An inventory of Alpine drought impact reports conducted by Stephan et al. (2021) reveals that pre-Alpine areas experience more-significant effects compared to higher elevations. The majority of reported impacts are related to agriculture and public water supply, with less focus on forestry and terrestrial ecosystems. Drought impacts are found to be most severe during summer and early autumn, likely due to the mitigating effect of spring snowmelt on water shortages. The analysis also highlights spatial variability across the Alps, with notably greater impacts observed in the northern Alpine regions. Eriksen and Hauri (2021) mentioned that forest fires have traditionally been more common on the southern side of the Alps, and the countries have introduced better forest fire management strategies.
5.5 Forest fire and tree cover loss
Contrary to our expectations, no significant differences in forest fire outbreaks were observed between the dry period (2018–2022) and the reference period (2010–2014). This trend was consistent across the northern, central, Alpine, and southern zones. Additionally, consultations with local offices, such as those in Austria, confirmed that there were neither more fires nor larger burnt areas during 2018–2022 compared to the reference period. The absence of significant differences in wildfire damage across all zones suggests that the fire prevention measures implemented, such as enhanced forest and fire management, monitoring, and rapid detection and response, as well as international collaboration, might play a more substantial role than drought conditions alone (e.g. REA, 2024). In Nordic countries, for example, differences in early detection, forest road density, and the number of local fire brigades contribute to variations in forest fire incidence and damage (Lehtonen and Venäläinen, 2020). Wildfires in the Alps are influenced by a range of factors, including the high level of human activity driven by recreational activities (Garbarino et al., 2020; Müller et al., 2020). Consequently, there is a complex interplay of elements affecting fire activity, including climatic conditions, forest management practices, preventive measures, public awareness, and the effectiveness of firefighting efforts. The countries in the southern zone experienced severe impacts from forest fires and not just during the 2018–2022 period. Our decision to include data from 2017, despite not being originally part of the study design, provided insights into the significant impact of fires during that year, especially in Portugal where a vast area of forest land was affected. This emphasises the importance of considering extreme events and their implications for forest management and conservation efforts. Further research is needed to explore the underlying drivers of fire activity and to develop effective strategies to mitigate the impacts of forest fires in vulnerable regions. For Alpine forests, data availability was limited to Austria and Switzerland for both periods, showing no significant differences in fire damage. Identifying trends in fire risk in the Alps is challenging due to differences in forest fire documentation systems between Alpine countries (Müller et al., 2020). Based on the available data for 2018–2022, the occurrence of forest fires in the Alps appears consistent with the long-term average. Although our study found no increase in forest fires in Europe during the hot and dry period of 2018–2020, research in the USA has clearly linked the rising frequency and severity of forest fires to climate change. For instance, northern and central California experienced a 5-fold increase in summer burned-forest area during 1996–2021 compared to 1971–1995 (Turco et al., 2023). In the western United States, climate change and other factors have doubled the cumulative forest fire area since 1984 (Abatzoglou and Williams, 2016). Global projections for the 21st century suggest that climate change will worsen fire weather conditions, affecting a significant portion of the burnable land worldwide (Abatzoglou et al., 2019). The significant disparities in TCL observed across European regions between the dry period of 2018–2022 and the reference period of 2010–2014 highlight the complex interactions between human activities, natural phenomena, and climate change, emphasising the importance of comprehensive forest management strategies to mitigate the impacts of environmental changes on forest ecosystems. The escalating frequency and intensity of extreme weather events such as storms, droughts, and wildfires pose significant threats to forest health and resilience. However, forests are under increasing pressure, not only from climate extremes but also from human activities such as logging, deforestation, and urbanisation, underscoring the urgent need for proactive measures to address these challenges. Further research is needed to better understand the specific drivers behind the disparities in reporting and to develop targeted interventions for sustainable forest conservation and management.
5.6 Future trends, biophysical feedback, and impacts on forests
Future global warming is expected to lead to more-frequent and intense periods of hot and dry conditions in European regions (e.g. Seneviratne et al., 2021), which will further enhance climate-related risks to European forests. Furthermore, extreme levels of compound heat and drought stress are projected to occur successively year after year, with a much higher likelihood in the coming decades than in recent years (Suarez-Gutierrez et al., 2023). For example, Hari et al. (2020) found a 7-fold increase in the occurrence of consecutive drought events as of 2018–2019 in Europe under SSP5-8.5 (Shared Socioeconomic Pathway). Gazol and Camarero (2022) expect an increase in forest drought mortality over the next decades due to the more-frequent compounding of extreme drought and heat waves. Martinez del Castillo et al. (2022) project severe future growth declines in European beech forests, ranging from 20 % to more than 50 % by 2090 depending on the region and climate change scenario (i.e. CMIP6 SSP1-2.6 and SSP5-8.5). This is in line with CMIP6 (SSP2-4.5) multi-model mean simulations, which support the notion that mean annual precipitation decreases with increasing proximity to the Mediterranean, linked to roughly similar spatial changes in surface runoff (IPCC 2021a, b). At the same time, evapotranspiration increases the further east in Europe one gets (IPCC 2021a, b). Combined, those two meteorological aspects lead to a pronounced surface-soil-moisture deficit, which increases the (hydrological) drought risk substantially (IPCC 2021a, b). Accordingly, forest disturbance regimes are expected to intensify with continuing global warming, leading to increasing forest biomass losses due to windthrow, fires, and insect outbreaks (Forzieri et al., 2021; Patacca et al., 2023).
Biophysical feedback from land use changes on near-surface temperature can be locally or regionally on the same order of magnitude as those associated with the effect of global greenhouse gas forcing (e.g. de Noblet-Ducoudré et al., 2012). The first regional climate model (RCM) ensemble experiments in the framework of the CORDEX Flagship Pilot Study (FPS LUCAS) investigated the effects of extreme forest cover changes on local and regional climate in Europe (Rechid et al., 2017). Davin et al. (2020) found significant biophysical effects of reforestation and afforestation on regional and local climates seasonally, with RCMs showing consistent winter warming due to albedo changes but differing summer temperature responses due to varying evaporative fractions. Summer temperature changes are mainly driven by land processes, while atmospheric processes dominate winter responses. Breil et al. (2020) found opposing effects of reforestation and afforestation on the diurnal temperature cycle at the surface and in the overlying atmospheric layer. Most RCMs simulate cooler daytime and warmer nighttime summer surface temperatures, aligning with other observational studies. In contrast, the diurnal temperature cycle in the overlying atmospheric surface layer is increased due to higher surface roughness, which increases turbulent heat fluxes. Sofiadis et al. (2022) investigated the impact of reforestation and afforestation on the seasonal soil temperature cycle using the LUCAS RCM ensemble, finding a general reduction in the annual amplitude across Europe, although not all models showed this trend. Observations at paired FLUXNET sites confirmed summer ground cooling in forested areas compared to open areas. While most models align with this trend, variability in change magnitude exists. Daloz et al. (2022) explored the snow–albedo effect of FPS LUCAS RCMs in Sub-polar and Alpine climates, and Mooney et al. (2022) examined extreme forest cover changes within FPS LUCAS simulations. Their findings suggest that reforestation and afforestation reduces the snow-albedo sensitivity index, enhancing snowmelt with robust direction but uncertain magnitude of change. The FPS LUCAS phase 1 simulations highlight the significance of biophysical feedback from forest cover changes in Europe, with potential for intensification under further climate change through regional and local processes.
Our main conclusions from this study are as follows.
-
European forests are highly vulnerable to heat and drought, with even currently resilient ecosystems at significant risk of severe damage in the decades to come.
-
The geographical variability in the distribution of forest damage needs to be integrated into Europe-wide strategies to effectively mitigate future impacts.
-
The study underscores the challenges in data collection and highlights the necessity for harmonised data and enhanced monitoring to address future environmental challenges effectively.
European forests are critically vulnerable to the combined effects of increasing heat and drought, which threaten even those ecosystems currently deemed resilient. This vulnerability is likely to escalate, leading to severe consequences such as heightened tree mortality, shifts in species composition, increased risk of insect pests and wildfires, and diminished forest productivity and carbon sequestration. These potential impacts are far-reaching, undermining the goals of reforestation and climate-smart management efforts (Verkerk et al., 2022; Albrich et al., 2023) and potentially exacerbating local and regional climate extremes (Lejeune et al., 2018).
As extreme heat and drought are projected to intensify, persist longer each year (Hundhausen et al., 2023), and become more frequent (Suarez-Gutierrez et al., 2023), the impacts on forest ecosystems are likely to increase. Central Europe is already facing considerable stress from these conditions, and other regions are expected to experience heightened impacts as well. Global warming is forecasted to prolong thermal summers and shorten winters in northern Europe (Ruosteenoja et al., 2020). The European Alps are predicted to undergo substantial warming throughout the 21st century, accompanied by a marked decrease in snow cover at lower elevations (Kotlarski et al., 2023). Additionally, regions such as the Iberian Peninsula may see persistent drought conditions by the late 21st century (Moemken et al., 2022). These projected changes highlight the urgent need for comprehensive adaptation and mitigation strategies to address the increasing frequency and severity of extreme climate events.
While the extent of damage might have been anticipated, the surprising element is the pronounced heterogeneity in its distribution across different regions. This variability underscores the necessity for Europe-wide strategies that accommodate regional differences. Effective mitigation and adaptation efforts must integrate these diverse regional impacts to comprehensively address and reduce future damage. Overcoming language barriers and improving information accessibility are essential for not only mitigating climate impacts but also raising public awareness of the severe effects of drought and heat. Forest managers must be better equipped to tackle these challenges through adaptive-management techniques and the selection of climate-resilient tree species, mixtures, or provenances. Tailored climate information, such as that demonstrated by Bülow et al. (2024) for the Karlsruhe municipal forest, is crucial for this purpose. Thus, a comprehensive, transdisciplinary approach to managing forest vulnerability should include robust forest management practices – such as species choice, thinning, or prescribed burning – alongside climate adaptation measures, early warning systems, and wildfire risk reduction strategies. Enhancing forest resilience through these measures on a regional scale will be pivotal in addressing future environmental challenges effectively.
The assessment and management of forest damage are significantly complicated by substantial challenges in data collection and reporting. This study highlights notable inconsistencies in impact reporting across sectors and countries, characterised by delays and gaps in data availability. For example, Spain's national forest damage inventories were outdated at the time of this study (AIEF, 2020), and comprehensive data for the Alpine zone were particularly scarce. To illustrate another example, the Swiss stone pine (Pinus cembra), which is crucial to Alpine forests, grows in small, fragmented populations across Switzerland, Germany, Austria, and Italy (EUFORGEN, 2024). Many natural systems extend across national borders, and understanding the impact of climate change on the Alps, as well as other regions, necessitates a broader, cross-national perspective. Additionally, while a description of heat and drought damage to grasslands was planned, limited data availability restricted this assessment despite the critical ecosystem services provided by grasslands. These inconsistencies in data availability impede the ability to rapidly assess multi-country drought impacts and develop effective responses. Addressing these challenges requires the establishment of harmonised data collection and enhanced forest monitoring. A unified, accessible platform for drought damage data and improved cross-linguistic and cross-sectoral communication are essential for effective impact assessment and response formulation.
To effectively address the complex challenges posed by recurrent heat waves and droughts, a comprehensive and collaborative approach is essential. The impacts of these extreme climate events extend beyond forests, affecting water resources, air quality, recreation, wood supply, and overall human wellbeing, and can also heighten risks such as political instability through forest fires and climate feedback. Recent extreme weather has highlighted deficiencies in current preparedness and the critical need for enhanced information accessibility for forest managers. Developing adaptive management techniques and climate-resilient forest strategies requires the joint efforts of researchers, policymakers, and forest managers. Integrating forest management, climate change adaptation, and global greenhouse gas reduction strategies is crucial for mitigating future environmental impacts and ensuring broader ecological and societal stability.
The majority of the data are publicly available, with detailed information on data sources provided in Sect. 1.2. In a few instances, data on insect infestations were obtained through direct requests to relevant state agencies or statistical offices.
The supplement related to this article is available online at: https://doi.org/10.5194/nhess-25-77-2025-supplement.
FK and DG conceptualised the outline of the study. Meteorological data and writing were contributed by KH, JPG, ER, LS-G, AR, EX, DR, and DG. Data collection and the writing of the first draft for the damage data were divided by zones: the northern zone (MRJ, J-PP, BG, FK), the central zone (PA, LMB, CB, KP-C, BG, DG, FK), the Alpine zone (MMM, PA, LS-G, CB, BG, FK), and the southern zone (ER, AR, LS-G, CB, BG, FK). The “Drought legacy” section was developed by SK, DG, and JMG, while “Future trends, biophysical feedback, and impacts on forests” were addressed by DR. Graphical depictions were prepared by FK and SV. All authors collected data and information and contributed to writing and revisions of the manuscript.
At least one of the (co-)authors is a member of the editorial board of Natural Hazards and Earth System Sciences. The peer-review process was guided by an independent editor, and the authors also have no other competing interests to declare.
Publisher's note: Copernicus Publications remains neutral with regard to jurisdictional claims made in the text, published maps, institutional affiliations, or any other geographical representation in this paper. While Copernicus Publications makes every effort to include appropriate place names, the final responsibility lies with the authors.
This article is part of the special issue “Past and future European atmospheric extreme events under climate change”. It is not associated with a conference.
We sincerely thank the editor, Andreas Hense, as well as Holger Lange and the two anonymous reviewers for their valuable comments and suggestions, which have significantly improved the quality of this paper.
This research has been supported by the Bundesministerium für Bildung und Forschung (BMBF), project ClimXtreme (Florian Knutzen, grant no. 01LP2324H; Efi Rousi, grant no. 01LP1901E,; Joaquim G. Pinto, grant no. 01LP1901A; Elena Xoplaki, grant no. 01LP1903C; Barry Gardiner, grant no. 01LP2324L; Laura Suarez-Gutierrez, grant no. 01LP2322E; and Daniel Gliksman, grant no. 01LP1903F). Laura Suarez-Gutierrez has received funding from the European Union’s Horizon Europe framework programme under Marie Skłodowska-Curie grant agreement no. 101064940. Ana Russo was supported by the Portuguese Fundação para a Ciência e a Tecnologia (FCT) I.P./MCTES through national funds (PIDDAC) – UIDB/50019/2020 IDL, DHEFEUS – 2022.09185.PTDC, and 2022.01167.CEECIND. Caterina Barrasso received financial support from the German Federal Ministry of Education and Research (BMBF) and the Saxon State Ministry of Science, Culture, and Tourism (SMWK) through funding of the Center for Scalable Data Analytics and Artificial Intelligence Dresden/Leipzig, project identification number SCADS24B. Joaquim G. Pinto received support from the AXA research fund. Mortimer M. Müller was supported by the IGNITE project (WF M6/Antragsnr. BMLRT/III-2021-M6/2 (FAI.36)) and FIREDATA project (WF M6/Antragsnr. BMLRT/III-2022-M6/1 (FAI.38)) funded by the Waldfonds of the Federal Ministry for Agriculture, Forestry, Regions and Water Management. Diana Rechid and Marius Rohde Johannessen were supported by the project “OptForEU – OPTimising FORest management decisions for a low-carbon, climate resilient future in Europe” Horizon Europe research and innovation programme under grant agreement no. 101060554. Elena Xoplaki was supported by the EU Horizon 2020 Project CLINT under grant agreement number 101003876, the BMWK project DAKI-FWS (grant no. 01MK21009I), and the EU Horizon Europe project MedEWSa under grant agreement no. 101121192.
The article processing charges for this open-access publication were covered by the Helmholtz-Zentrum Hereon.
This paper was edited by Andreas Hense and reviewed by Holger Lange and two anonymous referees.
Aalto, J. and Venäläinen, A. (Eds.): Climate change and forest management affect forest fire risk in Fennoscandia, Finnish Meteorological Institute Reports 2021:3, Helsinki, 156 pp., http://hdl.handle.net/10138/330898 (last access: 19 November 2024), 2021.
Abatzoglou, J. T. and Williams, A. P.: Impact of anthropogenic climate change on wildfire across western US forests, P. Natl. Acad. Sci. USA, 113, 11770–11775, https://doi.org/10.1073/pnas.1607171113, 2016.
Abatzoglou, J. T., Williams, A. P., and Barbero, R.: Global emergence of anthropogenic climate change in fire weather indices, Geophys. Res. Lett., 46, 326–336, https://doi.org/10.1029/2018GL080959, 2019.
Aeschbach-Hertig, W. and Gleeson, T.: Regional strategies for the accelerating global problem of groundwater depletion, Nat. Geosci., 5, 853–861, https://doi.org/10.1038/ngeo1617, 2012.
Agrar-&Forstbericht Südtirol: Bericht über das Jahr 2021, https://www.provinz.bz.it/land-forstwirtschaft/landwirtschaft/publikationen.asp?publ_action=300&publ_image_id=616940 (last access: 30 October 2024), 2021.
AIEF: Memoria del Inventario Español de Especies Terrestres, https://www.miteco.gob.es/es/biodiversidad/temas/inventarios-nacionales/es-00-memoria-19-dist_tcm30-524045.pdf (last access: 19 November 2024), 2019.
AIEF: Informe de Estado de la Diversidad Forestal en España, https://www.miteco.gob.es/es/biodiversidad/temas/inventarios-nacionales/idf2020_tcm30-524136.pdf (last access: 19 November 2024), 2020.
Albrich, K., Rammer, W., and Seidl, R.: Climate change causes critical transitions and irreversible alterations of mountain forests, Glob. Change Biol., 26, 4013–4027, https://doi.org/10.1111/gcb.15118, 2020.
Albrich, K., Seidl, R., Rammer, W., and Thom, D.: From sink to source: changing climate and disturbance regimes could tip the 21st century carbon balance of an unmanaged mountain forest landscape, Forestry, 96, 399–409, https://doi.org/10.1093/forestry/cpac022, 2023.
Allen, C. D., Macalady, A. K., Chenchouni, H., Bachelet, D., McDowell, N., Vennetier, M., Kitzberger, T., Rigling, A., Breshears, D. D., Hogg, E. H. (Ted), Gonzalez, P., Fensham, R., Zhang, Z., Castro, J., Demidova, N., Lim, J.-H., Allard, G., Running, S. W., Semerci, A., and Cobb, N.: A global overview of drought and heat-induced tree mortality reveals emerging climate change risks for forests, Forest Ecol. Manage., 259, 660–684, https://doi.org/10.1016/j.foreco.2009.09.001, 2010.
Allen, C. D., Breshears, D. D., and McDowell, N. G.: On underestimation of global vulnerability to tree mortality and forest die-off from hotter drought in the Anthropocene, Ecosphere, 6, 1–55, https://doi.org/10.1890/ES15-00203.1, 2015.
Alpconv: The Alps in 25 Maps: https://www.alpconv.org/fileadmin/user_upload/Publications/25maps.pdf (last access: 28 November 2024), 2018.
Anderegg, W. R., Kane, J. M., and Anderegg, L. D.: Consequences of widespread tree mortality triggered by drought and temperature stress, Nat. Clim. Change, 3, 30–36, https://doi.org/10.1038/nclimate1635, 2013.
AON: Weather, Climate, and Catastrophe Insight, https://www.aon.com/reinsurance/getmedia/1b516e4d-c5fa-4086-9393-5e6afb0eeded/20220125-2021-weather-climate-catastrophe-insight.pdf (last access: 30 October 2024), 2018.
APA: Seca, https://rea.apambiente.pt/content/seca (last access: 27 June 2023), 2023.
Bader, S., Collaud Coen, M., Duguay-Tetzlaff, A., Frei, C., Fukutome, S., Gehrig, R., Maillard Barras, E., Martucci, G., Romanens, G., Scherrer, S., Schlegel, T., Spirig, Ch., Stübi, R., Vuilleumier, L., and Zubler, E.: Klimareport 2018, Berichte & Bulletins, Bundesamt für Meteorologie und Klimatologie MeteoSchweiz, Bern, Switzerland, ISSN 2296-1488, 2019.
Banerjee, T., De Roo, F., and Mauder, M.: Explaining the convector effect in canopy turbulence by means of large-eddy simulation, Hydrol. Earth Syst. Sci., 21, 2987–3000, https://doi.org/10.5194/hess-21-2987-2017, 2017.
Bakke, S. J., Ionita, M., and Tallaksen, L. M.: The 2018 northern European hydrological drought and its drivers in a historical perspective, Hydrol. Earth Syst. Sci., 24, 5621–5653, https://doi.org/10.5194/hess-24-5621-2020, 2020.
Bakke, S. J., Ionita, M., and Tallaksen, L. M.: Recent European drying and its link to prevailing large-scale atmospheric patterns, Sci. Rep., 13, 21921, https://doi.org/10.1038/s41598-023-48861-4, 2023.
Bardalen, A., Pettersen, I., Dombu, S. V., Rosnes, O., Mittenzwei, K., and Skulstad, A.: Klimaendring utfordrer det norske matsystemet. Kunnskapsgrunnlag for vurdering av klimarisiko i verdikjeder med matsystemet som case, NIBIO rapport 8 (110), https://hdl.handle.net/11250/3013268 (last access: 27 November 2024), 2022.
Bastos, A., Orth, R., Reichstein, M., Ciais, P., Viovy, N., Zaehle, S., Anthoni, P., Arneth, A., Gentine, P., Joetzjer, E., Lienert, S., Loughran, T., McGuire, P. C., O, S., Pongratz, J., and Sitch, S.: Vulnerability of European ecosystems to two compound dry and hot summers in 2018 and 2019, Earth Syst. Dynam., 12, 1015–1035, https://doi.org/10.5194/esd-12-1015-2021, 2021.
BBC: Droughts in England and their impacts, BBC News, https://www.bbc.com/news/uk-england-lancashire-44853173 (last access: 14 March 2023), 2018.
Beguería, S., Vicente-Serrano, S. M., Reig, F., and Latorre, B.: Standardized precipitation evapotranspiration index (SPEI) revisited: parameter fitting, evapotranspiration models, tools, datasets and drought monitoring, Int. J. Climatol., 33, 2308–2319, 2013.
Beloiu, M., Stahlmann, R., and Beierkuhnlein, C.: Drought impacts in forest canopy and deciduous tree saplings in Central European forests, Forest Ecol. Manag., 509, 120075, https://doi.org/10.1016/j.foreco.2022.120075, 2022.
Bento, V. A., Ribeiro, A. F., Russo, A., Gouveia, C. M., Cardoso, R. M., and Soares, P. M.: The impact of climate change in wheat and barley yields in the Iberian Peninsula, Sci. Rep., 11, 1–12, 2021.
Beobide-Arsuaga, G., Düsterhus, A., Müller, W. A., Barnes, E. A., and Baehr, J.: Spring Regional Sea Surface Temperatures as a Precursor of European Summer Heatwaves, Geophys. Res. Lett., 50, e2022GL100727, https://doi.org/10.1029/2022gl100727, 2023.
Bezak, N. and Mikoš, M.: Changes in the compound drought and extreme heat occurrence in the 1961–2018 period at the European scale, Water, 12, 3543, 2020.
BFW: Borkenkaefer und Sturmschäden in Österreich bis 2020, https://www.bfw.gv.at/wp-content/uploads/Abb_Borkenkaefer_SturmSchnee_bis2020_Oesterreich.pdf (last access: 15 November 2024), 2020.
BFW: Borkenkäferbefall in Südösterreich, https://www.bfw.gv.at/pressemeldungen/borkenkaefer-fichtenwaelder-im-sueden-oesterreichs-stark-betroffen/ (last access: 15 November 2024), 2023.
Blunden, J. and Arndt, D. S.: State of the Climate in 2018, B. Am. Meteorol. Soc., 100, Si-S306, https://doi.org/10.1175/2019BAMSStateoftheClimate.1, 2019.
Bundesforste: Waldbilanz 2022, https://www.bundesforste.at/service-presse/presse/pressedetail/news/bundesforste-waldbilanz-2022-gepraegt-von-hitze-trockenheit-und-kaefer.html (last access: 15 November 2024), 2023.
BMEL: Ergebnisse der Waldzustandserhebung 2019, https://www.bmel.de/SharedDocs/Downloads/DE/_Wald/ergebnisse-waldzustandserhebung-2019.pdf?__blob=publicationFile&v=11 (last access: 19 November 2024), 2020.
BMEL: Ergebnisse der Waldzustandserhebung 2022, https://www.bmel-statistik.de/fileadmin/daten/0320000-2022.pdf (last access: 19 November 2024), 2023a.
BMEL: Internationaler Tag des Waldes 2021, https://www.bmel.de/DE/themen/wald/waelder-weltweit/tag-des-waldes.html (last access: 19 November 2024), 2023b.
Bork, K., Triebenbacher, C., and Hahn, A.: Schadholz muss schnell raus, BLW, 5, 48–50, 2024.
Boergens, E., Güntner, A., Dobslaw, H., and Dahle, C.: Quantifying the Central European droughts in 2018 and 2019 with GRACE Follow-On, Geophys. Res. Lett., 47, e2020GL087285, https://doi.org/10.1029/2020GL087285, 2020.
Bonaldo, D., Bellafiore, D., Ferrarin, C., Ferretti, R., Ricchi, A., Sangelantoni, L., and Vitelletti, M. L.: The summer 2022 drought: a taste of future climate for the Po valley (Italy)?, Reg. Environ. Change, 23, 1, https://doi.org/10.1007/s10113-022-02004-z, 2023.
Brás, T. A., Seixas, J., Carvalhais, N., and Jägermeyr, J.: Severity of drought and heatwave crop losses tripled over the last five decades in Europe, Environ. Res. Lett., 16, 065012, https://doi.org/10.1088/1748-9326/abf004, 2021.
Breil, M., Rechid, D., Davin, E. L., de Noblet-Ducoudré, N., Katragkou, E., Cardoso, R. M., Hoffmann, P., Jach, L. L., Soares, P. M. M., Sofiadis, G., Strada, S., Strandberg, G., Tölle, M. H., and Warrach-Sagi, K.: The opposing effects of reforestation and afforestation on the diurnal temperature cycle at the surface and in the lowest atmospheric model level in the European summer, J. Climate, 33, 9159–9179, 2020.
Brecka, A. F., Shahi, C., and Chen, H. Y.: Climate change impacts on boreal forest timber supply, Forest Policy Econ., 92, 11–21, 2018.
Brodribb, T. J., Powers, J., Cochard, H., and Choat, B.: Hanging by a thread? Forests and drought, Science, 368, 261–266, 2020.
Brun, P., Psomas, A., Ginzler, C., Thuiller, W., Zappa, M., and Zimmermann, N. E.: Large-scale early-wilting response of Central European forests to the 2018 extreme drought, Glob. Change Biol., 26, 7021–7035, 2020.
Büntgen, U., Urban, O., Krusic, P. J., Rybníček, M., Kolář, T., Kyncl, T., Ač, A., Koňasová, E., Čáslavský, J., Esper, J., Wagner, S., Saurer, M., Tegel, W., Dobrovolný, P., Cherubini, P., Reinig, F., and Trnka, M.: Recent European drought extremes beyond Common Era background variability, Nat. Geosci., 14, 190–196, 2021.
Bülow, K., Bauer, S., Steuri, B., Groth, M., Knutzen, F., and Rechid, D.: Stadtwald Karlsruhe im Klimawandel – Der Wald heute und in Zukunft, Zenodo, https://doi.org/10.5281/zenodo.11473737, 2024.
Buras, A., Rammig, A., and Zang, C. S.: The European Forest Condition Monitor: using remotely sensed forest greenness to identify hot spots of forest decline, Front. Plant Sci., 12, 689220, https://doi.org/10.3389/fpls.2021.689220, 2021.
Buras, A., Meyer, B., and Rammig, A.: Record reduction in European forest canopy greenness during the 2022 drought, EGU General Assembly 2023, Vienna, Austria, 24–28 April 2023, EGU23-8927, https://doi.org/10.5194/egusphere-egu23-8927, 2023.
Bussotti, F., Papitto, G., Di Martino, D., Cocciufa, C., Cindolo, C., Cenni, E., Bettini, D., Iacopetti, G., and Pollastrini, M.: Le condizioni delle foreste italiane stanno peggiorando a causa di eventi climatici estremi? Evidenze dalle reti di monitoraggio nazionali ICP Forests – CON.ECO.FOR., Forest@, 19, 74–81, https://doi.org/10.3832/efor4134-019, 2022.
Bussotti, F., Bettini, D., Carrari, E., Selvi, F., and Pollastrini, M.: Cambiamenti climatici in atto: osservazioni sugli impatti degli eventi siccitosi sulle foreste toscane, Forest@, 20, 1–9, https://doi.org/10.3832/efor4224-019, 2023.
Byrne, M. P. and O'Gorman, P. A.: Link between land-ocean warming contrast and surface relative humidities in simulations with coupled climate models, Geophys. Res. Lett., 40, 5223–5227, https://doi.org/10.1002/grl.50971, 2013.
Caesar, L., Rahmstorf, S., Robinson, A., Feulner, G., and Saba, V.: Observed fingerprint of a weakening Atlantic Ocean overturning circulation, Nature, 556, 191–196, https://doi.org/10.1038/s41586-018-0006-5, 2018.
Christidis, N., and Stott, P. A.: The influence of anthropogenic climate change on wet and dry summers in Europe, Sci. Bull., 66, 813–823, https://doi.org/10.1016/j.scib.2021.01.020, 2021.
CIPRA: Bergwald im Klimawandel, https://www.cipra.org/de/news/bergwald-im-klimawandel (last access: 15 November 2024), 2022.
CIW: Evaluatierapport waterschaarste en droogte 2018, https://www.integraalwaterbeleid.be/nl/nieuws/downloads-van-nieuwsberichten/evaluatierapport-waterschaarste-en-droogte-2018 (last access: 16 August 2024), 2019.
CIW: Evaluatierapport waterschaarste en droogte 2019, https://www.integraalwaterbeleid.be/nl/nieuws/downloads-van-nieuwsberichten/evaluatierapport-waterschaarste-en-droogte-2019 (last access: 16 August 2024), 2020.
CIW: Evaluatierapport waterschaarste en droogte 2020, https://www.integraalwaterbeleid.be/nl/overleg/droogtecommissie/evaluatierapport-waterschaarste-en-droogte-2020-1 (last access: 16 August 2024), 2021.
Copernicus: Mapping of emergency response for the 2023 floods, https://emergency.copernicus.eu/mapping/list-of-components/EMSR281 (last access: 15 November 2024), 2023.
Cartwright, J. M., Littlefield, C. E., Michalak, J. L., Lawler, J. J., and Dobrowski, S. Z.: Topographic, soil, and climate drivers of drought sensitivity in forests and shrublands of the Pacific Northwest, USA, Sci. Rep., 10, 18486, https://doi.org/10.1038/s41598-020-75273-5, 2020.
Daloz, A. S., Schwingshackl, C., Mooney, P., Strada, S., Rechid, D., Davin, E. L., Katragkou, E., de Noblet-Ducoudré, N., Belda, M., Halenka, T., Breil, M., Cardoso, R. M., Hoffmann, P., Lima, D. C. A., Meier, R., Soares, P. M. M., Sofiadis, G., Strandberg, G., Toelle, M. H., and Lund, M. T.: Land–atmosphere interactions in sub-polar and alpine climates in the CORDEX flagship pilot study Land Use and Climate Across Scales (LUCAS) models – Part 1: Evaluation of the snow-albedo effect, The Cryosphere, 16, 2403–2419, https://doi.org/10.5194/tc-16-2403-2022, 2022.
Davies, G. M., Gray, A., Rein, G., and Legg, C. J.: Peat consumption and carbon loss due to smouldering wildfire in a temperate peatland, Forest Ecol. Manag., 308, 169–177, https://doi.org/10.1016/j.foreco.2013.07.001, 2013.
Davies, S., Bathgate, S., Petr, M., Gale, A., Patenaude, G., and Perks, M.: Drought risk to timber production – A risk versus return comparison of commercial conifer species in Scotland, Forest Policy Econ., 117, 102189, https://doi.org/10.1016/j.forpol.2020.102189, 2020.
Davin, E. L., Rechid, D., Breil, M., Cardoso, R. M., Coppola, E., Hoffmann, P., Jach, L. L., Katragkou, E., de Noblet-Ducoudré, N., Radtke, K., Raffa, M., Soares, P. M. M., Sofiadis, G., Strada, S., Strandberg, G., Tölle, M. H., Warrach-Sagi, K., and Wulfmeyer, V.: Biogeophysical impacts of forestation in Europe: first results from the LUCAS (Land Use and Climate Across Scales) regional climate model intercomparison, Earth Syst. Dynam., 11, 183–200, https://doi.org/10.5194/esd-11-183-2020, 2020.
DAV: Waldbrand und Klima, https://magazin.alpenverein.de/artikel/waldbrand-klima-mensch_a8c8fe7a-67c8-417c-9e65-95835ba16f17 (last access: 19 November 2024), 2022.
de Noblet-Ducoudré, N., Boisier, J. P., Pitman, A., Bonan, G. B., Brovkin, V., Cruz, F., and Voldoire, A.: Determining robust impacts of land-use-induced land cover changes on surface climate over North America and Eurasia: results from the first set of LUCID experiments, J. Climate, 25, 3261–3281, https://doi.org/10.1175/JCLI-D-11-00338.1, 2012.
DAERA: Wildfire damage across Mournes assessed by DAERA and partner agencies, https://www.daera-ni.gov.uk/news/wildfire-damage-across-mournes-assessed-by-daera-and-partner-agencies (last access: 19 November 2024), 2022.
Danielewicz, W. (Ed.): Dąbrowy Krotoszyńskie monografia przyrodniczo-gospodarcza, G&P Oficyna Wydawnicza, PTL, Poznań, ISBN 978-83-7272-318-5, 2016.
Desiato, F., Fioravanti, G., Fraschetti, P., Perconti, W., Piervitali, E., and Pavan, V.: Gli indicatori del clima in Italia nel 2018 – ISPRA Report, https://www.isprambiente.gov.it/it/pubblicazioni/stato-dellambiente/gli-indicatori-del-clima-in-italia-nel-2018 (last access: 24 July 2022), 2018.
DFWR: Schäden durch Fowi, https://dfwr.de/wp-content/uploads/2022/01/DFWR-Position-Schaeden-Fowi-Langfassung-Studie.pdf (last access: 15 August 2024), 2021.
Drouard, M., Kornhuber, K., and Woollings, T.: Disentangling dynamic contributions to summer 2018 anomalous weather over Europe, Geophys. Res. Lett., 46, 12537–12546, https://doi.org/10.1029/2019GL084601, 2019.
Dubach, V., Beenken, L., Bader, M., Odermatt, O., Stroheker, S., Hölling, D., treenet, Vögtli, I., Augustinus, B. A., and Queloz, V.: Protection des forêts – Vue d'ensemble 2020, WSL Ber. 110, 57 p., https://doi.org/10.3929/ethz-a-004498101, 2021.
Duchez, A., Frajka-Williams, E., Josey, S. A., Evans, D. G., Grist, J. P., Marsh, R., McCarthy, G. D., Sinha, B., Berry, D. I., and Hirschi, J. J.-M.: Drivers of exceptionally cold North Atlantic Ocean temperatures and their link to the 2015 European heat wave, Environ. Res. Lett., 11, 074004, https://doi.org/10.1088/1748-9326/11/7/074004, 2016.
DESTATIS: Holzernte 2019, https://www.destatis.de/DE/Presse/Pressemitteilungen/2020/07/PD20_N041_412.html#:~:text=Im Jahr 2019 wurden 46,waren es 54 Millionen Kubikmeter (last access: 15 November 2024), 2020.
DESTATIS: Holzeinschlag, https://www.destatis.de/DE/Themen/ Branchen-Unternehmen/Landwirtschaft-Forstwirtschaft-Fischerei/Wald-Holz/aktuell-holzeinschlag.html (last access: 15 November 2024), 2023.
Dittus, A. J., Collins, M., Sutton, R., and Hawkins, E.: Reversal of projected European summer precipitation decline in a stabilizing climate, Geophys. Res. Lett., 51, e2023GL107448, https://doi.org/10.1029/2023GL107448, 2024.
Dolomitenstadt: 220 Mio. Borkenkäfer gingen in Osttirol in die Falle, https://www.dolomitenstadt.at/2022/08/03/220-mio-borkenkaefer-gingen-in-osttirol-in-die-falle/ (last access: 15 November 2024), 2023.
Dobor, L., Hlásny, T., Rammer, W., Zimová, S., Barka, I., and Seidl, R.: Is salvage logging effectively dampening bark beetle outbreaks and preserving forest carbon stocks?, J. Appl. Ecol., 57, 67–76, 2020a.
Dobor, L., Hlásny, T., and Zimová, S.: Contrasting vulnerability of monospecific and species-diverse forests to wind and bark beetle disturbance: The role of management, Ecol. Evol., 10, 12233–12245, 2020b.
DSB: Skogbrannsesongen 2018, https://www.dsb.no/globalassets/dokumenter/rapporter/skogbrannsesongen_2018_nn.pd (last access: 19 October 2024), 2019.
DWD: https://www.dwd.de/DE/wetter/thema_des_tages/2022/4/20.html (last access: 15 November 2024), 2022.
Dyderski, M. K., Pawlik, Ł., Chwistek, K., and Czarnota, P.: Tree aboveground biomass increment and mortality in temperate mountain forests: Tracing dynamic changes along 25-year monitoring period, For. Ecol. Manag., 540, 121054, https://doi.org/10.1016/j.foreco.2023.121054, 2023.
EC-JRC Drought Reports, https://joint-research-centre.ec.europa.eu/european-and-global-drought-observatories/drought-reports_en (last access: 21 August 2024), 2024.
EFFIS: Annual Statistics for UK, https://effis.jrc.ec.europa.eu/apps/effis.statistics/estimates (last access: 20 March 2023), 2023a.
EFFIS: Annual Statistics for Ireland, https://effis.jrc.ec.europa.eu/apps/effis.statistics/estimates (last access: 20 March 2023), 2023b.
Eriksen, C. and Hauri, A.: Climate Change in the Swiss Alps, CSS Analyses in Security Policy, 290, https://doi.org/10.3929/ethz-b-000496457, 2021.
EUFORGEN: Pinus cembra, https://www.euforgen.org/species/pinus-cembra (last access: 22 August 2024), 2024.
European Commission, Libertà, G., Vivancos, T., Leray, T., Costa, H., San-Miguel-Ayanz, J., Branco, A., Durrant, T., Lana, F., Nuijten, D., Ahlgren, A., Löffler, P., Ferrari, D., De Rigo, D., Boca, R., and Maianti, P.: Forest fires in Europe, Middle East and North Africa 2017, Publ. Off. EU, https://doi.org/10.2760/663443, 2018.
EUROSTAT: Forests and forestry statistics, https://ec.europa.eu/eurostat/documents/3217494/7777899/KS-FK-16-001-EN-N.pdf/cae3c56f-53e2-404a-9e9e-fb5f57ab49e3?t=1484314012000 (last access: 15 November 2024), 2016.
EUROSTAT: Latest statistics on forest and wood products, https://ec.europa.eu/eurostat/web/products-eurostat-news/w/edn-20230321-1 (last access: 24 April 2023), 2023.
Euwid: Sweden assuming 5.1m m3 of beetle-damaged wood for 2022, https://www.euwid-wood-products.com/news/roundwood-sawnwood/sweden-assuming-51m-m3-of-beetle-damaged-wood-for-2022-211222/ (last access: 22 March 2024), 2022.
Fan, Y., Miguez-Macho, G., Jobbágy, E. G., Jackson, R. B., and Otero-Casal, C.: Hydrologic regulation of plant rooting depth, P. Natl. Acad. Sci., 114, 10572–10577, https://doi.org/10.1073/pnas.1712381114, 2017.
Feller, U., Kingston-Smith, A. H., and Centritto, M.: Editorial: abiotic stresses in agroecology: a challenge for whole plant physiology, Front. Environ. Sci., 5, 13, https://doi.org/10.3389/fenvs.2017.00013, 2017.
Feuerwehrverband: Rekord-Waldbrandsommer 2022: Fast 4300 Hektar Wald verbrannt – Waldeigentümer und Feuerwehren fordern finanzielle Unterstützung für Präventionsmaßnahmen, https://www.feuerwehrverband.de/rekord-waldbrandsommer-2022-fast-4300-hektar-wald-verbrannt-waldeigentuemer-und-feuerwehren-fordern-finanzielle-unterstuetzung-fuer-praeventionsmassnahmen/ (last access: 15 November 2024), 2022.
Fernandez-Carrillo, A., Patočka, Z., Dobrovolný, L., Franco-Nieto, A., and Revilla-Romero, B.: Monitoring bark beetle forest damage in Central Europe. A remote sensing approach validated with field data, Remote Sens., 12, 3634, https://doi.org/10.3390/rs12213634, 2020.
Feurdean, A., Vannière, B., Finsinger, W., Warren, D., Connor, S. C., Forrest, M., Liakka, J., Panait, A., Werner, C., Andrič, M., Bobek, P., Carter, V. A., Davis, B., Diaconu, A.-C., Dietze, E., Feeser, I., Florescu, G., Gałka, M., Giesecke, T., Jahns, S., Jamrichová, E., Kajukało, K., Kaplan, J., Karpińska-Kołaczek, M., Kołaczek, P., Kuneš, P., Kupriyanov, D., Lamentowicz, M., Lemmen, C., Magyari, E. K., Marcisz, K., Marinova, E., Niamir, A., Novenko, E., Obremska, M., Pędziszewska, A., Pfeiffer, M., Poska, A., Rösch, M., Słowiński, M., Stančikaitė, M., Szal, M., Święta-Musznicka, J., Tanţău, I., Theuerkauf, M., Tonkov, S., Valkó, O., Vassiljev, J., Veski, S., Vincze, I., Wacnik, A., Wiethold, J., and Hickler, T.: Fire hazard modulation by long-term dynamics in land cover and dominant forest type in eastern and central Europe, Biogeosciences, 17, 1213–1230, https://doi.org/10.5194/bg-17-1213-2020, 2020.
Forest health: https://assets.gov.ie/136864/6a39a3ce-3f1d-461b-bbd9-7dd9bf7da570.pdf (last access: 15 November 2024), 2021.
Forest Research: A review of the evidence base on tree health and pests, https://cdn.forestresearch.gov.uk/2008/01/fcrn101.pdf (last access: 20 March 2023), 2008.
Forest Research: Public Opinion of Forestry 2019 – Northern Ireland, https://cdn.forestresearch.gov.uk/2022/03/pof2020ni.pdf (last access: 20 March 2023), 2019.
Forest Research: Public Opinion of Forestry 2021: UK and England, https://cdn.forestresearch.gov.uk/2022/02/pof_uk_eng_2021.pdf (last access: 20 March 2023), 2021.
Forest Research: Provisional Woodland Statistics 2022, https://cdn.forestresearch.gov.uk/2022/06/PWS-statsnotice-16jun22.pdf (last access: 20 March 2023), 2022a.
Forest Research: Forestry Statistics 2022, https://www.forestresearch.gov.uk/tools-and-resources/statistics/forestry-statistics/ (last access: 20 March 2023), 2022b.
Forest Research: Pest and Disease Resources – Phytophthora pluvialis, https://www.forestresearch.gov.uk/tools-and-resources/fthr/pest-and-disease-resources/phytophthora-pluvialis/ (last access: 20 March 2023), 2022c.
Forest Statistics Ireland: https://www.teagasc.ie/media/website/crops/forestry/advice/Forest-Statistics-Ireland-2020.pdf (last access: 20 March 2023), 2020.
Forzieri, G., Girardello, M., Ceccherini, G., Spinoni, J., Feyen, L., Hartmann, H., Beck, P. S. A., Camps-Valls, G., Chirici, G., Mauri, A., and Cescatti, A.: Emergent vulnerability to climate-driven disturbances in European forests, Nat. Commun., 12, 1081, https://doi.org/10.1038/s41467-021-21399-7, 2021.
GAN-NIK: Resultados 2021 Red de Evaluacion Fitosanitaria de las Mas, https://www.navarra.es/NR/rdonlyres/4FC06980-DB33-40F3-8698-3BC9938F0142/480640/Resultados2021ReddeEvaluacionFitosanitariadelasMas.pdf (last access: 20 August 2024), 2019.
Garbarino, M., Morresi, D., Urbinati, C., Malandra, F., Motta, R., Sibona, E. M., Vitali, A., and Weisberg, P. J.: Contrasting land use legacy effects on forest landscape dynamics in the Italian Alps and the Apennines, Landscape Ecol., 35, 2679–2694, https://doi.org/10.1007/s10980-020-01013-9, 2020.
García-León, D., Casanueva, A., Standardi, G., Burgstall, A., Flouris, A. D., and Nybo, L.: Current and projected regional economic impacts of heatwaves in Europe, Nat. Commun., 12, 5807, https://doi.org/10.1038/s41467-021-26050-z, 2021.
García-Herrera, R., Garrido-Perez, J. M., Barriopedro, D., Ordóñez, C., Vicente-Serrano, S. M., Nieto, R., Gimeno, L., Sorí, R., and Yiou, P.: The European 2016/17 Drought, J. Climate, 32, 3169–3187, https://doi.org/10.1175/JCLI-D-18-0331.1, 2019.
Gazol, A. and Camarero, J. J.: Compound climate events increase tree drought mortality across European forests, Sci. Total Environ., 816, 151604, https://doi.org/10.1016/j.scitotenv.2021.151604, 2022.
Gazol, A., Camarero, J. J., Jiménez, J. J., Moret-Fernández, D., López, M. V., Sangüesa-Barreda, G., and Igual, J. M.: Beneath the canopy: Linking drought-induced forest die-off and changes in soil properties, For. Ecol. Manag., 422, 294–302, https://doi.org/10.1016/j.foreco.2018.03.019, 2018.
GeoSphere Austria: Klimamonitoring, https://www.zamg.ac.at/cms/de/klima/klima-aktuell/klimamonitoring/?param=t&period=period-ymd-2024-08-20&ref=1 (last access: 22 August 2024), 2024.
Geological Survey of Sweden: Årsredovisning 2017, https://resource.sgu.se/produkter/broschyrer/arsredovisning-2017.pdf (last access: 15 November 2024), 2017.
George, J. P., Bürkner, P. C., Sanders, T. G., Neumann, M., Cammalleri, C., Vogt, J. V., and Lang, M.: Long-term forest monitoring reveals constant mortality rise in European forests, Plant Biol., 24, 199–209, https://doi.org/10.1111/plb.13430, 2022.
Gliksman, D., Averbeck, P., Becker, N., Gardiner, B., Goldberg, V., Grieger, J., Handorf, D., Haustein, K., Karwat, A., Knutzen, F., Lentink, H. S., Lorenz, R., Niermann, D., Pinto, J. G., Queck, R., Ziemann, A., and Franzke, C. L. E.: Review article: A European perspective on wind and storm damage – from the meteorological background to index-based approaches to assess impacts, Nat. Hazards Earth Syst. Sci., 23, 2171–2201, https://doi.org/10.5194/nhess-23-2171-2023, 2023.
Global Fire Monitoring Center: News, https://gfmc.online/media/2018/02-2018/news_20180223_pt.html (last access: 15 November 2024), 2018.
Greenpeace: Un año horribilis para España: incendios, sequía, olas de calor e inundaciones, https://es.greenpeace.org/es/sala-de-prensa/comunicados/2022-un-ano-horribilis-para-espana-incendios-sequia-olas-de-calor-e-inundaciones/ (last access: April 2023), 2022.
Gimbel, K. F., Puhlmann, H., and Weiler, M.: Does drought alter hydrological functions in forest soils?, Hydrol. Earth Syst. Sci., 20, 1301–1317, https://doi.org/10.5194/hess-20-1301-2016, 2016.
Głowacka, B. (Ed.), Hilszczański, J., Jabłoński, T., Łukaszewicz, J., Skrzecz, I., and Tarwacki, G.: Metodyka integrowanej ochrony drzewostanów iglastych, Instytut Badawczy Leśnictwa, ISBN 978-83-62830-28-2, 2013.
Grodzki, W.: The decline of Norway spruce Picea abies (L.) Karst. stands in Beskid Slaski and Zywiecki: Theoretical concept and reality, Beskydy, 3, 19–26, 2010.
Grünig, M., Seidl, R., and Senf, C.: Increasing aridity causes larger and more severe forest fires across Europe, Glob. Change Biol., 29, 1648–1659, https://doi.org/10.1111/gcb.16385, 2023.
Grünzweig, J. M., de Boeck, H. J., Rey, A., Santos, M. J., Adam, O., Bahn, M., Belnap, J., Deckmyn, G., Dekker, S. C., Flores, O., Gliksman, D., Helman, D., Hultine, K. R., Liu, L., Meron, E., Michael, Y., Sheffer, E., Throop, H. L., Tzuk, O., and Yakir, D.: Dryland mechanisms could widely control ecosystem functioning in a drier and warmer world, Nat. Ecol. Evol., 6, 1064–1076, https://doi.org/10.1038/s41559-022-01779-y, 2022.
Gouvernement Français: France Relance – Toutes les mesures du plan de relance national, https://agriculture.gouv.fr/telecharger/118602 (last access: 15 November 2024), 2020.
Gunther, M.: Uvanlig barkbilleangrep i Vestfold. Forskning.no https://www.forskning.no/insekter-nibio-partner/uvanlig-barkbilleangrep-i-vestfold/1319291 (last access: 17 December 2024). 2019.
Haarsma, R. J., Selten, F. M., and Drijfhout, S. S.: Decelerating Atlantic meridional overturning circulation main cause of future west European summer atmospheric circulation changes, Environ. Res. Lett., 10, 094007, https://doi.org/10.1088/1748-9326/10/9/094007, 2015.
Hanewinkel, M., Cullmann, D. A., Schelhaas, M. J., Nabuurs, G. J., and Zimmermann, N. E.: Climate change may cause severe loss in the economic value of European forest land, Nat. Clim. Change, 3, 203–208, https://doi.org/10.1038/nclimate1687, 2013.
Hanssen-Bauer, I., Hisdal, H., Hygen, H. O., and Mayer, S.: Climate in Norway 2100: a national climate assessment, Norwegian Center for Climate Services report 1/2017, https://www.miljodirektoratet.no/globalassets/publikasjoner/m741/m741.pdf (last access: 27 November 2024), 2017.
Hari, V., Rakovec, O., Markonis, Y., Hanel, M., and Kumar, R.: Increased future occurrences of the exceptional 2018–2019 Central European drought under global warming, Sci. Rep., 10, 1–10, https://doi.org/10.1038/s41598-020-68872-9, 2020.
Hartick, C., Furusho-Percot, C., Goergen, K., and Kollet, S.: An interannual probabilistic assessment of subsurface water storage over Europe using a fully coupled terrestrial model, Water Resour. Res., 57, e2020WR027828, https://doi.org/10.1029/2020WR027828, 2021.
Hauser, M., Gudmundsson, L., Orth, R., Jézéquel, A., Haustein, K., Vautard, R., van Oldenborgh, G. J., Wilcox, L., and Seneviratne, S. I.: Methods and model dependency of extreme event attribution: The 2015 European drought, Earth’s Future, 5, 1034–1043, https://doi.org/10.1002/2017EF000612, 2017.
Heinze, B.: Bei uns und über dem Gartenzaun: die Entwicklung des Eschentriebsterbens in Österreich im europäischen Kontext, BFW-Praxisinformation Nr. 43, 7–12, Translation: Reneema Hazarika/BFW, https://shop.bfw.ac.at/bfw-praxisinformation/bfw-praxisinfo-43-2017.html (last access: 19 November 2024), 2017.
Hellwig, J., de Graaf, I. E. M., Weiler, M., and Stahl, K.: Large-Scale Assessment of Delayed Groundwater Responses to Drought, Water Resour. Res., 56, e2019WR025441, https://doi.org/10.1029/2019WR025441, 2020.
Hemery, G., Petrokofsky, G., Ambrose-Oji, B., Forster, J., Hemery, T., and O'Brien, L.: Awareness, action, and aspirations in the forestry sector in responding to environmental change: Report of the British Woodlands Survey 2020, 33 pp., 2020.
Henley, J.: Europe's rivers run dry as scientists warn drought could be worst in 500 years, https://www.theguardian.com/environment/2022/aug/ (last access: 27 February 2023), 2022.
Hermann, M., Röthlisberger, M., Gessler, A., Rigling, A., Senf, C., Wohlgemuth, T., and Wernli, H.: Meteorological history of low-forest-greenness events in Europe in 2002–2022, Biogeosciences, 20, 1155–1180, https://doi.org/10.5194/bg-20-1155-2023, 2023.
Hewelke, E., Oktaba, L., Gozdowski, D., Kondras, M., Olejniczak, I., and Górska, E. B.: Intensity and persistence of soil water repellency in pine forest soil in a temperate continental climate under drought conditions, Water, 10, 1121, https://doi.org/10.3390/w10091121, 2018.
Highland Council: Scotland's firefighters responded to more than one wildfire a day during spring last year, https://www.highland.gov.uk/news/article/15161/scotland_s_firefighters_responded_to_ more_than_one_wildfire_a_day_during_spring_last_year (last access: 22 March 2023), 2023.
Hicks, L. C., Rahman, M. M., Carnol, M., Verheyen, K., and Rousk, J.: The legacy of mixed planting and precipitation reduction treatments on soil microbial activity, biomass and community composition in a young tree plantation, Soil Biol. Biochem., 124, 227–235, https://doi.org/10.1016/j.soilbio.2018.06.022, 2018.
Hlásny, T., Krokene, P., Liebhold, A., Montagné-Huck, C., Müller, J., Qin, H., Raffa, K., Schelhaas, M.-J., Seidl, R., Svoboda, M., and Viiri, H.: Living with bark beetles: impacts, outlook and management options, No. 8, European Forest Institute, https://doi.org/10.36333/fs08, 2019.
Hlásny, T., Zimová, S., Merganičová, K., Štěpánek, P., Modlinger, R., and Turčáni, M.: Devastating outbreak of bark beetles in the Czech Republic: Drivers, impacts, and management implications, For. Ecol. Manag., 490, 119075, https://doi.org/10.1016/j.foreco.2021.119075, 2021.
Holman, I. P., Hess, T. M., Rey, D., and Knox, J. W.: A multi-level framework for adaptation to drought within temperate agriculture, Front. Environ. Sci., 8, 589871, https://doi.org/10.3389/fenvs.2020.589871, 2021.
Hoy, A., Haensel, S., Skalak, P., Ustrnul, Z., and Bochníček, O.: The extreme European summer of 2015 in a long-term perspective, Int. J. Climatol., 37, 943–962, https://doi.org/10.1002/joc.4722, 2017.
Hundhausen, M., Feldmann, H., Laube, N., and Pinto, J. G.: Future heat extremes and impacts in a convection-permitting climate ensemble over Germany, Nat. Hazards Earth Syst. Sci., 23, 2873–2893, https://doi.org/10.5194/nhess-23-2873-2023, 2023.
Huuskonen, S., Domisch, T., Finér, L., Hantula, J., Hynynen, J., Matala, J., Miina, J., Neuvonen, S., Nevalainen, S., Niemistö, P., Nikula, A., Piria, T., Siitonen, J., Smolander, A., Tonteri, T., Uotila, K., and Viiri, H.: What is the potential for replacing monocultures with mixed-species stands to enhance ecosystem services in boreal forests in Fennoscandia?, For. Ecol. Manag., 479, 118558, https://doi.org/10.1016/j.foreco.2020.118558, 2021.
Hroššo, B., Mezei, P., Potterf, M., Majdák, A., Blaženec, M., Korolyova, N., and Jakuš, R.: Drivers of Spruce Bark Beetle (Ips typographus) Infestations on Downed Trees after Severe Windthrow, Forests, 11, 1290, https://doi.org/10.3390/f11121290, 2020.
ICP forests: Annual Report, https://www.icp-forests.org/pdf/TR2007.pdf (last access: 27 June 2023), 2007.
ICCP: Douville, H., Raghavan, K., Renwick, J., Allan, R. P., Arias, P. A., Barlow, M., Cerezo-Mota, R., Cherchi, A., Gan, T. Y., Gergis, J., Jiang, D., Khan, A., Pokam Mba, W., Rosenfeld, D., Tierney, J., and Zolina, O.: Water Cycle Changes, in: Climate Change 2021: The Physical Science Basis, Contribution of Working Group I to the Sixth Assessment Report of the Intergovernmental Panel on Climate Change, edited by: Masson-Delmotte, V., Zhai, P., Pirani, A., Connors, S. L., Péan, C., Berger, S., Caud, N., Chen, Y., Goldfarb, L., Gomis, M. I., Huang, M., Leitzell, K., Lonnoy, E., Matthews, J. B. R., Maycock, T. K., Waterfield, T., Yelekçi, O., and Yu, R., New York, NY, USA, 1055–1210, https://doi.org/10.1017/9781009157896.010, 2021a.
ICCP: IPCC: Summary for Policymakers, in: Climate Change 2021: The Physical Science Basis, Contribution of Working Group I to the Sixth Assessment Report of the Intergovernmental Panel on Climate Change, edited by: Masson-Delmotte, V., Zhai, P., Pirani, A., Connors, S. L., Péan, C., Berger, S., Caud, N., Chen, Y., Goldfarb, L., Gomis, M. I., Huang, M., Leitzell, K., Lonnoy, E., Matthews, J. B. R., Maycock, T. K., Waterfield, T., Yelekçi, O., Yu, R., and Zhou, B., Cambridge University Press, Cambridge, United Kingdom and New York, NY, USA, 3–32, https://doi.org/10.1017/9781009157896.001, 2021b.
Ilmastokatsaus: Elokuu 2019, https://doi.org/10.35614/ISSN-2341-6408-IK-2019-08-00, 2019.
Ilmastokatsaus: Ilmastovuosikatsaus 2020, https://doi.org/10.35614/ISSN-2341-6408-IVK-2020-00, 2020.
Ilmastokatsaus: Ilmastovuosikatsaus 2021, https://doi.org/10.35614/ISSN-2341-6408-IVK-2021-00, 2021.
Ilmastokatsaus: Ilmastovuosikatsaus 2022, https://doi.org/10.35614/ISSN-2341-6408-IVK-2022-00, 2022.
IMKTRO: Wetter und Klima – Fakten zum Klimawandel – Klimawandel in Mitteleuropa – Niederschlag, https://www.kit.edu/IMKTRO (last access: 21 August 2024), 2023a.
IMKTRO: Wetter und Klima – Fakten zum Klimawandel – Klimawandel in Mitteleuropa – Temperatur, https://www.kit.edu/IMKTRO (last access: 21 August 2024), 2023b.
Inward, D. J. G., Caiti, E., Barnard, K., Hasbroucq, S., Reed, K., and Grégoire, J. C.: Evidence of cross-channel dispersal into England of the forest pest Ips typographus, J. Pest Sci., 97, 1823–1837, https://doi.org/10.1007/s10340-024-01763-4, 2024.
Ionita, M. and Nagavciuc, V.: Changes in drought features at the European level over the last 120 years, Nat. Hazards Earth Syst. Sci., 21, 1685–1701, https://doi.org/10.5194/nhess-21-1685-2021, 2021a.
Ionita, M., Dima, M., Nagavciuc, V., Scholz, P., and Dima, M.: Past megadroughts in central Europe were longer, more severe and less warm than modern droughts, Commun. Earth Environ., 2, 61, https://doi.org/10.1038/s43247-021-00130-w, 2021b.
Ionita, M., Nagavciuc, V., Scholz, P., and Dima, M.: Long-term drought intensification over Europe driven by the weakening trend of the Atlantic Meridional Overturning Circulation, J. Hydrol. Reg. Stud., 42, 101176, https://doi.org/10.1016/J.EJRH.2022.101176, 2022.
Jabłoński, T., Małecka, M., Sierota, Z., Tarwacki, G., Sukovata, L., Sowińska, A., Ślusarski, S., Wolski, R., Plewa, R., Grodzki, W., Szmidla, H., Sikora, K., and Pudełko, M.: Krótkoterminowa prognoza występowania ważniejszych szkodników i chorób infekcyjnych drzew leśnych w Polsce w 2019 r., Instytut Badawczy Leśnictwa, Analizy i Raporty, 26, 160 pp., ISBN 978-83-62830-76-3, 2019a (in Polish).
Jabłoński, T., Tarwacki, G., and Sukovata, L.: Pine forest conditions in Poland in 201–2018, Conf. Pap., Pine forests: current status, existing challenges and ways forward, Kyiv, 2019b.
Jactel, H., Koricheva, J., and Castagneyrol, B.: Responses of forest insect pests to climate change: not so simple, Curr. Opin. Insect Sci., 35, 103–108, https://doi.org/10.1016/j.cois.2019.07.010, 2019.
Jenkins, M. and Schaap, B.: Forest ecosystem services, Background Analytical Study, 1, https://www.un.org/esa/forests/wp-content/uploads/2018/05/UNFF13_BkgdStudy_ForestsEcoServices.pdf (last access: 19 November 2024), 2018.
Jiang, Y., Marchand, W., Rydval, M., Matula, R., Janda, P., Begović, K., Thom, D., Fruleux, A., Buechling, A., Pavlin, J., Nogueira, J., Dušátko, M., Málek, J., Kníř, T., Veber, A., and Svoboda, M.: Drought resistance of major tree species in the Czech Republic, Agric. For. Meteorol., 348, 109933, https://doi.org/10.1016/j.agrformet.2024.109933, 2024.
Karavani, A., Boer, M. M., Baudena, M., Colinas, C., Díaz-Sierra, R., Pemán, J., de Luis, M., Enríquez-de-Salamanca, Á., and Resco de Dios, V.: Fire-induced deforestation in drought-prone Mediterranean forests: drivers and unknowns from leaves to communities, Ecol. Monogr., 88, 141–169, https://doi.org/10.1002/ecm.1285, 2018.
Kautz, M., Peter, F. J., Harms, L., Kammen, S., and Delb, H.: Patterns, drivers and detectability of infestation symptoms following attacks by the European spruce bark beetle, J. Pest Sci., 96, 403–414, 2023.
Kendon, M., McCarthy, M., Jevrejeva, S., Matthews, A., Sparks, T., and Garforth, J.: State of the UK Climate 2020, Int. J. Climatol., https://doi.org/10.1002/joc.7285, 2021.
Kendon, M., McCarthy, M., Jevrejeva, S., Matthews, A., Sparks, T., Garforth, J., and Kennedy, J.: State of the UK Climate 2021, Int. J. Climatol., https://doi.org/10.1002/joc.7787, 2022.
Kendon, M., McCarthy, M., Jevrejeva, S., Matthews, A., Williams, J., Sparks, T., and West, F.: State of the UK Climate 2022, Int. J. Climatol., https://doi.org/10.1002/joc.8167, 2023.
Kirchmeier-Young, M. C., Gillett, N. P., Zwiers, F. W., Cannon, A. J., and Anslow, F. S.: Attribution of the influence of human-induced climate change on an extreme fire season, Earth Fut., 7, 2–10, 2019.
Kirkpatrick Baird, F., Stubbs Partridge, J., and Spray, D.: Anticipating and mitigating projected climate-driven increases in extreme drought in Scotland, 2021–2040, NatureScot Res. Rep., 1228, ISBN 978-1-78391-878-2, 2021.
Knoke, T., Gosling, E., Thom, D., Chreptun, C., Rammig, A., and Seidl, R.: Economic losses from natural disturbances in Norway spruce forests – A quantification using Monte-Carlo simulations, Ecol. Econ., 185, 107046, https://doi.org/10.1016/j.ecolecon.2021.107046, 2021.
Koehler, J., Dietz, A. J., Zellner, P., Baumhoer, C. A., Dirscherl, M., Cattani, L., Vlahović, Ž., Alasawedah, M. H., Mayer, K., Haslinger, K., Bertoldi, G., Jacob, A., and Kuenzer, C.: Drought in Northern Italy: Long Earth Observation Time Series Reveal Snow Line Elevation to Be Several Hundred Meters Above Long-Term Average in 2022, Remote Sens., 14, 6091, https://doi.org/10.3390/rs14236091, 2022.
Kollet, S. J. and Maxwell, R. M.: Capturing the influence of groundwater dynamics on land surface processes using an integrated, distributed watershed model, Water Resour. Res., 44, 2, https://doi.org/10.1029/2007WR006004, 2008.
Kosenius, A.-K., Tulla, T., Horne, P., Vanha-Majamaa, I., and Kerkelä, L.: Economics of forest fire management and ecosystem services – Cost analysis from North Karelia (in Finnish), PTT Working Papers 165, 54 pp., ISBN 978-952-224-157-3, 2014.
Kotlarski, S., Gobiet, A., Morin, S., Olefs, M., Rajczak, J., and Samacoïts, R.: 21st Century alpine climate change, Clim. Dynam., 60, 65–86, 2023.
Kozhoridze, G., Korolyova, N., and Jakuš, R.: Norway spruce susceptibility to bark beetles is associated with increased canopy surface temperature in a year prior disturbance, For. Ecol. Manage., 547, 121400, https://doi.org/10.1016/j.foreco.2023.121400, 2023.
Krikken, F., Lehner, F., Haustein, K., Drobyshev, I., and van Oldenborgh, G. J.: Attribution of the role of climate change in the forest fires in Sweden 2018, Nat. Hazards Earth Syst. Sci., 21, 2169–2179, https://doi.org/10.5194/nhess-21-2169-2021, 2021.
Krumm, F., Rigling, A., Bollmann, K., Brang, P., Dürr, C., Gessler, A., Schuck, A., Schulz-Marty, T., and Winkel, G.: Synthesis: Improving biodiversity conservation in European managed forests needs pragmatic, courageous, and regionally-rooted management approaches, European Forest Institute (EFI), Swiss Federal Institute for Forest, Snow and Landscape Research (WSL), 608–633, 2020.
Kunert, N.: Das Ende der Kiefer als Hauptbaumart in Mittelfranken, AFZ-Der Wald, 3, 24–25, 2019.
Kunert, N.: Preliminary indications for diverging heat and drought sensitivities in Norway spruce and Scots pine in Central Europe, iForest, 13, 89–96, 2020.
Kurz-Besson, C., Otieno, D., Lobo do Vale, R., Siegwolf, R., Schmidt, M., Herd, A., Nogueira, C., David, T. S., David, J. S., Tenhunen, J., Pereira, J. S., and Chaves, M.: Hydraulic lift in cork oak trees in a savannah-type Mediterranean ecosystem and its contribution to the local water balance, Plant Soil, 282, 361–378, https://doi.org/10.1007/s11104-006-0001-5, 2006.
Kurz-Besson, C., Lousada, J. L., Gaspar, M. J., Correia, I., Soares, P. M. M., Cardoso, R. M., Russo, A., Varino, F., Mériaux, C., Trigo, R. M., and Gouveia, C. M.: Effects of recent minimum temperature and water deficit increases on Pinus pinaster radial growth and wood density in Southern Portugal, Front. Plant Sci., 7, 1170, https://doi.org/10.3389/fpls.2016.01170, 2016.
Kwiatkowski, M., Rutkiewicz, A., and Sawicki, A. (Eds.): Klęski żywiołowe w lasach, Instytut Badawczy Leśnictwa, ISBN 978-83-62830-85-5, 2020.
Laaha, G., Gauster, T., Tallaksen, L. M., Vidal, J.-P., Stahl, K., Prudhomme, C., Heudorfer, B., Vlnas, R., Ionita, M., Van Lanen, H. A. J., Adler, M.-J., Caillouet, L., Delus, C., Fendekova, M., Gailliez, S., Hannaford, J., Kingston, D., Van Loon, A. F., Mediero, L., Osuch, M., Romanowicz, R., Sauquet, E., Stagge, J. H., and Wong, W. K.: The European 2015 drought from a hydrological perspective, Hydrol. Earth Syst. Sci., 21, 3001–3024, https://doi.org/10.5194/hess-21-3001-2017, 2017.
LASY: Trudny, upalny czas dla leśników, https://www.lasy.gov.pl/pl/informacje/aktualnosci/trudny-upalny-czas-dla-lesnikow, last access: 27 June 2023.
Lech, P., Żółciak, A., and Hildebrand, R.: Occurrence of European Mistletoe (Viscum album L.) on Forest Trees in Poland and Its Dynamics of Spread in the Period 2008–2018, Forests, 11, 83, https://doi.org/10.3390/f11010083, 2019.
Lech, P., Zajączkowski, G. (Ed.), Boczoń, A., Hildebrand, R., Kluziński, L., Kowalska, J., Małachowska, J., Wawrzoniak, J., and Zajączkowski, G.: Stan zdrowotny lasów Polski w 2020 roku, Instytut Badawczy Leśnictwa, Sękocin Stary, http://www.gios.gov.pl/monlas/raporty/raport_2020/raport_2020.pdf (last access: 28 November 2024), 2021.
Lehtonen, I. and Venäläinen, A.: Metsäpalokesä 2018 muuttuvassa ilmastossa – poikkeuksellinen vuosi vai uusi normaali?, Finn. Meteorol. Inst. Rep., 2020:2, https://doi.org/10.35614/isbn.9789523361089, 2020.
Lejeune, Q., Davin, E. L., Gudmundsson, L., Winckler, J., and Seneviratne, S. I.: Historical deforestation locally increased the intensity of hot days in northern mid-latitudes, Nat. Clim. Change, 8, 386–390, https://doi.org/10.1038/s41558-018-0140-2, 2018.
Leuschner, C.: Drought response of European beech (Fagus sylvatica L.) – a review, Perspect. Plant Ecol. Evol. Syst., https://doi.org/10.1016/j.ppees.2020.125576, 2020.
Leverkus, A. B., García Murillo, P., Jurado Doña, V., and Pausas, J. G.: Wildfires: Opportunity for restoration?, Science, 363, 134–135, https://doi.org/10.1126/science.aaw2134, 2019.
Li, M., Yao, Y., Simmonds, I., Luo, D., Zhong, L., and Chen, X.: Collaborative impact of the NAO and atmospheric blocking on European heatwaves, with a focus on the hot summer of 2018, Environ. Res. Lett., 15, 114003, https://doi.org/10.1088/1748-9326/aba6ad, 2020.
Liebhold, A. M., Brockerhoff, E. G., Kalisz, S., Nuñez, M. A., Wardle, D. A., and Wingfield, M. J.: Biological invasions in forest ecosystems, Biol. Invasions, 19, 3437–3458, https://doi.org/10.1007/s10530-017-1450-7, 2017.
Lindner, M., Maroschek, M., Netherer, S., Kremer, A., Barbati, A., Garcia-Gonzalo, J., Marchetti, M.: Climate change impacts, adaptive capacity, and vulnerability of European forest ecosystems, For. Ecol. Manage., 259, 698–709, https://doi.org/10.1016/j.foreco.2009.09.023, 2010.
Locatelli, T., Beauchamp, K., Perks, M., Xenakis, G., Nicoll, B., and Morison, J.: Drought risk in Scottish forests, Forest Research, https://doi.org/10.7488/era/1292, 2021.
López, R., Cano, F. J., Choat, B., Cochard, H., and Gil, L.: Plasticity in vulnerability to cavitation of Pinus canariensis occurs only at the driest end of an aridity gradient, Front. Plant Sci., 7, 769, https://doi.org/10.3389/fpls.2016.00769, 2016.
MAA (Ministère de l'Agriculture et de l'Alimentation): Récolte de bois et production de sciages en 2018, Agreste Chiffres et Données, n°2019-17, ISSN 2259-5104, 2019a.
MAA (Ministère de l'Agriculture et de l'Alimentation): Récolte de bois et production de sciages en 2019, Agreste Primeur, n°2021-2, ISSN 1760-7132, 2021a.
MAA (Ministère de l'Agriculture et de l'Alimentation): Récolte de bois en 2020. Repli de 2,5 % dans le contexte de l'épidémie de Covid-19, Agreste Primeur, n°2022-2, ISSN 0246-1803, 2022a.
MAA (Ministère de l'Agriculture et de l'Alimentation): Récolte de bois et production de sciages en 2021, Agreste Chiffres et Données, n°2023-3, ISSN 2259-5104, 2023.
Mao, J., Nierop, K. G. J., Dekker, S. C., Dekker, L. W., and Chen, B.: Understanding the mechanisms of soil water repellency from nanoscale to ecosystem scale: a review, J. Soils Sediments, 19, 171–185, https://doi.org/10.1007/s11368-018-2195-9, 2019.
Martinez del Castillo, E., Zang, C. S., Buras, A., and Others: Climate-change-driven growth decline of European beech forests, Commun. Biol., 5, 163, https://doi.org/10.1038/s42003-022-03107-3, 2022.
Matías Resina, L., Bose, A. K., Gessler, A., Bolte, A., Bottero, A., Buras, A., and Cailleret, M.: Growth and resilience responses of Scots pine to extreme droughts across Europe depend on predrought growth conditions, Glob. Change Biol., 26, 4521–4537, https://doi.org/10.1111/gcb.15166, 2020.
Matiu, M., Crespi, A., Bertoldi, G., Carmagnola, C. M., Marty, C., Morin, S., Schöner, W., Cat Berro, D., Chiogna, G., De Gregorio, L., Kotlarski, S., Majone, B., Resch, G., Terzago, S., Valt, M., Beozzo, W., Cianfarra, P., Gouttevin, I., Marcolini, G., Notarnicola, C., Petitta, M., Scherrer, S. C., Strasser, U., Winkler, M., Zebisch, M., Cicogna, A., Cremonini, R., Debernardi, A., Faletto, M., Gaddo, M., Giovannini, L., Mercalli, L., Soubeyroux, J.-M., Sušnik, A., Trenti, A., Urbani, S., and Weilguni, V.: Observed snow depth trends in the European Alps: 1971 to 2019, The Cryosphere, 15, 1343–1382, https://doi.org/10.5194/tc-15-1343-2021, 2021.
Melin, M. (Ed.), Terhonen, E. (Ed.), Aarnio, L., Hantula, J., Helenius, P., Henttonen, H., Huitu, O., Härkönen, M., Isberg, T., Kaitera, J., Kasanen, R., Koivula, M., Kuitunen, P., Korhonen, K. T., Laurila, I., Lindberg, H., Linnakoski, R., Luoranen, J., Matala, J., Niemimaa, J., Nuorteva, H., Piri, T., Poimala, A., Poteri, M., Pouttu, A., Siitonen, J., Silver, T., Strandström, M., Uimari, A., Vainio, E., Vanha-Majamaa, I., Vuorinen, M., and Ylioja, T.: Metsätuhot vuonna 2021 (in Finnish), Luonnonvara- ja biotalouden tutkimus 38/2022, Natural Resources Institute Finland, Helsinki, http://urn.fi/URN:ISBN:978-952-380-423-4 (last access: 19 November 2024), 2022.
Merkur: Schloss Neuschwanstein Waldbrand Gefahr, https://www.merkur.de/bayern/schloss-neuschwanstein-waldbrand-tirol-feuer-gefahr-bayern-feuer-trockenheit-grenze-news-91407046.html, last access: 19 February 2024.
Mette, T. and Kölling, C.: Die Zukunft der Kiefer in Franken, LWF Aktuell, 2, 14–17, 2020.
MET Norway: Tørkesommeren 2018, MetINFO, 14, https://www.met.no/publikasjoner/met-info (last access: 15 November 2024), 2019.
Metsäkeskus: Kirjanpainajatuhojen kasvuun kannattaa varautua, https://www.metsakeskus.fi/fi/ajankohtaista/kirjanpainajatuhojen-kasvuun-kannattaa-varautua, last access: 1 March 2023.
Mezei, P., Fleischer, P., Rozkošný, J., Kurjak, D., Dzurenko, M., Rell, S., Lalík, M., and Galko, J.: Weather conditions and host characteristics drive infestations of sessile oak (Quercus petraea) trap trees by oak bark beetles (Scolytus intricatus), For. Ecol. Manage., 503, 119775, https://doi.org/10.1016/j.foreco.2021.119775, 2022.
Michel, A., Prescher, A.-K., and Schwärzel, K. (Eds.): Forest Condition in Europe: The 2019 Assessment, ICP Forests Technical Report, BFW Austrian Research Centre for Forests, Vienna, ISBN 978-3-903258-17-4, 2019.
Michel, A., Kirchner, T., Prescher, A.-K., and Schwärzel, K. (Eds.): Forest Condition in Europe: The 2020 Assessment, ICP Forests Technical Report, Thünen Institute, https://doi.org/10.3220/ICPTR1606916913000, 2020.
Michel, A., Kirchner, T., Prescher, A.-K., and Schwärzel, K. (Eds.): Forest Condition in Europe: The 2021 Assessment, ICP Forests Technical Report, Thünen Institute, https://doi.org/10.3220/ICPTR1624952851000, 2021.
Michel, A., Kirchner, T., Prescher, A.-K., and Schwärzel, K. (Eds.): Forest Condition in Europe: The 2022 Assessment, ICP Forests Technical Report, Thünen Institute, https://doi.org/10.3220/ICPTR1656330928000, 2022.
Milanovic, S., Markovic, N., Pamucar, D., Gigovic, L., Kostic, P., and Milanovic, S. D.: Forest fire probability mapping in Eastern Serbia: Logistic regression versus random forest method, Forests, 12, 5, https://doi.org/10.3390/f12010005, 2021.
Miljødirektoratet: Klimatiltak i Norge mot 2030: Oppdatert kunnskapsgrunnlag om utslippsreduksjonspotensial, barrierer og mulige virkemidler, Miljødirektoratet: Oslo, https://www.miljodirektoratet.no/publikasjoner/2023/juni-2023/klimatiltak-i-norge-mot-2030/ (last access: 27 November 2024), 2023.
Mitchell, D., Heaviside, C., Schaller, N., Allen, M., Ebi, K. L., Fischer, E. M., and Vardoulakis, S.: Extreme heat-related mortality avoided under Paris Agreement goals, Nat. Clim. Change, 8, 551–553, https://doi.org/10.1038/s41558-018-0210-1, 2018.
Moemken, J., Koerner, B., Ehmele, F., Feldmann, H., and Pinto, J. G.: Recurrence of drought events over Iberia. Part II: Future changes using regional climate projections, Tellus A, 74, 262–279, https://doi.org/10.16993/tellusa.52, 2022.
Mohr, S., Ehret, U., Kunz, M., Ludwig, P., Caldas-Alvarez, A., Daniell, J. E., Ehmele, F., Feldmann, H., Franca, M. J., Gattke, C., Hundhausen, M., Knippertz, P., Küpfer, K., Mühr, B., Pinto, J. G., Quinting, J., Schäfer, A. M., Scheibel, M., Seidel, F., and Wisotzky, C.: A multi-disciplinary analysis of the exceptional flood event of July 2021 in central Europe – Part 1: Event description and analysis, Nat. Hazards Earth Syst. Sci., 23, 525–551, https://doi.org/10.5194/nhess-23-525-2023, 2023.
Möhring, B., Bitter, A., Bub, G., Dieter, M., Dög, M., Hanewinkel, M., Hatzfeld, N., Köhler, J., Ontrup, G., Rosenberger, R., Seintsch, B., and Thoma, F.: Schadenssumme insgesamt 12,7 Mrd. Euro – Abschätzung der ökonomischen Schäden der Extremwetterereignisse der Jahre 2018 bis 2020 in der Forstwirtschaft, Holz-Zentralblatt, 9, 155–158, 2021.
Montanari, A., Nguyen, H., Rubinetti, S., Ceola, S., Galelli, S., Rubino, A., and Zanchettin, D.: Why the 2022 Po River drought is the worst in the past two centuries, Sci. Adv., 9, eadg8304, https://doi.org/10.1126/sciadv.adg8304, 2023.
Mooney, P. A., Rechid, D., Davin, E. L., Katragkou, E., de Noblet-Ducoudré, N., Breil, M., Cardoso, R. M., Daloz, A. S., Hoffmann, P., Lima, D. C. A., Meier, R., Soares, P. M. M., Sofiadis, G., Strada, S., Strandberg, G., Toelle, M. H., and Lund, M. T.: Land–atmosphere interactions in sub-polar and alpine climates in the CORDEX Flagship Pilot Study Land Use and Climate Across Scales (LUCAS) models – Part 2: The role of changing vegetation, The Cryosphere, 16, 1383–1397, https://doi.org/10.5194/tc-16-1383-2022, 2022.
Moravec, V., Markonis, Y., Rakovec, O., Svoboda, M., Trnka, M., Kumar, R., and Hanel, M.: Europe under multi-year droughts: how severe was the 2014–2018 drought period?, Environ. Res. Lett., 16, 034062, https://doi.org/10.1088/1748-9326/abe828, 2021.
Morin, X., Fahse, L., Jactel, H., Scherer-Lorenzen, M., García-Valdés, R., and Bugmann, H.: Long-term response of forest productivity to climate change is mostly driven by change in tree species composition, Sci. Rep., 8, 5627, https://doi.org/10.1038/s41598-018-23763-y, 2018.
Morcillo, L., Gallego, D., González, E., and Vilagrosa, A.: Forest decline triggered by phloem parasitism-related biotic factors in Aleppo pine (Pinus halepensis), Forests, 10, 608, https://doi.org/10.3390/f10080608, 2019.
Motta, R., Ascoli, D., Corona, P., Marchetti, M., and Vacchiano, G.: Selvicoltura e schianti da vento. Il caso della “tempesta Vaia,” For. J. Silvic. For. Ecol., 15, 94, https://doi.org/10.3832/EFOR2990-015, 2018.
Müller, M. M., Vilà-Vilardell, L., Vacik, H., Mayer, C., Mayr, S., Carrega, P., and Maier, H.: Forest fires in the Alps: State of knowledge, future challenges and options for an integrated fire management, EUSALP Action Group, 8, https://doi.org/10.13140/RG.2.2.15609.42081, 2020.
Müller, M. M.: Rekordbrand TÜPl Allentsteig, https://fireblog.boku.ac.at/2022/04/12/rekordbrand-tuepl-allentsteig, last access: 12 April 2022.
Müller, L. M. and Bahn, M.: Drought legacies and ecosystem responses to subsequent drought, Glob. Change Biol., 28, 5086–5103, https://doi.org/10.1111/gcb.16351, 2022.
Nardi, D., Jactel, H., Pagot, E., Samalens, J. C., and Marini, L.: Drought and stand susceptibility to attacks by the European spruce bark beetle: A remote sensing approach, Agric. For. Entomol., 25, 119–129, https://doi.org/10.1111/afe.12533, 2023.
NBI-7: Lerink, B., Schelhaas, M. J., Clerkx, S., Teeuwen, S., Oldenburger, J., and Beerkens, G.: 7e Nederlandse Bosinventarisatie: een gemengde boodschap, Vakblad Natuur Bos Landschap, 19, 8–11, 2022.
Neuvonen, S.: Ilmastonmuutos ja metsien hyönteistuhot (in Finnish), Metsätieteen aikakauskirja, 10498, https://doi.org/10.14214/ma.10498, 2020.
Nevalainen, S. and Pouttu, A. (Eds.): Metsätuhot vuonna 2016 (in Finnish), Luonnonvara- ja biotalouden tutkimus 50/2017, Natural Resources Institute Finland, Helsinki, http://urn.fi/URN:ISBN:978-952-326-447-2 (last access: 15 November 2024), 2017.
NIBIO: Kilden Forest data portal, https://kilden.nibio.no/?lang=nb&topic=skogportal&bgLayer=graatone_cache&X=6773739.61&Y=-94941.10&zoom=1.979699915287064&layers_opacity=0.75,0.75,0.75,0.75&layers=barkbille_registrering_17,barkbille_historiske,barkbille_granressurser,barkbille_utsatte_omrader&catalogNodes=1237,1238 (last access: 15 November 2024), 2023.
NordicWoodJournal: Forest fires in Sweden – huge areas burned in 2018: https://nordicwoodjournal.com/editorial/forest-fires-sweden/ (last access: 20 November 2024).
Norwegian Center for Climate Services: Observations and weather statistics, https://seklima.met.no/observations/ (last access: 15 November 2024), 2023.
NRW: https://naturalresources.wales/about-us/news-and-blogs/news/fires-cause-100k-of-damage-to-afan-valley-and-seven-sister-forests/?lang=en (last access: 18 November 2024), 2020.
Nuorteva, H. (Ed.): Metsätuhot vuonna 2018, Luonnonvara- ja biotalouden tutkimus 85/2019, Natural Resources Institute Finland, Helsinki, http://urn.fi/URN:ISBN:978-952-326-878-4 (last access: 19 November 2024), 2019 (in Finnish).
Nuorteva, H. (Ed.), Kytö, M. (Ed.), Aarnio, L., Ahola, A., Balázs, A., Elfving, R., Haapanen, M., Hantula, J., Henttonen, H., Huitu, O., Ihalainen, A., Kaitera, J., Kuitunen, P., Kashif, M., Korhonen, K. T., Lindberg, H., Linnakoski, R., Matala, J., Melin, M., Neuvonen, S., Niemimaa, J., Pietilä, V., Piri, T., Poteri, M., Pusenius, J., Silver, T., Strandström, M., Tikkanen, O.-P., Uimari, A., Vanha-Majamaa, I., Viiri, H., Vuorinen, M., and Ylioja, T.: Metsätuhot vuonna 2019 (in Finnish), Luonnonvara- ja biotalouden tutkimus 1/2022, Natural Resources Institute Finland, Helsinki, http://urn.fi/URN:ISBN:978-952-380-348-0 (last access: 19 November 2024), 2022.
NVE: Groundwater observations Norway, July 2018, https://www.senorge.no/ (last access: 15 November 2024), 2023.
NW-FVA: Waldzustandsbericht Niedersachsen 2022, https://www.ml.niedersachsen.de/download/190134/Waldzustandsbericht_Niedersachsen_ 2022.pdf (last access: 15 November 2024), 2022.
O'Hanlon, R., Ryan, C., Choiseul, J., Murchie, A. K., and Williams, C. D.: Catalogue of pests and pathogens of trees on the island of Ireland, Biol. Environ. Proc. Roy. Irish Acad., 121B, 21–45, https://doi.org/10.1353/bae.2021.0005, 2021.
Öhrn, P., Berlin, M., Elfstrand, M., Krokene, P., and Jönsson, A. M.: Seasonal variation in Norway spruce response to inoculation with bark beetle-associated bluestain fungi one year after a severe drought, Forest Ecol. Manag., 496, 119443, https://doi.org/10.1016/j.foreco.2021.119443, 2021.
Olefs, M., Formayer, H., Gobiet, A., Marke, T., Schöner, W., and Revesz, M.: Past and future changes of the Austrian climate–Importance for tourism, J. Outdoor Recreat. Tour., 34, 100395, https://doi.org/10.1016/j.jort.2021.100395, 2021.
ONF (Office National des Forêts): Forêts publiques françaises: quel nouveau visage?, https://www.onf.fr/onf/lonf-agit/+/8cf::forets-publiques-francaises-quel-nouveau-visage.html (last access: 15 November 2024), 2020.
ONF (Office National des Forêts): En forêt, la crise des scolytes s'accélère partout en France, https://www.onf.fr/onf/+/2e0::epidemie-de-scolytes-les-forestiers-de-lonf-sur-le-front.html (last access: 15 November 2024), 2021.
Orwig, D. A. and Abrams, M. D.: Variation in radial growth responses to drought among species, site, and canopy strata, Trees, 11, 474–484, https://doi.org/10.1007/s004680050114, 1997.
Patacca, M., Lindner, M., Lucas-Borja, M. E., Cordonnier, T., Fidej, G., Gardiner, B., and Schelhaas, M. J.: Significant increase in natural disturbance impacts on European forests since 1950, Glob. Change Biol., 29, 1359–1376, https://doi.org/10.1111/gcb.16578, 2023.
Peñuelas, J. and Filella, I.: Deuterium labelling of roots provides evidence of deep water access and hydraulic lift by Pinus nigra in a Mediterranean forest of NE Spain, Environ. Exp. Bot., 49, 201–208, https://doi.org/10.1016/S0098-8472(02)00070-9, 2003.
Pereira, M. G., Trigo, R. M., DaCamara, C. C., Pereira, J. M. C., and Leite, S. M.: Synoptic patterns associated with large summer forest fires in Portugal, Agr. Forest Meteorol., 129, 11–25, https://doi.org/10.1016/j.agrformet.2004.12.007, 2005.
Perugini, L., Caporaso, L., Marconi, S., Cescatti, A., Quesada, B., de Noblet-Ducoudré, N., and Arneth, A.: Biophysical effects on temperature and precipitation due to land cover change, Environ. Res. Lett., 12, 053002, https://doi.org/10.1088/1748-9326/aa6b3f, 2017.
Peters, W., Bastos, A., Ciais, P., and Vermeulen, A.: A historical, geographical and ecological perspective on the 2018 European summer drought, Philos. T. Roy. Soc. B, 375, 20190505, https://doi.org/10.1098/rstb.2019.0505, 2020.
Pettit, J. M., Voelker, S. L., DeRose, R. J., and Burton, J. I.: Spruce beetle outbreak was not driven by drought stress: Evidence from a tree-ring iso-demographic approach indicates temperatures were more important, Glob. Change Biol., 26, 5829–5843, https://doi.org/10.1111/gcb.15291, 2020.
Philipp, M., Wegmann, M., and Kübert-Flock, C.: Quantifying the Response of German Forests to Drought Events via Satellite Imagery, Remote Sens., 13, 1845, https://doi.org/10.3390/rs13091845, 2021.
Pilli, R., Vizzarri, M., and Chirici, G.: Combined effects of natural disturbances and management on forest carbon sequestration: the case of Vaia storm in Italy, Ann. For. Sci., 78, 1–18, https://doi.org/10.1007/s13595-021-01043-6, 2021.
Pirtskhalava-Karpova, N., Trubin, A., Karpov, A., and Jakuš, R.: Drought initialised bark beetle outbreak in Central Europe: Meteorological factors and infestation dynamic, Forest Ecol. Manag., 554, 121666, https://doi.org/10.1016/j.foreco.2023.121666, 2024.
Piton, B., Benest, F., Caroulle, F., Cuny, H., Gosselin, M., Montagné-Huck, C., Nicolas, M., and Rocquencourt, A.: État et évolution des forêts françaises métropolitaines, Synthèse des indicateurs de gestion durable 2020, https://foret.ign.fr/api/upload/IGD_2020_SYNTHESE.pdf (last access: 19 November 2024), 2021.
Plewa, R. and Mokrzycki, T.: Występowanie, biologia i znaczenie gospodarcze kornika ostrozębnego Ips acuminatus (Gyllenhal, 1827) (Coleoptera, Curculionidae, Scolytinae) w Polsce, Sylwan, 161, 619–629, https://doi.org/10.26202/sylwan.2017077, 2017.
Polish Supreme Chamber of Control: https://www.nik.gov.pl/aktualnosci/zapobieganie-suszy-rolniczej.html, last access: 27 June 2023.
Popkin, G.: Forest fight, Science, 374, 1184–1189, https://doi.org/10.1126/science.acx9733, 2021.
Prieto, I., Armas, C., and Pugnaire, F. I.: Water release through plant roots: new insights into its consequences at the plant and ecosystem level, New Phytol., 193, 830–841, 2012.
Przybyl, K.: Wpływ warunków klimatycznych na zamieranie dębów w Polsce oraz symptomy choroby, Arboretum Kornickie, 34, https://rcin.org.pl/Content/189871/KOR001_148916.pdf (last access: 28 November 2024), 1989 (in Polish).
Rabbel, I., Bogena, H., Neuwirth, B., and Diekkrüger, B.: Using sap flow data to parameterize the Feddes water stress model for Norway Spruce, Water-Sui, 10, https://doi.org/10.3390/w10030279, 2018.
RAF Italia 2017–2018: Rapporto sullo stato delle foreste e del settore forestale in Italia, Rapporto sullo stato delle foreste e del settore forestale in Veneto 2020, https://www.reterurale.it/flex/cm/pages/ServeBLOB.php/L/IT/IDPagina/19231 (last access: 15 November 2024), 2019.
Rakovec, O., Samaniego, L., Hari, V., Markonis, Y., Moravec, V., Thober, S., et al.: The 2018–2020 multi-year drought sets a new benchmark in Europe, Earth's Future, 10, e2021EF002394, https://doi.org/10.1029/2021EF002394, 2022.
REA: https://rea.ec.europa.eu/news/fighting-flames-eu-funded-projects-protecting-forests-fire-destruction-2024-07-23_en, last access: 21 August 2024.
Rechid, D., Davin, E., de Noblet-Ducoudré, N., and Katragkou, E.: CORDEX Flagship Pilot Study “LUCAS-Land Use & Climate Across Scales”-a new initiative on coordinated regional land use change and climate experiments for Europe, EGU General Assembly Conference Abstracts, 13172, Geophysical Research Abstracts, Vol. 19, EGU2017-13172, 23–28 April 2017, EGU General Assembly 2017, Vienna, Austria, 2017.
Ribeiro, A. F., Russo, A., Gouveia, C. M., and Pires, C. A.: Drought-related hot summers: a joint probability analysis in the Iberian Peninsula, Weather Climate Extremes, 30, 100279, https://doi.org/10.1016/j.wace.2020.100279, 2020.
Rico, L., Ogaya, R., Barbeta, A., and Peñuelas, J.: Changes in DNA methylation fingerprint of Quercus ilex trees in response to experimental field drought simulating projected climate change, Plant Biol., 16, 419–427, https://doi.org/10.1111/plb.12049, 2014.
Rita, A., Camarero, J. J., Nolè, A., Borghetti, M., Brunetti, M., Pergola, N., Serio, C., Vicente-Serrano, S. M., Tramutoli, V., and Ripullone, F.: The impact of drought spells on forests depends on site conditions: the case of 2017 summer heat wave in southern Europe, Glob. Change Biol., 26, 851–863, 2020.
Rotenberg, E. and Yakir, D.: Contribution of semi-arid forests to the climate system, Science, 327, 451–454, https://doi.org/10.1126/science.1180556, 2010.
Rousi, E., Selten, F., Rahmstorf, S., and Coumou, D.: Changes in North Atlantic Atmospheric Circulation in a Warmer Climate Favor Winter Flooding and Summer Drought over Europe, J. Climate, 34, 2277–2295, https://doi.org/10.1175/JCLI-D-20-0311.1, 2021.
Rousi, E., Kornhuber, K., Beobide-Arsuaga, G., Luo, F., and Coumou, D.: Accelerated western European heatwave trends linked to more-persistent double jets over Eurasia, Nat. Commun., 13, 31432, https://doi.org/10.1038/s41467-022-31432-y, 2022.
Rousi, E., Fink, A. H., Andersen, L. S., Becker, F. N., Beobide-Arsuaga, G., Breil, M., Cozzi, G., Heinke, J., Jach, L., Niermann, D., Petrovic, D., Richling, A., Riebold, J., Steidl, S., Suarez-Gutierrez, L., Tradowsky, J. S., Coumou, D., Düsterhus, A., Ellsäßer, F., Fragkoulidis, G., Gliksman, D., Handorf, D., Haustein, K., Kornhuber, K., Kunstmann, H., Pinto, J. G., Warrach-Sagi, K., and Xoplaki, E.: The extremely hot and dry 2018 summer in central and northern Europe from a multi-faceted weather and climate perspective, Nat. Hazards Earth Syst. Sci., 23, 1699–1718, https://doi.org/10.5194/nhess-23-1699-2023, 2023.
Ruffault, J., Curt, T., Moron, V., Trigo, R. M., Mouillot, F., Koutsias, N., and Dupuy, J. L.: Increased likelihood of heat-induced large wildfires in the Mediterranean Basin, Sci. Rep., 10, 13790, https://doi.org/10.1038/s41598-020-70069-z, 2020.
Rukh, S., Sanders, T. G. M., Krüger, I., Schad, T., and Bolte, A.: Distinct responses of European beech (Fagus sylvatica L.) to drought intensity and length: a review of the impacts of the 2003 and 2018–2019 drought events in Central Europe, Forests, 14, 248, https://doi.org/10.3390/f14020248, 2023.
Ruosteenoja, K., Markkanen, T., and Räisänen, J.: Thermal seasons in northern Europe in projected future climate, Int. J. Climatol., 40, 4444–4462, https://doi.org/10.1002/joc.6471, 2020.
Russo, A., Gouveia, C. M., Páscoa, P., DaCamara, C. C., Sousa, P. M., and Trigo, R. M.: Assessing the role of drought events on wildfires in the Iberian Peninsula, Agr. Forest Meteorol., 237, 50–59, https://doi.org/10.1016/j.agrformet.2017.01.021, 2017.
Saintonge, F.-X., Gillette, M., Blaser, S., Queloz, V., and Leroy, Q.: Situation et gestion de la crise liée aux scolytes de l'Épicéa commun fin 2021 dans l'est de la France, en Suisse et en Wallonie, Rev. For. Fr., 73, 619–641, https://doi.org/10.20870/revforfr.2021.7201, 2021.
Salomón, R. L., Peters, R. L., Zweifel, R., Sass-Klaassen, U. G. W., Stegehuis, A. I., Smiljanic, M., Poyatos, R., Babst, F., Cienciala, E., Fonti, P., Lerink, B. J. W., Lindner, M., Martinez-Vilalta, J., Mencuccini, M., Nabuurs, G.-J., van der Maaten, E., von Arx, G., Bär, A., Akhmetzyanov, L., Balanzategui, D., Bellan, M., Bendix, J., Berveiller, D., Blaženec, M., Čada, V., Carraro, V., Cecchini, S., Chan, T., Conedera, M., Delpierre, N., Delzon, S., Ditmarová, Ľ., Dolezal, J., Dufrêne, E., Edvardsson, J., Ehekircher, S., Forner, A., Frouz, J., Ganthaler, A., Gryc, V., Güney, A., Heinrich, I., Hentschel, R., Janda, P., Ježík, M., Kahle, H.-P., Knüsel, S., Krejza, J., Kuberski, Ł., Kučera, J., Lebourgeois, F., Mikoláš, M., Matula, R., Mayr, S., Oberhuber, W., Obojes, N., Osborne, B., Paljakka, T., Plichta, R., Rabbel, I., Rathgeber, C. B. K., Salmon, Y., Saunders, M., Scharnweber, T., Sitková, Z., Stangler, D. F., Stereńczak, K., Stojanović, M., Střelcová, K., Světlík, J., Svoboda, M., Tobin, B., Trotsiuk, V., Urban, J., Valladares, F., Vavrčík, H., Vejpustková, M., Walthert, L., Wilmking, M., Zin, E., Zou, J., and Steppe, K.: The 2018 European heatwave led to stem dehydration but not to consistent growth reductions in forests, Nat. Commun., 13, 31432, https://doi.org/10.1038/s41467-021-27579-9, 2022.
San-Miguel-Ayanz, J., Oom, D., Artes, T., Viegas, D. X., Fernandes, P., Faivre, N., and Castellnou, M.: Forest fires in Portugal in 2017, in: Science for Disaster Risk Management 2020: Acting Today, Protecting Tomorrow, edited by: Casajus Valles, A., Marin Ferrer, M., Poljanšek, K., and Clark, I., EUR 30183 EN, Publications Office of the European Union, Luxembourg, https://doi.org/10.2760/571085, 2020.
SCB: Marken i Sverige, https://www.scb.se/hitta-statistik/sverige-i-siffror/miljo/marken-i-sverige/ (last access: 21 November 2024), 2020.
Scherrer, D., Ascoli, D., Conedera, M., Fischer, C., Maringer, J., Moser, B., Wohlgemuth, T. et al.: Canopy disturbances catalyse tree species shifts in Swiss forests, Ecosystems, 25, 199–214, https://doi.org/10.1007/s10021-021-00649-1, 2022.
Schnabel, F., Purrucker, S., Schmitt, L., Engelmann, R. A., Kahl, A., Richter, R., Seele-Dilbat, C., Skiadaresis, G., and Wirth, C.: Cumulative growth and stress responses to the 2018–2019 drought in a European floodplain forest, Glob. Change Biol., 28, 1870–1883, https://doi.org/10.1111/gcb.16028, 2021.
Schreiner Zeitung: https://www.schreinerzeitung.ch/de/artikel/zweithochster-je-registrierter-borkenkaferbefall-der-schweiz (last access: 18 November 2024), 2020.
Schuldt, B., Knutzen, F., Delzon, S., Jansen, S., Müller-Haubold, H., Burlett, R., Clough, Y., and Leuschner, C.: How adaptable is the hydraulic system of European beech in the face of climate change-related precipitation reduction?, New Phytol., 210, 443–458, https://doi.org/10.1111/nph.13798, 2016.
Schuldt, B., Buras, A., Arend, M., Vitasse, Y., Beierkuhnlein, C., Damm, A., Gharun, M., Grams, T. E. E., Hauck, M., Hajek, P., Hartmann, H., Hiltbrunner, E., Hoch, G., Holloway-Phillips, M., Körner, C., Larysch, E., Lübbe, T., Nelson, D. B., Rammig, A., Rigling, A., Rose, L., Ruehr, N. K., Schumann, K., Weiser, F., Werner, C., Wohlgemuth, T., Zang, C. S., and Kahmen, A.: A first assessment of the impact of the extreme 2018 summer drought on Central European forests, Basic Appl. Ecol., 45, 86–103, https://doi.org/10.1016/j.baae.2020.04.003, 2020.
Schumacher, D. L., Zachariah, M., Otto, F., Barnes, C., Philip, S., Kew, S., Vahlberg, M., Singh, R., Heinrich, D., Arrighi, J., van Aalst, M., Thalheimer, L., Raju, E., Hauser, M., Hirschi, M., Gudmundsson, L., Beaudoing, H. K., Rodell, M., Li, S., Yang, W., Vecchi, G. A., Vautard, R., Harrington, L. J., and Seneviratne, S. I.: High temperatures exacerbated by climate change made 2022 Northern Hemisphere soil moisture droughts more likely, World Weather Attribution, United Kingdom, https://coilink.org/20.500.12592/f00cjq (last access: 9 December 2024), 2022.
Schumacher, D. L., Zachariah, M., Otto, F., Barnes, C., Philip, S., Kew, S., Vahlberg, M., Singh, R., Heinrich, D., Arrighi, J., van Aalst, M., Hauser, M., Hirschi, M., Bessenbacher, V., Gudmundsson, L., Beaudoing, H. K., Rodell, M., Li, S., Yang, W., Vecchi, G. A., Harrington, L. J., Lehner, F., Balsamo, G., and Seneviratne, S. I.: Detecting the human fingerprint in the summer 2022 western–central European soil drought, Earth Syst. Dynam., 15, 131–154, https://doi.org/10.5194/esd-15-131-2024, 2024.
Scottish Forestry: https://www.forestry.gov.scot/sustainable-forestry/tree-health/tree-pests-and-diseases/great-spruce-bark-beetle-in-scotland (last access: 18 November 2024), 2023a.
Scottish Forestry: https://www.forestry.gov.scot/publications/1385-updated-d-micans-distribution-map-in-scotland-january-2022/download (last access: 18 November 2024), 2023b.
Seaton, F. M., Jones, D. L., Creer, S., George, P. B. L., Smart, S. M., Lebron, I., Barrett, G., Emmett, B. A., and Robinson, D. A.: Plant and soil communities are associated with the response of soil water repellency to environmental stress, Sci. Total Environ., 687, 929–938, https://doi.org/10.1016/j.scitotenv.2019.06.050, 2019.
Sefton, C., Muchan, K., Parry, S., Matthews, B., Barker, L. J., Turner, S., and Hannaford, J.: The 2019/2020 floods in the UK: a hydrological appraisal, Weather, https://doi.org/10.1002/wea.3993, 2021.
Seidl, R., Schelhaas, M. J., Rammer, W., et al.: Increasing forest disturbances in Europe and their impact on carbon storage, Nat. Clim. Change, 4, 806–810, https://doi.org/10.1038/nclimate2318, 2014.
Seidl, R., Thom, D., Kautz, M., Martin-Benito, D., Peltoniemi, M., Vacchiano, G., Wild, J., Ascoli, D., Petr, M., Honkaniemi, J., Lexer, M. J., Trotsiuk, V., Mairota, P., Svoboda, M., Fabrika, M., Nagel, T. A., and Reyer, C. P. O.: Forest disturbances under climate change, Nat. Clim. Chang., 7, 395–402, https://doi.org/10.1038/nclimate3303, 2017.
Seidl, R., Honkaniemi, J., Aakala, T., Aleinikov, A., Angelstam, P., Bouchard, M., Boulanger, Y., Burton, P. J., De Grandpré, L., Gauthier, S., Hansen, W. D., Jepsen, J. U., Jõgiste, K., Kneeshaw, D. D., Kuuluvainen, T., Lisitsyna, O., Makoto, K., Mori, A. S., Pureswaran, D. S., Shorohova, E., Shubnitsina, E., Taylor, A. R., Vladimirova, N., Vodde, F., and Senf, C.: Globally consistent climate sensitivity of natural disturbances across boreal and temperate forest ecosystems, Ecography, 43, 967–978, https://doi.org/10.1111/ecog.04995, 2020.
Senf, C. and Seidl, R.: Persistent impacts of the 2018 drought on forest disturbance regimes in Europe, Biogeosciences, 18, 5223–5230, https://doi.org/10.5194/bg-18-5223-2021, 2021a.
Senf, C. and Seidl, R.: Storm and fire disturbances in Europe: Distribution and trends, Glob. Change Biol., 27, 3605–3619, 2021b.
Seneviratne, S. I., Zhang, X., Adnan, M., Badi, W., Dereczynski, C., Di Luca, A., Ghosh, S., Iskandar, I., Kossin, J., Lewis, S., Otto, F., Pinto, I., Satoh, M., Vicente-Serrano, S. M., Wehner, M., and Zhou, B.: Chapter 11: Weather and climate extreme events in a changing climate, 2021.
Sierota, Z., Grodzki, W., and Szczepkowski, A.: Abiotic and Biotic Disturbances Affecting Forest Health in Poland over the Past 30 Years: Impacts of Climate and Forest Management, Forests, 10, 75, https://doi.org/10.3390/f10010075, 2019.
Sierota, Z. and Grodzki, W.: Picea abies-Armillaria-Ips: A strategy or coincidence?, Forests, 11, 1023, https://doi.org/10.3390/F11091023, 2020.
Sioen, G., Verschelde, P., and Roskams, P.: Bosvitaliteitsinventaris 2018. Resultaten uit het bosvitaliteitsmeetnet (Level 1), Rapports van het Instituut voor Natuur- en Bosonderzoek, Nr. 20, Instituut voor Natuur- en Bosonderzoek, https://doi.org/10.21436/inbor.16207115, 2019.
Sioen, G., Verschelde, P., and Roskams, P.: Bosvitaliteitsinventaris 2019: Resultaten uit het bosvitaliteitsmeetnet (Level 1), Rapports van het Instituut voor Natuur- en Bosonderzoek, Nr. 20, Instituut voor Natuur- en Bosonderzoek, https://doi.org/10.21436/inbor.18050253, 2020.
Sioen, G., Verschelde, P., and Roskams, P.: Bosvitaliteitsinventaris 2020: Resultaten uit het bosvitaliteitsmeetnet (Level 1), Rapports van het Instituut voor Natuur- en Bosonderzoek, Nr. 20, Instituut voor Natuur- en Bosonderzoek, https://doi.org/10.21436/inbor.34283136, 2021.
Sioen, G., Verschelde, P., and Roskams, P.: Bosvitaliteitsinventaris 2021. Resultaten uit het bosvitaliteitsmeetnet (Level 1), Rapports van het Instituut voor Natuur- en Bosonderzoek, Nr. 7, Instituut voor Natuur- en Bosonderzoek, https://doi.org/10.21436/inbor.71783042, 2022.
Sioen, G., Verschelde, P., and Roskams, P.: Bosvitaliteitsinventaris 2022. Resultaten uit het bosvitaliteitsmeetnet (Level 1), Rapports van het Instituut voor Natuur- en Bosonderzoek, Nr. 4, https://doi.org/10.21436/inbor.90109478, 2023.
Sire, L., Schmidt Yáñez, P., Wang, C., Bézier, A., Courtial, B., Cours, J., Fontaneto, D., Larrieu, L., Bouget, C., Thorn, S., Müller, J., Yu, D. W., Monaghan, M. T., Herniou, E. A., and Lopez-Vaamonde, C.: Climate-induced forest dieback drives compositional changes in insect communities that are more pronounced for rare species, Commun. Biol., 5, 57, https://doi.org/10.1038/s42003-021-02968-4, 2022.
Skrzecz, I., Tkaczyk, M., and Oszako, T.: Current Problems of Forest Protection (25–27 October 2022, Katowice Poland), Appl. Sci., 12, 12745, https://doi.org/10.3390/app122412745, 2022.
SMHI: Året 2022. Mycket tört i sydöstra Sverige, https://www.smhi.se/klimat/klimatet-da-och-nu/arets-vader/aret-2022-mycket-torrt-i-sydostra-sverige-1.190565 (last access: 15 November 2024), 2023.
Sofiadis, G., Katragkou, E., Davin, E. L., Rechid, D., de Noblet-Ducoudre, N., Breil, M., Cardoso, R. M., Hoffmann, P., Jach, L., Meier, R., Mooney, P. A., Soares, P. M. M., Strada, S., Tölle, M. H., and Warrach Sagi, K.: Afforestation impact on soil temperature in regional climate model simulations over Europe, Geosci. Model Dev., 15, 595–616, https://doi.org/10.5194/gmd-15-595-2022, 2022.
Spinoni, J., Vogt, J. V., Naumann, G., Barbosa, P., and Dosio, A.: Will drought events become more frequent and severe in Europe?, Int. J. Climatol., 38, 1718–1736, 2018.
SSB: Landskogtakseringen (forest volume statistics Norway), https://www.ssb.no/statbank/table/06289/tableViewLayout1/ (last access: 15 November 2024), 2022.
Stagge, J. H., Kingston, D. G., Tallaksen, L. M., and Hannah, D. M.: Observed drought indices show increasing divergence across Europe, Sci. Rep., 7, 1–10, https://doi.org/10.1038/s41598-017-14283-2, 2017.
Statsforvalteren: Årsmelding 2019. Skogbruket i Vestfold og Telemark, https://www.statsforvalteren.no/siteassets/fm-vestfold-og-telemark/landbruk-og-mat/skogbruk/arsmelding-2020-hele-med-logo.pdf (last access: 27 November 2024), 2020.
Statsforvalteren: Årsmelding 2020. Skogbruket i Vestfold og Telemark, https://www.statsforvalteren.no/contentassets/73e08d3b324a45db9077831c5fa2c04b/skogbruket-i-vestfold-og-telemark-2021.pdf (last access: 27 November 2024), 2021.
Stelzl, A., Pointl, M., and Fuchs-Hanusch, D.: Estimating Future Peak Water Demand with a Regression Model Considering Climate Indices, Water, 13, 1912, https://doi.org/10.3390/w13141912, 2021.
Stephan, R., Erfurt, M., Terzi, S., Žun, M., Kristan, B., Haslinger, K., and Stahl, K.: An inventory of Alpine drought impact reports to explore past droughts in a mountain region, Nat. Hazards Earth Syst. Sci., 21, 2485–2501, https://doi.org/10.5194/nhess-21-2485-2021, 2021.
Středová, H., Fukalová, P., Chuchma, F., and Středa, T.: A complex method for estimation of multiple abiotic hazards in forest ecosystems, Water, 12, 2872, https://doi.org/10.3390/w12102872, 2020.
Sturm, J., Santos, M. J., Schmid, B., and Damm, A.: Satellite data reveal differential responses of Swiss forests to unprecedented 2018 drought, Glob. Chang. Biol., 28, 2956–2978, https://doi.org/10.1111/gcb.16194, 2022.
Suarez-Gutierrez, L., Li, C., Müller, W. A., and Marotzke, J.: Internal variability in European summer temperatures at 1.5 °C and 2 °C of global warming, Environ. Res. Lett., 13, 064026, https://doi.org/10.1088/1748-9326/aaba58, 2018.
Suarez-Gutierrez, L., Müller, W. A., and Marotzke, J.: Extreme heat and drought typical of an end-of-century climate could occur over Europe soon and repeatedly, Commun. Earth Environ., 4, 415, https://doi.org/10.1038/s43247-023-01075-y, 2023.
Süßel, F. and Brüggemann, W.: Tree water relations of mature oaks in southwest Germany under extreme drought stress in summer 2018, Plant Stress, 1, 100010, https://doi.org/10.1016/j.stress.2021.100010, 2021.
Sommerfeld, A., Rammer, W., Heurich, M., Hilmers, T., Müller, J., and Seidl, R.: Do bark beetle outbreaks amplify or dampen future bark beetle disturbances in Central Europe?, J. Ecol., 109, 737–749, 2021.
SRF: https://www.srf.ch/news/schweiz/der-schweizer-wald-leidet-extremer-befall-von-borkenkaefern (last access: 15 November 2024), 2020.
Susza: Data from official governamental page, compared with other European Countries, https://www.gov.pl/web/susza/susza (last access: 28 November 2024), 2023.
Swedish Board of Agriculture: Långsiktiga effekter av torkan 2018, och hur jordbruket kan bli mer motståndskraftigt mot extremväder, Jorbruksverkets rapport RA 19/13, https://webbutiken.jordbruksverket.se/sv/artiklar/ra1913.html (last access: 19 November 2024), 2019.
SZ: https://www.sueddeutsche.de/bayern/schloss-neuschwanstein-allgaeu-waldbrand-1.5547353 (last access: 15 November 2024), 2022.
Tagesschau: https://www.rainews.it/tgr/tagesschau/articoli/2022/10/ tag-Borkenkaefer-5000-Hektar-in-Suedtirol-befallen-e7437c6e-2139-4b00-87d4-bf6d265595e9.html (last access: 15 November 2024), 2022.
Telegraph: https://www.telegraph.co.uk/news/fire-storm-the-wildfires-sweeping-europe-and-britain/ (last access: on 14 March 2023), 2018.
Terhonen, E., Melin, M., Aarnio, L., Granberg, F., Hantula, J., Henttonen, H., Huitu, O., Huuskonen, S., Härkönen, M., Kaitera, J., Koivula, M., Kokko, A., Kokkonen, J., Korhonen, K. T., Laurila, I., Lehto, T., Luoranen, J., Niemimaa, J., Nuorteva, H., Pennanen, T., Piri, T., Poimala, A., Pouttu, A., Pätäri, V., Siitonen, J., Silver, T., Strandström, M., Sutela, S., Tikkanen, O.-P., Vainio, E., Vanha-Majamaa, I., Velmala, S., and Ylioja, T.: Metsätuhot vuonna 2022 (in Finnish), Luonnonvara- ja biotalouden tutkimus 48/2023, Natural Resources Institute Finland, Helsinki, http://urn.fi/URN:ISBN:978-952-380-694-8 (last access: 19 November 2024), 2023.
Teuling, A. J., Seneviratne, S. I., Stöckli, R., Reichstein, M., Moors, E., Ciais, P., Luyssaert, S., van den Hurk, B., Ammann, C., Bernhofer, C., Dellwik, E., Gianelle, D., Gielen, B., Grünwald, T., Klumpp, K., Montagnani, L., Moureaux, C., Sottocornola, M., and Wohlfahrt, G.: Contrasting response of European forest and grassland energy exchange to heatwaves, Nat. Geosci., 3, 722–727, 2010.
Teutschbein, C., Jonsson, E., Todorović, A., Tootoonchi, F., Stenfors, E., and Grabs, T.: Drought Propagation through the Water-Energy-Food-Ecosystem Nexus: a Nordic Perspective, https://doi.org/10.1016/j.jhydrol.2022.128963, 2022a.
Teutschbein, C., Montano, B. Q., Todorović, A., and Grabs, T.: Streamflow droughts in Sweden: Spatiotemporal patterns emerging from six decades of observations, J. Hydrol. Regional Stud., 42, 101171, https://doi.org/10.1016/j.ejrh.2022.101171, 2022b.
The Herald: Scotland escaped global 2021 wildfire crisis so far, https://www.heraldscotland.com/news/19482198.scotland-escaped-global-2021-wildfire-crisis-far/ (last access: 14 March 2023), 2021.
Thonfeld, F., Gessner, U., Holzwarth, S., Kriese, J., da Ponte, E., Huth, J., and Kuenzer, C.: A First Assessment of Canopy Cover Loss in Germany's Forests after the 2018–2020 Drought Years, Remote Sens., 14, 562, https://doi.org/10.3390/rs14030562, 2022.
Thom, D. and Seidl, R.: Accelerating Mountain Forest Dynamics in the Alps, Ecosystems, 25, 603–617, https://doi.org/10.1007/s10021-021-00674-0, 2022.
Thom, D., Rammer, W., Laux, P., Smiatek, G., Kunstmann, H., Seibold, S., and Seidl, R.: Will forest dynamics continue to accelerate throughout the 21st century in the Northern Alps?, https://doi.org/10.1111/gcb.16133, 2022.
Thrippleton, T., Lüscher, F., and Bugmann, H.: Climate change impacts across a large forest enterprise in the Northern Pre-Alps: dynamic forest modelling as a tool for decision support, Eur. J. Forest Res., 139, 483–498, 2020.
TMIL: https://infrastruktur-landwirtschaft.thueringen.de/fileadmin/Forst_und_Jagd_Fischerei/Forstwirtschaft/2022_Waldzustandsbericht_barrierefrei.pdf (last access: 15 November 2024), 2022.
Toreti, A., Bavera, D., Acosta Navarro, J., Cammalleri, C., de Jager, A., Di Ciollo, C., Hrast Essenfelder, A., Maetens, W., Magni, D., Masante, D., Mazzeschi, M., Niemeyer, S., and Spinoni, J.: Drought in Europe August 2022, Publications Office of the European Union, Luxembourg, JRC13049, https://doi.org/10.2760/264241, 2022a.
Toreti, A., Bavera, D., Avanzi, F., Cammalleri, C., De Felice, M., de Jager, A., Di Ciollo, C., Gabellani, S., Maetens, W., Magni, D., Manfron, G., Masante, D., Mazzeschi, M., McCormick, N., Naumann, G., Niemeyer, S., Rossi, L., Seguini, L., Spinoni, J., and van den Berg, M.: Drought in northern Italy March 2022, EUR 31037 EN, Publications Office of the European Union, Luxembourg, JRC128974, https://doi.org/10.2760/781876, 2022b.
Toreti, A., Bavera, D., Acosta Navarro, J., Arias-Muñoz, C., Avanzi, F., Marinho Ferreira Barbosa, P., De Jager, A., Di Ciollo, C., Ferraris, L., Fioravanti, G., Gabellani, S., Grimaldi, S., Hrast Essenfelder, A., Isabellon, M., Jonas, T., Maetens, W., Magni, D., Masante, D., Mazzeschi, M., McCormick, N., Meroni, M., Rossi, L., Salamon, P., and Spinoni, J.: Drought in Europe March 2023, EUR 31448 EN, Publications Office of the European Union, Luxembourg, JRC133025, https://doi.org/10.2760/998985, 2023.
Toth, D., Maitah, M., Maitah, K., and Jarolínová, V.: The impacts of calamity logging on the development of spruce wood prices in Czech forestry, Forests, 11, 283, https://doi.org/10.3390/f11030283, 2020.
Turco, M., Jerez, S., Augusto, S., Tarín-Carrasco, P., Ratola, N., Jiménez-Guerrero, P., and Trigo, R. M.: Climate drivers of the 2017 devastating fires in Portugal, Sci. Rep., 9, 13886, https://doi.org/10.1038/s41598-019-50281-2, 2019.
Turco, M., Abatzoglou, J. T., Herrera, S., Zhuang, Y., Jerez, S., Lucas, D. D., AghaKouchak, A., and Cvijanovic, I.: Anthropogenic climate change impacts exacerbate summer forest fires in California, Proc. Natl. Acad. Sci., 120, e2213815120, https://doi.org/10.1073/pnas.2213815120, 2023.
Turner, S., Barker, L. J., Hannaford, J., Muchan, K., Parry, S., and Sefton, C.: The 2018/2019 drought in the UK: a hydrological appraisal, Weather, 76, 248–253, 2021.
Tyukavina, A., Potapov, P., Hansen, M. C., Pickens, A. H., Stehman, S. V., Turubanova, S., Parker, D., Zalles, V., Lima, A., Kommareddy, I., Song, X.-P., Wang, L., and Harris, N.: Global trends of forest loss due to fire from 2001 to 2019, Front. Remote Sens., 3, 825190, https://doi.org/10.3389/frsen.2022.825190, 2022.
UBA: Waldbrände in Deutschland, Umweltbundesamt, https://www.umweltbundesamt.de/daten/land-forstwirtschaft/waldbraende#waldbrande-in-deutschland (last access: 15 November 2024), 2023a.
UBA: Das Monitoringbericht 2023, Umweltbundesamt, https://www.umweltbundesamt.de/sites/default/files/medien/376/publikationen/das-monitoringbericht_2023_bf_korr.pdf (last access: 19 August 2023), 2023b.
Ukkola, A. M., De Kauwe, M. G., Roderick, M. L., Abramowitz, G., and Pitman, A. J.: Robust future changes in meteorological drought in CMIP6 projections despite uncertainty in precipitation, Geophys. Res. Lett., 47, e2020GL087820, https://doi.org/10.1029/2020GL087820, 2020.
UN: Member States, United Nations, https://www.un.org/en/about-us/member-states (last access: 9 February 2024), 2024.
UNECE Committee on Forest and the Forest Industry and the European Forestry Commission (Foresta2022): Sweden: Country market statement 2022, UNECE, https://unece.org/forestry-timber/documents/2022/10/informal-documents/sweden-country-market-statement-2022 (last access 1 March 2023), 2022.
Van Der Wiel, K., Batelaan, T. J., and Wanders, N.: Large increases of multi-year droughts in north-western Europe in a warmer climate, Clim. Dyn., 59, 1–20, https://doi.org/10.1007/S00382-022-06373-3, 2022.
Van Loon, A. F.: Hydrological drought explained, WIREs Water, 2, 359–392, 2015.
Veijalainen, N., Ahopelto, L., Marttunen, M., Jääskeläinen, J., Britschgi, R., Orvomaa, M., Belinskij, A., and Keskinen, M.: Severe drought in Finland: Modeling effects on water resources and assessing climate change impacts, Sustainability, 11, 2450, https://doi.org/10.3390/su11082450, 2019.
Venäläinen, A., Lehtonen, I., and Mäkelä, A.: Laaja-alaisia metsäpaloja mahdollistavat säätilanteet Suomen ilmastossa [The risk of large forest fires in Finland], Finnish Meteorological Institute, Reports 2016:3 (in Finnish, English Abstract), https://helda.helsinki.fi/handle/10138/161478 (last access: 15 November 2024), 2016.
Verkerk, P. J., Delacote, P., Hurmekoski, E., Kunttu, J., Matthews, R., Mäkipää, R., Mosley, F., Perugini, L., Reyer, C. P. O., Roe, S., and Trømborg, E.: Forest-based climate change mitigation and adaptation in Europe. From Science to Policy, 14, European Forest Institute, https://doi.org/10.36333/fs14, 2022.
Vicedo-Cabrera, A. M., Scovronick, N., Sera, F., Royé, D., Schneider, R., Tobias, A., Astrom, C., Guo, Y., Honda, Y., Hondula, D. M., Abrutzky, R., Tong, S., Coelho, M., de Sousa Zanotti Stagliorio, P. H., Nascimento Saldiva, E., Lavigne, P., Matus Correa, N. Valdes Ortega, H. Kan, S. Osorio, Kyselý, J., Urban, A., Orru, H., Indermitte, E., Jaakkola, J. J. K., Ryti, N., Pascal, M., Schneider, A., Katsouyanni, K., Samoli, E., Mayvaneh, F., Entezari, A., Goodman, P., Zeka, A., Michelozzi, P., de’Donato, F., Hashizume, M., Alahmad, B., Hurtado Diaz, M., De La Cruz Valencia, C., Overcenco, A., Houthuijs, D., Ameling, C., Rao, S., Di Ruscio, F., Carrasco-Escobar, G., Seposo, X., Silva, S., Madureira, J., Holobaca, I. H., Fratianni, S., Acquaotta, F., Kim, H., Lee, W., Iniguez, C., Forsberg, B., Ragettli, M. S., Guo, Y. L. L., Chen, B. Y., Li, S., Armstrong, B., Aleman, A., Zanobetti, A., Schwartz, J., Dang, T. N., Dung, D. V., Gillett, N., Haines, A., Mengel, M., Huber, V., Gasparrini, A.: The burden of heat-related mortality attributable to recent human-induced climate change, Nat. Clim. Change, 11, 492–500, 2021.
Vicente-Serrano, S. M., Beguería, S., Lorenzo-Lacruz, J., Camarero, J. J., López-Moreno, J. I., Azorin-Molina, C., Revuelto, J., Morán-Tejeda, E., and Sanchez-Lorenzo, A.: Performance of drought indices for ecological, agricultural, and hydrological applications, Earth Interact., 16, 1–27, 2012.
Vicente-Serrano, S. M., Gouveia, C., Camarero, J. J., Beguería, S., Trigo, R., López-Moreno, J. I., Azorín-Molina, C., Pasho, E., and Lorenzo-Lacruz, J., Revuelto, J., Morán-Tejeda, E., and Sanchez-Lorenzo, A.: The response of vegetation to drought time-scales across global land biomes, P. Natl. Acad. Sci. USA., 110, 52–57, 2013.
Vicente-Serrano, S. M., Quiring, S. M., Pena-Gallardo, M., Yuan, S., and Dominguez-Castro, F.: A review of environmental droughts: Increased risk under global warming?, Earth-Sci. Rev., 201, 102953, https://doi.org/10.1016/j.earscirev.2019.102953, 2020.
Vogel, M. M., Zscheischler, J., Wartenburger, R., Dee, D., and Seneviratne, S. I.: Concurrent 2018 hot extremes across Northern Hemisphere due to human-induced climate change, Earth's Future, 7, 692–703, 2019.
Vogel, J., Paton, E., Aich, V., and Bronstert, A.: Increasing compound warm spells and droughts in the Mediterranean Basin, Weather Clim. Extremes, 32, 100312, https://doi.org/10.1016/j.wace.2021.100312, 2021.
Waldschutz: Leichte Zunahme von Buchdrucker-Befallsherden, https://www.dora.lib4ri.ch/wsl/islandora/object/wsl:32875/datastream/PDF/Stroheker-2023-Leichte_Zunahme_von_Buchdrucker-Befallsherden-(published_version).pdf (last access: 15 November 2024), 2023.
Wataha: An increase in the bark beetle population in Norwegian forests, Wataha, https://wataha.no/en/2021/11/15/an-increase-in-the-bark-beetle-population-in-norwegian-forests/ (last access: 21 August 2024), 2021.
Watts, N., Amann, M., Arnell, N., Ayeb-Karlsson, S., Beagley, J., Belesova, K., Boykoff, M., Byass, P., Cai, W., Campbell-Lendrum, D., Capstick, S., Chambers, J., Coleman, S., Dalin, C., Daly, M., Dasandi, N., Dasgupta, S., Davies, M., Di Napoli, C., Dominguez-Salas, P., Drummond, P., Dubrow, R., Ebi, K. L., Eckelman, M., Ekins, P., Escobar, L. E., Georgeson, L., Golder, S., Grace, D., Graham, H., Haggar, P., Hamilton, I., Hartinger, S., Hess, J., Hsu, S.-C., Hughes, N., Jankin Mikhaylov, S., Jimenez, M. P., Kelman, I., Kennard, H., Kiesewetter, G., Kinney, P. L., Kjellstrom, T., Kniveton, D., Lampard, P., Lemke, B., Liu, Y., Liu, Z., Lott, M., Lowe, R., Martinez-Urtaza, J., Maslin, M., McAllister, L., McGushin, A., McMichael, C., Milner, J., Moradi-Lakeh, M., Morrissey, K., Munzert, S., Murray, K. A., Neville, T., Nilsson, M., Odhiambo Sewe, M., Oreszczyn, T., Otto, M., Owfi, F., Pearman, O., Pencheon, D., Quinn, R., Rabbaniha, M., Robinson, E., Rocklöv, J., Romanello, M., Semenza, J. C., Sherman, J., Shi, L., Springmann, M., Tabatabaei, M., Taylor, J., Triñanes, J., Shumake-Guillemot, J., Vu, B., Wilkinson, P., Winning, M., Gong, P., Montgomery, H., and Costello, A.: The 2020 report of The Lancet Countdown on health and climate change: Responding to converging crises, Lancet, 397, 129–170, 2021.
Wawrzoniak, J. (Ed.), Boczoń, A., Hildebrand, R., Kowalska, A., Lech, P., Małachowska, J., Wawrzoniak, J., and Zajączkowski, G.: Stan zdrowotny lasów Polski w 2018 roku, Instytut Badawczy Lesnictwa, Sękocin Stary, http://www.gios.gov.pl/monlas/raporty/raport_2018/raport_2018.pdf (last access: 28 November 2024), 2019 (in Polish).
Weigel, R., Bat-Enerel, B., Dulamsuren, C., Muffler, L., Weithmann, G., and Leuschner, C.: Summer drought exposure, stand structure, and soil properties jointly control the growth of European beech along a steep precipitation gradient in northern Germany, Glob. Change Biol., 29, 763–779, 2023.
WSL: Trockenheit 2018: Buchen mit frühzeitig verfärbtem Laub neigen zum Absterben in den Folgejahren, WSL, https://www.wsl.ch/de/news/trockenheit-2018-buchen-mit-fruehzeitig-verfaerbtem-laub-neigen-zum-absterben-in-den-folgejahren/ (last access: 20 November 2024), 2022.
WSL: Leichte Zunahme des Borkenkäfer-Befalls im Jahr 2022, WSL, https://www.wsl.ch/de/news/leichte-zunahme-des-borkenkaefer-befalls-im-jahr-2022/#:~:text=Ausblick,überdurchschnittlich warm und trocken bleibt (last access: 15 November 2024), 2023a.
WSL: Zwischenberichte, WSL, https://www.waldwissen.net/assets/technik/inventur/wsl_zwischenergebnisse-lfi5/Zwischenergebnisse_LFI_print.pdf#page=2 (last access: 15 November 2024), 2023b.
Winkel, G., Lovrić, M., Muys, B., Katila, P., Lundhede, T., Pecurul, M., Pettenella, D., Pipart, N., Plieninger, T., Prokofieva, I., Parra, C., Pülzl, H., Roitsch, D., Roux, J.-L., Thorsen, B. J., Tyrväinen, L., Torralba, M., Vacik, H., Weiss, G., and Wunder, S.: Governing Europe's forests for multiple ecosystem services: Opportunities, challenges, and policy options, For. Policy Econ., 145, 102849, https://doi.org/10.1016/j.forpol.2022.102849, 2022.
Zahradník, P. and Zahradníková, M.: Salvage felling in the Czech Republic`s forests during the last twenty years, Cent. Eur. For. J., 65, 12–20, 2019.
Winland-project Policy Brief VII: Kuivuus koettelee myös Suomea – Olemmeko tarpeeksi varautuneita?, ISBN 978-952-60-3774-5 (PDF), https://winlandtutkimus.fi/wp-content/uploads/2019/04/Winland-kuivuus.pdf (last access: 2 June 2022), 2019 (in Finnish).
Worlds Aid: Worlds Aid, https://www.worldsaid.com/node/1378 (last access: 15 November 2024), 2022.
Wulff, S. and Roberge, J.-M.: Forest monitoring: Synthesis of 2010–2018 results, SLU, https://pub.epsilon.slu.se/21827/1/wulff_s_et_al_210201.pdf (last access: 15 November 2024), 2020.
Yu, G. R., Zhuang, J., Nakayama, K., and Jin, Y.: Root water uptake and profile soil water as affected by vertical root distribution, Plant Ecol., 189, 15–30, 2007.
Yu, Z., Wang, J., Liu, S., Rentch, J. S., Sun, P., and Lu, C.: Global gross primary productivity and water use efficiency changes under drought stress, Environ. Res. Lett., 12, 014016, https://doi.org/10.1088/1748-9326/aa5258, 2017.
Xoplaki, E., Ellsäßer, F., Grieger, J., Nissen, K. M., Pinto, J., Augenstein, M., Chen, T.-C., Feldmann, H., Friederichs, P., Gliksman, D., Goulier, L., Haustein, K., Heinke, J., Jach, L., Knutzen, F., Kollet, S., Luterbacher, J., Luther, N., Mohr, S., Mudersbach, C., Müller, C., Rousi, E., Simon, F., Suarez-Gutierrez, L., Szemkus, S., Vallejo-Bernal, S. M., Vlachopoulos, O., and Wolf, F.: Compound events in Germany in 2018: drivers and case studies, EGUsphere [preprint], https://doi.org/10.5194/egusphere-2023-1460, 2023.
Zas, R., Touza, R., Sampedro, L., Lario, F. J., Bustingorri, G., and Lema, M.: Variation in resin flow among Maritime pine populations: Relationship with growth potential and climatic responses, For. Ecol. Manag., 474, 118351, https://doi.org/10.1016/j.foreco.2020.118351, 2020.
Zscheischler, J., Martius, O., Westra, S., Bevacqua, E., Raymond, C., Horton, R. M., van den Hurk, B., AghaKouchak, A., Jézéquel, A., Mahecha, M. D., Maraun, D., Ramos, A. M., Ridder, N. N., Thiery, W., and Vignotto, E.: A typology of compound weather and climate events, Nat. Rev. Earth Environ., 1, 333–347, 2020.