the Creative Commons Attribution 4.0 License.
the Creative Commons Attribution 4.0 License.
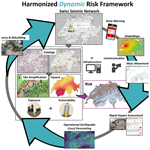
Towards a dynamic earthquake risk framework for Switzerland
Maren Böse
Laurentiu Danciu
Athanasios Papadopoulos
John Clinton
Carlo Cauzzi
Irina Dallo
Leila Mizrahi
Tobias Diehl
Paolo Bergamo
Yves Reuland
Andreas Fichtner
Philippe Roth
Florian Haslinger
Frédérick Massin
Nadja Valenzuela
Nikola Blagojević
Lukas Bodenmann
Eleni Chatzi
Donat Fäh
Franziska Glueer
Marta Han
Lukas Heiniger
Paulina Janusz
Dario Jozinović
Philipp Kästli
Federica Lanza
Timothy Lee
Panagiotis Martakis
Michèle Marti
Men-Andrin Meier
Banu Mena Cabrera
Maria Mesimeri
Anne Obermann
Pilar Sanchez-Pastor
Luca Scarabello
Nicolas Schmid
Anastasiia Shynkarenko
Bozidar Stojadinović
Domenico Giardini
Stefan Wiemer
Scientists from different disciplines at ETH Zurich are developing a dynamic, harmonised, and user-centred earthquake risk framework for Switzerland, relying on a continuously evolving earthquake catalogue generated by the Swiss Seismological Service (SED) using the national seismic networks. This framework uses all available information to assess seismic risk at various stages and facilitates widespread dissemination and communication of the resulting information. Earthquake risk products and services include operational earthquake (loss) forecasting (OE(L)F), earthquake early warning (EEW), ShakeMaps, rapid impact assessment (RIA), structural health monitoring (SHM), and recovery and rebuilding efforts (RRE). Standardisation of products and workflows across various applications is essential for achieving broad adoption, universal recognition, and maximum synergies. In the Swiss dynamic earthquake risk framework, the harmonisation of products into seamless solutions that access the same databases, workflows, and software is a crucial component. A user-centred approach utilising quantitative and qualitative social science tools like online surveys and focus groups is a significant innovation featured in all products and services. Here we report on the key considerations and developments of the framework and its components. This paper may serve as a reference guide for other countries wishing to establish similar services for seismic risk reduction.
- Article
(14658 KB) - Full-text XML
- BibTeX
- EndNote
Europe faces a significant earthquake risk due to its tectonic situation, high population density, business value, and the age and condition of buildings (e.g. Danciu et al., 2022; Crowley et al., 2021). This includes areas with moderate seismic activity, such as Switzerland, where earthquakes have the potential to cause significant loss, with projected costs of major events exceeding Euro 100 billion (Wiemer et al., 2016, 2023). Building codes and retrofitting are the most effective measures to reduce earthquake risk, but emerging technologies, such as operational earthquake forecasting (OEF) or earthquake early warning (EEW), can also improve resilience by means of reducing exposure (e.g. Cauzzi et al., 2016a; Papadopoulos et al., 2023a).
The seismic risk to which a structure is exposed depends on its type, location, occupancy, and local site conditions; an individual's risk is also affected by their exact location within the structure. Seismic risk is therefore highly time-dependent (dynamic) and subject to change, because the underlying hazard, or the exposure, changes. In the short term, the risk may increase during an active seismic sequence in the vicinity. On a more immediate timescale, seismic risk is greatly increased once an event initiates and before the strong shaking begins. In the long term, seismic risk increases with rapid urbanisation and densification of the building stock. The vulnerability of certain structures may be altered by preceding events, contributing to an evolving seismic risk landscape during an ongoing seismic sequence. Compared to a static approach that assumes a constant level of hazard and risk, the dynamic risk approach allows for timely identification of changes, enabling more accurate estimates and thus more effective mitigation measures and improved safety outcomes. Here we describe the dynamic earthquake risk framework that we are implementing for Switzerland.
The national Seismic Hazard Model (SUIhaz2015; Wiemer et al., 2016) and the recently released first national Earthquake Risk Model of Switzerland (ERM-CH23; Wiemer et al., 2023; Papadopoulos et al., 2023b) serve as the basis for tools and systems which we are developing as part of a dynamic, harmonised, and user-centred earthquake risk framework for Switzerland. Within our framework, earthquake risk is evaluated consistently and in a harmonised manner, whether for the immediate next few seconds or projecting ahead for the next 5 decades. The framework uses all available information to evaluate seismic risk at various stages of the earthquake cycle (Fig. 1) and facilitates widespread dissemination and communication of the resulting information. This involves various services, products, and research developed at the Swiss Seismological Service (SED), the Department of Earth Science, and the Institute of Structural Engineering (IBK) at the Eidgenössische Technische Hochschule (ETH) Zurich, including operational earthquake (loss) forecasting (OE(L)F), earthquake early warning (EEW), ShakeMaps, rapid impact assessment (RIA), structural health monitoring (SHM), and recovery and rebuilding efforts (RRE).
Harmonisation of products and workflows across different applications is crucial to ensure broad acceptance and universal recognition of products, as well as to maximise synergies and impact. The first element of our framework is the high-quality characterisation of seismicity in Switzerland using the Swiss Seismic Network operated by the SED as the backbone (Fig. 1) with reliable monitoring and recording of seismic events, reaching a completeness magnitude of ML1.0 in most Swiss regions and ML1.5 in less densely instrumented areas. Real-time waveform data and derived catalogue parameters contribute directly to EEW, ShakeMaps, and OE(L)F, and play a key role in immediate disaster response and public safety measures.
The second element of our framework involves the assessment of ground shaking hazards. Using data from the Swiss Seismic Network, we derive predictive ground motion models, which we combine with seismotectonic and seismogenic sources (again strongly informed by the seismicity catalogue described above) for a comprehensive long-term seismic hazard assessment. These assessments are used to update seismic design guidelines and regulations, and to inform seismic risk assessment.
The third element of our framework focuses on assessing the consequences of earthquakes and estimating losses at different levels of exposure, from the national scale down to individual communities. The RIA system developed at the SED provides rapid estimates of potential structural and economic losses. The SHM and RRE systems developed at the IBK are essential for post-earthquake damage assessment of structures and recovery predictions.
Finally, a carefully crafted communication strategy accompanies the entire lifecycle of our initiative, from the development of operational services and the construction of data models to the dissemination of data products. All services and tools in our framework are based on state-of-the-art research infrastructure, including powerful computational tools and databases.
A critical component of the Swiss dynamic risk framework is the standardisation into seamless products that access the same databases, workflows, and software, and they are based on standard models: the Swiss EEW system (Massin et al., 2021) uses the same ground-motion models as employed in the Swiss ShakeMaps (Cauzzi et al., 2015, 2022) and the Swiss RIA system utilises Swiss ShakeMap as input which includes the same site amplification layers (Bergamo et al., 2023) used in ERM-CH23. Rapid impact is calculated using OpenQuake (Pagani et al., 2014) for scenario products, RIA, RRE, and probabilistic products, while the impact on people and buildings is determined from national building databases and their vulnerability. OELF calculations employ short-term seismicity forecasts in synergy with components of the hazard and risk models utilised for long-term hazard and risk calculations and RIA products. All products are informed by a single, continuously evolving earthquake catalogue, as well as continuous waveforms generated by the SED, using the national seismic networks.
All products and services feature a significant innovation, namely a user-centred approach that utilises quantitative and qualitative social science tools such as online surveys and focus groups. The visual representation of rapid impact, for instance, was developed based on feedback from focus groups and discussions with stakeholders at the federal and cantonal levels and includes new visualisations of uncertainties. The risk map was adapted to the needs of the public, which were assessed through a representative, nationwide survey.
Here we report on the main components of the Swiss dynamic earthquake risk framework, most of which have been developed within the scope of the European Union Horizon 2020 Real-time earthquake rIsk reduction for a ReSilient Europe (RISE; http://www.rise-eu.org/home/, last access: November 2023) project (Fig. 1). We start with a summary of the seismic hazard and risk in Switzerland and then continue with a description of the recent advances in seismic monitoring capabilities over the last decade, which are crucial for the downstream risk mitigation products and services that we focus on in the second part of this paper. Finally, we discuss the SED strategy for implementing and communicating earthquake hazard and risk products to the public and stakeholders in Switzerland. Our paper may serve as a reference for other countries seeking to establish a similar framework.
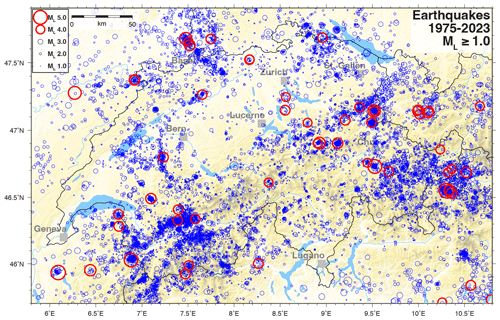
Figure 2Map of Switzerland and the surrounding area showing all seismicity of ML ≥ 1.0 since 1975 in the SED earthquake catalogue (bulletin locations). Events of ML ≥ 4.0 are highlighted by bold red circles.
Seismic hazard and risk in Switzerland
Switzerland is exposed to a considerable threat of earthquakes. Around 1000 to 1500 earthquakes are detected in Switzerland and neighbouring countries every year, including 10 to 20 events that are felt by the population (Fig. 2). The 2015 Swiss seismic hazard model, SUIhaz2015 (Fig. 3a; Wiemer et al., 2016), which assesses the likelihood of ground shaking, forecasts that earthquakes of magnitude 5 or greater are likely to occur every 8 to 15 years. The severity of impacts on buildings depends on the location and depth of the earthquake. Earthquakes with a magnitude of 6 or greater, which can cause extensive and severe damage, occur on average every 50 to 150 years and can strike any part of Switzerland at any time. The last earthquake of this magnitude occurred close to the town of Sierre in the Upper Valais in 1946 (Fäh et al., 2011). The canton of Valais faces the highest level of seismic hazard in Switzerland, followed by Basel, Grisons, the St. Gallen Rhine Valley, and central Switzerland.
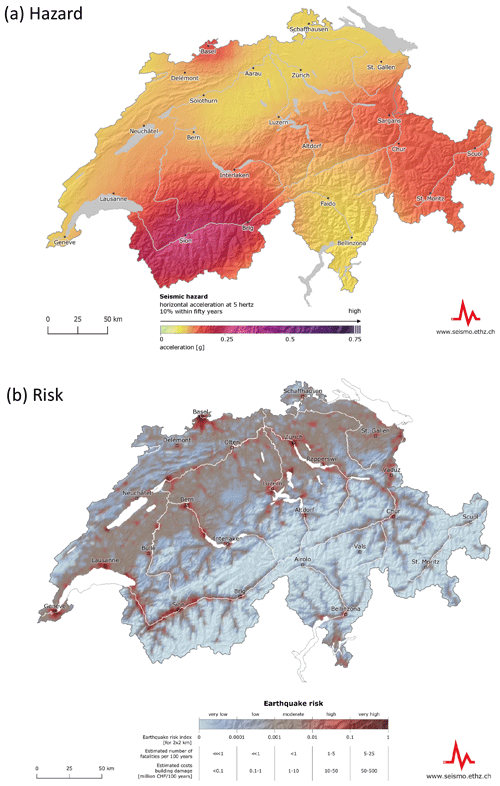
Figure 3(a) Swiss Hazard Map (SUIhaz2015; Source: Swiss Seismological Service at ETH Zurich) showing the horizontal acceleration at 5 Hz; the probability of a building constructed on rock-like ground type experiencing this is 10 % within 50 years (i.e. mean return period of 475 years). On average, 5 Hz represents the natural frequency of buildings with two to five floors, which make up the largest proportion of construction in Switzerland. The value of 475 years underlies Swiss Seismic Building Codes: an earthquake-resistant residential or office building should be able to withstand any earthquake with an average return period of 475 years. (b) National Earthquake Risk Model of Switzerland (ERM-CH23; Source: Swiss Seismological Service at ETH Zurich). The colour scale refers to a composite index based on equally weighted, normalised average structural/nonstructural loss and fatalities during 100 years. Values are provided in a 2×2 km grid. Comparatively high seismic risk is shown in dark red, lower risk is pale blue.
SUIhaz2015 has been implemented in the most recent version of Swiss building code SIA 261 (2020). It updated the hazard model from 2003 (Giardini et al., 2004). The first seismic hazard model for Switzerland, used in Swiss building codes until 2003, was that of Sägesser and Mayer-Rosa (1978) which was based on the historical catalogue available at that time, as well as on macroseismic intensity data.
Methods for estimating site-specific amplification and local seismic hazard were developed at the SED during the past decades and were implemented in microzonation studies, e.g. as for the region of Basel (e.g. Fäh and Huggenberger, 2006). A number of approaches were developed to estimate site-specific amplification based on geophysical measurements and earthquake recordings (e.g. Edwards et al., 2013; Michel et al., 2017; Poggi et al., 2017; Perron et al., 2022; Panzera et al., 2021, 2022). Recently, a project started to update the microzonation for the Basel region. All this experience was used to define the elastic response spectra in the Swiss building code (2020) and to implement a national regulation related to microzonation in SIA 261 (2020).
Geographic features such as large and deep peri-alpine lakes, steep slopes, and alluvial basins with a high water table make Switzerland susceptible to secondary hazards (e.g. Fritsche et al., 2012; Fäh et al., 2012). Using geophysical imaging, seismic monitoring, numerical modelling, and other techniques, the SED has been conducting research on earthquake-induced hazards including (i) rockfalls and landslides (e.g. Burjánek et al., 2018; Kleinbrod et al., 2019; Glueer et al., 2021; Häusler et al., 2022), (ii) lake tsunamis (e.g. Strupler et al., 2018; Kremer et al., 2022; Shynkarenko et al., 2022), and (iii) liquefaction (e.g. Fritsche et al., 2012; Roten et al., 2014; Janusz et al., 2022). Findings from these studies have been incorporated into rapid estimates of earthquake-induced mass movements and liquefaction probabilities via the SED ShakeMap application (Cauzzi et al., 2018a; Sect. 3.3).
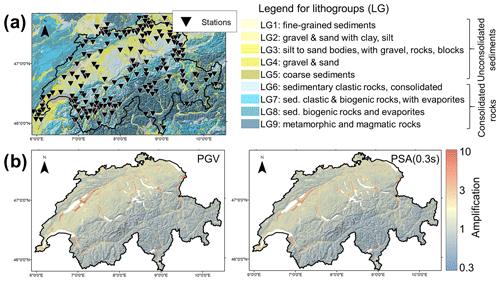
Figure 4(a) Geographical locations of the 243 (urban) free-field stations having recorded at least five earthquakes with signal-to-noise ratios > 3 in the period 2000–2021, superimposed on the lithological classification of Switzerland employed to derive the national soil response model. (b) PGV (left) and PSA (0.3 s) (right) amplification maps (with Vs30 = 1105 m s−1), part of the national soil response model (Bergamo et al., 2023).
While seismic hazard in Switzerland has been extensively studied, a formal effort to quantify seismic risk, which assesses the potential impact of earthquakes on both people and structures, as well as the resulting financial losses, was not available in the public domain until recently. In March 2023, the SED, in partnership with the Federal Office for the Environment (FOEN) and the Federal Office for Civil Protection (FOCP), released the first National Earthquake Risk Model of Switzerland (ERM-CH23; Fig. 3b; Wiemer et al., 2023). ERM-CH23 is implemented for use with OpenQuake (Pagani et al., 2014), developed by the Global Earthquake Model (GEM) foundation. As with most contemporary risk models, ERM-CH23 follows a modular structure (Mitchell-Wallace et al., 2017), with five generally decoupled components pertaining to seismic hazard on a reference rock, amplification, structural vulnerability, exposure, and consequence models. These components were developed through collaboration with national and international partners. Unlike past attempts that sought to assess earthquake risk at a continental (Crowley et al., 2021) or global scale (Silva et al., 2020), ERM-CH23 is largely supported by high-quality and high-resolution data.
ERM-CH23 has been developed to estimate the economic damage in Switzerland caused by earthquakes, which resulted in a projected average cost of CHF 11 to 44 billion for buildings and contents alone, over a 100-year period. Urban areas, particularly the cities of Basel, Geneva, Zurich, Lucerne, and Bern, face the greatest risk due to their size and the concentration of people and assets that could be impacted by an earthquake. Additionally, these cities contain numerous vulnerable buildings located on soft soil types, which can significantly amplify seismic waves. As a culmination of many years of research and expertise at the SED (e.g. Michel et al., 2017; Hobiger et al., 2021; Bergamo et al., 2021), a national site amplification model (Fig. 4) has been created as part of ERM-CH23, using geospatial prediction techniques constrained on local site response measured at instrumented sites (Bergamo et al., 2023). This model is based on (i) the direct mapping of observed site amplification factors at about 245 seismic stations, extracted with empirical spectral modelling technique (ESM, Edwards et al., 2013) and (ii) layers of site condition indicators (multi-scale topographic slope, estimated bedrock depth, lithological classification of soil; Fig. 4a). The dataset of empirical amplification factors was finally interpolated over the national territory using site condition proxies as predictor variables and the regression kriging algorithm (Hengl et al., 2007) as a geospatial prediction framework. The resulting amplification model consists of four soil response layers for peak ground velocity, PGV, and 5 % damped pseudo-spectral acceleration, PSA, at periods of 1.0, 0.6, and 0.3 s (e.g. Fig. 4b), each with associated maps of epistemic and aleatory variability following the definition in Al Atik et al. (2010). The amplification maps for PGV and PSA were also converted into layers of aggravation or reduction in macroseismic intensity by means of the relations of Faenza and Michelini (2010, 2011).
The exposure model represents the results of a national effort to obtain an extensive georeferenced database of all building objects in Switzerland. ERM-CH23 makes use of over 2.25 million building entries in the database, after excluding close to 900 000 too small or unclassified objects. Among others, for each object the building database contains information such as the period of construction, building function, footprint area, volume, height, and reconstruction cost (determined for each building individually and validated with data from the cantonal building insurance companies). Together with ground surveys to assess the frequency of different building materials in several cities, they underpin the ERM-CH23 exposure model. The number of occupants in each building is defined through de-aggregation of georeferenced housing and employment statistics (Papadopoulos et al., 2023b).
Relying on the aforementioned surveys and past experience, the building taxonomy proposed in Lagomarsino and Giovinazzi (2006) was found to be suitable and applicable to Switzerland, with minor modifications. Two sets of fragility curves were derived, one in terms of macroseismic intensity for the relevant part of the logic tree of the overall model and one in terms of spectral acceleration (at 0.3 or 0.6 s) for the other part (Wiemer et al., 2023). The former relies on the methodology described in Lagomarsino and Giovinazzi (2006) and Lagomarsino et al. (2021), together with engineering judgement about Swiss practice. For the development of the latter, a statistical investigation of building blueprints was first performed to identify average geometric characteristics of various building types. Capacity curves, idealised in bilinear form, were obtained from numerical models (Lestuzzi et al., 2017).
2.1 Swiss Seismic Network
The Swiss Seismic Network counts today about 220 permanent stations (network code CH; Swiss Seismological Service (SED, 1983) with the aim of monitoring seismic activity in Switzerland, supporting scientific research, and assessing seismic hazard and risk (Clinton et al., 2011; Diehl et al., 2021b; Fig. 5). The network is divided into two main groups of stations. The first group is composed of about 50 backbone broadband stations (known as the “SDSNet”) that have very sensitive seismic sensors (broadband velocity instruments, often referred to as “weak-motion”) placed in quiet areas with optimal vault conditions. These stations are evenly spread throughout Switzerland and can detect and locate microseismic activity. Each of these sites also has a state-of-the-art force-balance accelerometer (often referred to as a “strong-motion” instrument). The second group is composed of approximately 150 strong-motion stations (known as “SSMNet”) that are primarily located in high-risk urban areas of Switzerland, such as Basel and the Rhone Valley in the Valais (e.g. Clinton et al., 2011; Cauzzi and Clinton, 2013). The SSMNet network is concluding a multiannual renewal project (2009–2023) which involved the renovation and significant expansion of the network, as well as the systematic site characterisation of all newly instrumented sites (Michel et al., 2014; SED, 2015; Hobiger et al., 2021). In addition to these permanent stations, the Swiss Seismic Network operates another ∼70 temporary stations, which were installed for a variety of reasons, including the monitoring of geothermal exploration (SED, 2006), aftershocks and seismic sequences (SED, 2005), mass movements (SED, 2012), glaciers (SED, 1985), underground rock physics laboratories (SED, 2018a), and for risk studies (SED, 2018b). The particularly dense network infrastructure in the Valais is host to the Valais Near Fault Observatory (Chiaraluce et al., 2022). An extra ∼10 stations inside Switzerland but operated by external providers are included in the SED processing to improve the detection and characterisation of seismic events, e.g. related to geothermal exploration (SED, 2021). Around 50 stations operated by seismic agencies in neighbouring countries are also included in the real-time monitoring, which are crucial for accurate event location and lowering the magnitude of completeness in and around the border regions.
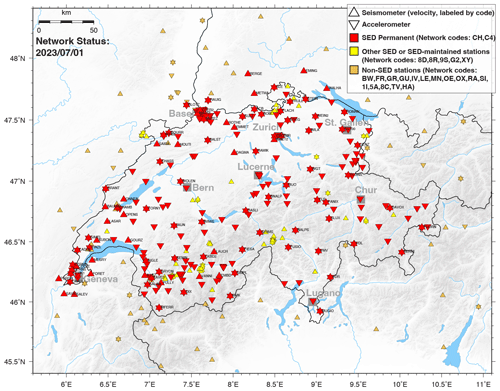
Figure 5Map of Switzerland and the surrounding area showing broadband seismometers and strong-motion accelerometers monitored by the Swiss Seismic Network as of July 2023. The map shows permanent and temporary stations operated by the SED, as well as stations operated by external partners inside and outside of Switzerland.
The majority of broadband sensors in the Swiss Seismic Network are Streckeisen STS-2 and STS-2.5 and Nanometrics T240 or T120; the Kinemetrics EpiSensor is deployed for strong-motion stations. The network uses modern ultra-low latency digitisers (typically Nanometrics Centaur, Taurus, and Kinemetrics Q330), and most sensors are acquired at sampling rates between 200 and 250 samples per second. A newly developed sensor concept allows the SED to easily deploy large numbers of temporary stations rapidly in more remote locations with real-time streaming. GNSS datasets are currently not collected, processed, or integrated by the SED.
2.2 Seismic data processing
Over the past 20 years, the number of stations within the Swiss Seismic Network has grown steadily. Data have been continuously archived since 1999, with the advent of the first broadband sensors. Today, continuous data collection is standard and the network collects around 20 GB of data every day; the total archive size is currently close to 100 TB. The SED operates a European Integrated Data Archive (EIDA) node (Strollo et al., 2021), and the majority of the waveform data, along with the SED earthquake catalogue, are open and accessible via community-standard International Federation of Digital Seismograph Networks (FDSN) web services for data access and download (Table 1).
Table 1Status of earthquake risk-related products and services in Switzerland (as of November 2023).
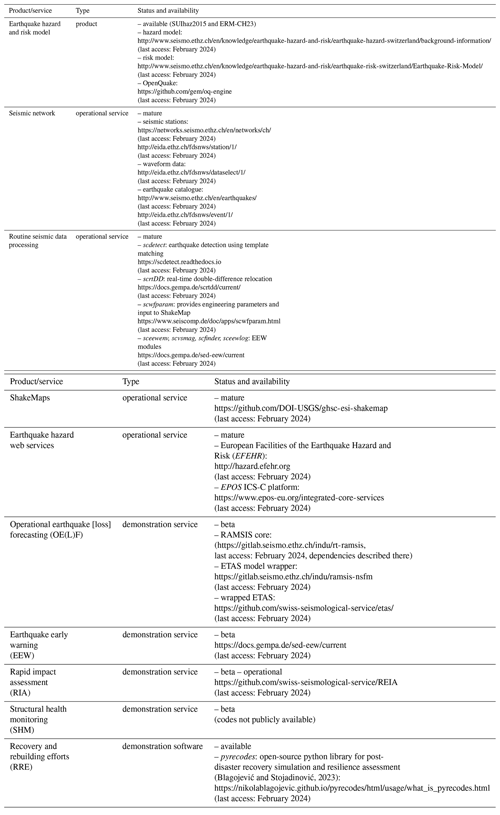
Since 2012, the Swiss Seismic Network has been utilising SeisComP (https://www.seiscomp.de/, last access: November 2023), a software developed by the German Research Centre for Geosciences (GFZ) Potsdam and gempa GmbH (https://www.gempa.de/, last access: November 2023), for earthquake monitoring and seismic data processing. SeisComP supports real-time data acquisition, archiving, and distribution, as well as automated earthquake detection and quantification, manual earthquake review, and catalogue management.
2.2.1 Detection and location
The real-time automated processing at the SED involves event triggering using station-specific short term-average (STA)/long term-average (LTA) thresholds, refined post-picking using Baer (Baer and Kradolfer, 1987) and Akaike information criterion (AIC) pickers, association of picks using scautoloc or scanloc (Grigoli et al., 2018), and location of events with NonLinLoc (Lomax et al., 2000) using Swiss-specific 1D and 3D velocity models. Several projects have been initiated at the SED over the last decades to improve the existing velocity models at different scales. A first 3D P-wave velocity model for Switzerland was developed by Husen et al. (2003), followed by a regional 3D local earthquake tomography (LET) P-wave velocity model by Diehl et al. (2009). A refined Pg and Sg LET model (parametrisation km) was computed by Diehl et al. (2021a). In their study, Diehl et al. (2021a) demonstrated that a sub-kilometre accuracy of epicentres can be achieved in most parts of Switzerland by using Pg and Sg phases in combination with an accurate 3D velocity model and the dense seismic network operated by the SED. Especially in very densely instrumented parts of the network, in which the distance to the closest observing station is smaller than 1.5 times the focal depth for most of the seismicity, the new velocity model also achieves sub-kilometre accuracy of focal depths (Diehl et al., 2021a; Lee et al., 2023). This 3D velocity model has been used for relocation and high-resolution seismotectonic interpretations in several recent studies (e.g. Lanza et al., 2022; Diehl et al., 2023) and, since June 2022, has been the standard model for bulletin locations by the SED. Furthermore, the LET model was locally improved in southwestern Switzerland by application of a staggered-grid approach, resulting in a km model parametrisation for the region of the Rhône–Simplon fault zone (Lee, 2023). The SED is working on an extension of these models to the entire crust, a Swiss-wide 3D Qp and Qs attenuation model, and a new Alpine-wide 3D P-wave crustal model using the data of the AlpArray experiment (e.g. Hetényi et al., 2018).
2.2.2 Source characterisation
Over the last couple of years, the SED has updated its strategy for magnitude determination to align it with the latest developments in engineering seismology and seismic hazard studies in Switzerland. This includes the adoption of a new local magnitude relationship MLhc (Edwards et al., 2015; Racine et al., 2020) and the seamless computation of the moment magnitude, Mw, based on spectral fitting, MwSpec (Edwards et al., 2010). In this article we use the generic “ML” notation for local magnitudes at the SED. Station corrections for local magnitudes have been included, and these changes have been implemented retrospectively for all events since 1 January 2009. Since November 2021, MLhc has been the authoritative Swiss-specific local magnitude used by the SED, and its computation has been integrated with SeisComP. Magnitudes are provided for all origins, and the preferred origin is selected using a SED developed origin score that considers the number of picks, pick residuals, and azimuthal gap. For earthquakes larger than ML 2.5, alerts are automatically sent to federal and cantonal authorities (Sect. 4.2), a ShakeMap is created (Sect. 3.3), and the strong-motion portal (http://strongmotionportal.seismo.ethz.ch/home/, last access: November 2023) is populated. Manual review is performed using the SeisComP scolv GUI. For large events, of ML > 3.5, manual moment tensors are calculated using the scmtv GUI and published in SED reports released once every year/every 2 years (e.g. Diehl et al., 2021b). The earthquake catalogue is curated through scolv. The SED is currently working on strategies to disseminate and visualise its existing first-motion and moment-tensor catalogues for public access.
2.2.3 Advanced processing
In addition to the SeisComP standard modules mentioned above, the SED has developed internally, or with support from gempa, specific modules for advanced processing. These include
-
scwfparam for providing engineering intensity measures and input to ShakeMap (Cauzzi et al., 2016b);
-
sceewenv, scvsmag, scfinder, and sceewlog for EEW (Massin et al., 2021);
-
scdetect for earthquake detection using template matching (see below);
-
scrtDD for real-time double-difference relocation (see below).
2.2.4 Earthquake detection from template matching – scdetect
Real-time earthquake detection is crucial for the characterisation of earthquake sequences. Scdetect is a highly configurable module for real-time earthquake detection based on template matching using computationally efficient waveform cross-correlation (Armbruster et al., 2022; Mesimeri et al., 2024). The workflow of scdetect is fully integrated with the SeisComP architecture and allows users to visualise and refine the detected earthquakes using SeisComP's built-in GUI applications. Scdetect is currently being real-time tested in Switzerland in areas of high seismic activity using templates from past earthquake sequences with the goal of detecting small magnitude earthquakes that are missed by the current operational pipelines.
2.2.5 Real-time double-difference relocation – scrtDD
To understand the spatio-temporal evolution of natural and induced seismicity, it is essential to have real-time, high-precision hypocentre locations, allowing one to determine the geometry and extent of seismically active faults, as well as the volume affected by stimulation procedures. The spatio-temporal evolution of seismicity can also provide information about fluid-flow processes and hydraulic properties, including the possible existence of hydraulic connections (e.g. Diehl et al., 2017). Although relative relocation procedures have been developed for decades (e.g. Console and Di Giovambattista, 1987; Waldhauser and Ellsworth, 2000), they are rarely applied in routine, real-time processing. To address this, the SED has developed the scrtDD software module (Scarabello and Diehl, 2021), which performs real-time and near-real-time double-difference relocations following the procedures described in Waldhauser and Ellsworth (2000) and Waldhauser (2009) within the SeisComP architecture. The module combines differential times derived from automatic and manual picks as well as waveform cross-correlation with archived data from past nearby events (Scarabello et al., 2020). The differential-time data are subsequently inverted to compute the single-event relative location of a newly detected earthquake with respect to the double-difference background catalogue following the procedure of Waldhauser (2009). The module also includes the capacity to generate or update a double-difference background catalogue using the standard multi-event double-difference method of Waldhauser and Ellsworth (2000). To ensure that new events are continuously included in the background catalogue and that real-time relocations remain accurate in areas of sparse background seismicity, the SED has implemented both single-event and multi-event relocation procedures in their operational monitoring system since 2021. Currently, the SED is developing and testing concepts for more advanced visualisation and dissemination of SED's double-difference catalogues.
Other advanced methods, which are currently being explored and evaluated at the SED, include the methods described in the following sections.
2.2.6 Noise interferometry
To monitor variations in mechanical and structural properties in the crust, the SED is applying seismic noise interferometry techniques, which involve reconstructing approximative Green's functions, typically referred to as cross-correlation functions, by correlating continuous ambient seismic noise records (e.g. Nakata et al., 2019). From the cross-correlation functions, ballistic waves are used to image the subsurface (e.g. Obermann et al., 2016; Molinari et al., 2020) and coda waves are used for time-lapse imaging (e.g. Obermann et al., 2013, 2014). Unlike earthquakes, seismic noise offers a constant source of signals that can be recorded anywhere on Earth. The spatial resolution of noise interferometry is primarily limited by the geometry and aperture of the seismic network, as well as the stability in noise excitation across frequency bands. While sparse, noisy stations often only allow for the reconstruction of the fundamental-mode surface wave, quiet stations in dense arrays allow for the reconstruction of body waves with a much-increased depth resolution. In addition to the monitoring of natural processes, coda wave-based noise interferometry has great potential for the time-lapse monitoring of local engineering applications, such as dams, hydraulic stimulations, or carbon storage. Changes in seismic velocity and waveform similarity are proxies for aseismic stress changes in the subsurface that could indicate weakening, stress build-up, or imminent failure. At geothermal project sites, coda wave interferometry has already proven its potential to detect unexpected reservoir dynamics earlier than the microseismic response alone (Obermann et al., 2015; Hillers et al., 2015; Toledo et al., 2022; Sánchez-Pastor et al., 2019) and as such could become a valuable contributor to our earthquake risk framework in the future.
2.2.7 Fibre-optic deformation sensing
During the past decade, fibre-optic sensing techniques, previously used mostly for perimeter security and infrastructure monitoring applications, have emerged as a new seismic recording paradigm. In particular, distributed acoustic sensing (DAS) offers high spatial resolution at the metre scale, as well as a frequency bandwidth from megahertz to kilohertz (e.g. Lindsey et al., 2020; Paitz et al., 2021). Complementing conventional seismometer recordings, DAS fills a niche in cases where kilometre-long fibre-optic cables can either be co-used or easily deployed. The former includes fibre-optic sensing in densely populated cities (Ajo-Franklin et al., 2019; Spica et al., 2020), under water (Spica et al., 2022), or in avalanche-prone regions (Paitz et al., 2023), with the help of telecommunication fibres. This enables urban subsurface imaging with a lateral resolution on the order of 10 m and the detection of earthquakes and avalanches for monitoring and early warning purposes. On volcanoes, glaciers, and ice sheets, fibre-optic cables for sensing applications can often be deployed with relative ease, thereby providing new opportunities for high-resolution studies of volcanic or glacial dynamics (Walter et al., 2020; Klaasen et al., 2021; Jousset et al., 2022). More recent developments in integrated fibre-optic sensing overcome the limited interrogation distance of DAS, typically several tens of kilometres, at the expense of reduced spatial resolution (Marra et al., 2018; Bogris et al., 2022). Applications of integrated sensing for seismic imaging and earthquake characterisation, especially in the oceans, are promising but still in their infancy. At the current stage, fibre-optic seismology is still in exploratory mode, and the identification of clear applications where it would be beneficial within an earthquake risk framework is work in progress. Specific next steps include the routine incorporation of DAS data in near-real-time earthquake detections and locations, as well as the detection of secondary effects, such as landslides and avalanches using existing telecom infrastructure.
2.2.8 Machine learning
Over the last couple of years, machine learning and deep learning techniques have started to rapidly transform earthquake seismology (e.g. Mousavi and Beroza, 2022). Automated seismic processing methods are currently capable of producing large data products (such as seismicity catalogues) of high quality that match or even exceed the reliability and fidelity of those made by human data processing experts. The SED is actively pursuing research in deep learning-based earthquake science, including event classification, seismicity monitoring methods, site characterisation, planetary seismology, and seismicity forecasts (e.g. Maranò et al., 2012; Hammer et al., 2013; Meier et al., 2019; Dahmen et al., 2022). This work involves implementing various machine learning models for seismic signal denoising, phase detection and arrival time estimation, signal / noise classification, phase association, first motion polarity classification, and others. The SED uses non-machine learning-based methods as benchmarks to evaluate the effectiveness of these new approaches. For all monitoring tasks, the SED plans to compare established and available models against newly trained models and models transfer-learned using Swiss data. A crucial aspect of these efforts will be the testing of the machine learning methods at various scales of seismicity monitoring, including underground laboratory experiments, geothermal reservoir scales, as well as national and regional monitoring scales.
3.1 Operational earthquake (loss) forecasting (OEF and OELF)
Operational earthquake forecasting (OEF) and operational earthquake loss forecasting (OELF) are scientific approaches to forecasting the short-term probability of occurrence and the associated economic and societal impact of earthquakes. OEF utilises statistical analysis of historical earthquake data, seismic activity patterns, and geological features in a specific region to determine the probability of earthquakes above a certain magnitude occurring over a given time period. OELF builds upon these OEF probabilities and assesses the potential loss of life, property, and infrastructure that could result.
Earthquake probabilities and the resulting short-term hazard and risk can vary by several orders of magnitude between quiet periods and clustered sequences, such as aftershocks sequences or swarms (Van Stiphout et al., 2010). Unlike long-term earthquake forecasts, which inform long-term risk mitigation measures such as building codes, the operationally calculated short-term earthquake probabilities and the corresponding loss estimates generated by OEF and OELF respectively provide crucial information for crisis management in case of a major earthquake. To complement the long-term earthquake forecasts that are part of SUIhaz2015, the SED is therefore working on an epidemic-type aftershock sequence (ETAS) earthquake forecasting model (Ogata, 1988) for Switzerland that describes the temporal fluctuations in earthquake probabilities. ETAS models are well suited for this task: they are being used for OEF by agencies worldwide (Marzocchi et al., 2014; Harte, 2019; Van der Elst et al., 2022) and are the most extensively tested time-dependent models available (Woessner et al., 2011; Ogata et al., 2013; Strader et al., 2017; Savran et al., 2020). Furthermore, an expert elicitation conducted within the RISE project showed wide consensus among experts that the ETAS model shall be used as a default model for earthquake forecasting (Mizrahi et al., 2023). In ETAS, earthquakes are partitioned into background seismicity and aftershock clusters. In the Swiss case, the background seismicity model is based on the SUIhaz2015 time-independent rate forecast, and clustered seismicity is modelled using ETAS parameters calibrated with the local SED earthquake catalogue. The SED is developing and testing multiple ETAS-based models for Switzerland (Mizrahi, 2022), ranging from simple models that only rely on a comprehensive earthquake catalogue as input to more complex models that consider variations in catalogue completeness and additional information from SUIhaz2015. To evaluate the performance of the models, pseudo-prospective forecasting experiments and retrospective consistency tests (Cattania et al., 2018; Nandan et al., 2021; Bayliss et al., 2022) are being conducted.
Besides the scientific model to probabilistically describe future earthquake occurrence, the SED is also developing the IT infrastructure required to produce automated earthquake and loss forecasts for Switzerland in real-time (see example in Fig. 6). Both systems are initially operated internally at the SED for evaluation and refinement and will at a later stage be made available to federal agencies and the general public in Switzerland. In particular, the OELF system will provide actionable information to individuals, public authorities, and other stakeholders, based on the updated earthquake rate forecast from the OEF system and ERM-CH23. Strategies for the effective communication of earthquake probabilities and uncertainties to the public are important and have been and continue to be extensively studied at the SED using surveys and discussions with focus groups and stakeholders at the federal and cantonal levels (Sect. 4.2).
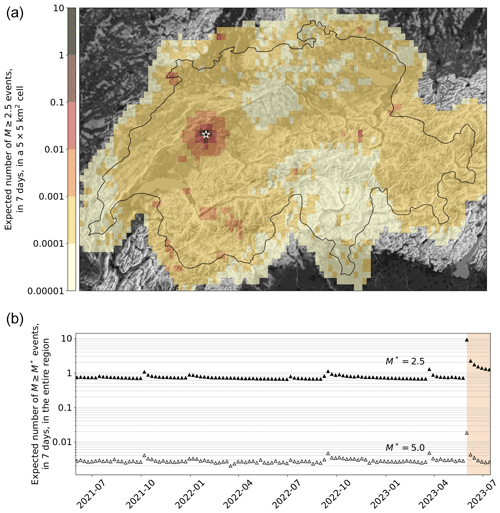
Figure 6Time-dependent earthquake forecast for Switzerland after a hypothetical Mw 6.0 earthquake near Bern, Switzerland, at midnight on 1 June 2023 (white star in a). (a) Spatial distribution of the expected number of felt earthquakes (ML ≥ 2.5) in the first 7 d following the event, per grid cell (roughly 5×5 km). (b) Temporal evolution of 7 d forecasts for the entire region shown in (a). The filled and empty triangles represent the expected number of ML ≥ 2.5 and ML ≥ 5.0 earthquakes respectively. The shaded background marks the time interval after the occurrence of the Mw 6.0 event.
3.2 Earthquake early warning (EEW)
Earthquake early warning (EEW) systems are designed to rapidly detect earthquakes and provide people and automated systems with time to prepare and take protective action before strong shaking arrives (e.g. Allen et al., 2009; Cremen and Galasso, 2020). Although the EEW provided alert times are short (depending on the distance between the earthquake and the location to be warned), they are considered sufficient to allow taking cover, stopping trains or elevators, shutting down industrial processes, or triggering automated shutdown systems. EEW is considered an important tool for earthquake risk reduction and disaster management, as it may help to reduce the number of casualties and damage to infrastructure and buildings during an earthquake, as well as to minimise social and economic disruption (e.g. Papadopoulos et al., 2023a).
For around 1 decade, the SED has been developing open-source software and methods for EEW using a set of SeisComP modules (such as sceewenv, scvsmag, sceewlog, and scfinder) known as the ETHZ-SED SeisComP EEW (ESE) system (Massin et al., 2021). The core of ESE is formed by the Virtual Seismologist (VS; Cua, 2005) and Finite-Fault Rupture Detector (FinDer; Böse et al., 2012) algorithms. VS provides fast EEW magnitudes using existing SeisComP detection and location modules, while FinDer identifies fault rupture extent by matching growing patterns of observed high-frequency seismic acceleration amplitudes with modelled templates. The SED is currently developing a new SeisComP module to compare the observed and predicted ground-motion envelopes with the goal to select origins and magnitudes from the independent VS and FinDer source parameter estimates, while suppressing false alerts (Jozinović et al., 2023).
In Switzerland, VS and FinDer are not yet used for public alerting but rather for testing and demonstration of EEW (see example in Fig. 7). VS uses phase picks to provide fast locations and magnitudes for any event detected by the Swiss Seismic Network, while FinDer is typically activated only for earthquakes with magnitudes greater than 3.5. The median delay for the first VS (since 2014) and FinDer (since 2017) alert is 8.7 and 7 s respectively, but earthquakes are frequently detected in as little as 4 to 6 s when they occur in areas with a high station density (Massin et al., 2021). Typically, it takes 3.5 s for the P-waves to propagate from the hypocentre to the fourth closest station in the Swiss Seismic Network, the minimum number of stations required by the algorithms. The SED continues to optimise the Swiss Seismic Network for EEW, although the benefit from further station densification appears limited (Böse et al., 2022). Despite the rare occurrence of large earthquakes in Switzerland, a recent public survey shows that 70 % of the Swiss population would like rapid notifications for all earthquakes that are felt, even if they have a low damage potential (Dallo et al., 2022a). Future mass notifications for EEW in Switzerland could be enabled either through the Swiss Alertswiss and MeteoSwiss multi-hazard platforms, which can receive and display push notifications on mobile devices, or through cell broadcast once available.
3.3 Swiss ShakeMaps
Ground-motion maps provide critical information on the severity and distribution of ground shaking generated by an earthquake. The SED has been utilising the ShakeMap® application (Worden et al., 2020) in Switzerland for approximately 15 years (Cauzzi et al., 2022) and is a core founder and contributor to the European ShakeMap initiative that promotes international collaboration and harmonisation of ShakeMap procedures in the greater European region (Cauzzi et al., 2018b; Michelini et al., 2023). ShakeMap rapidly maps seismic shaking information based on recorded and predicted intensity measures, such as peak ground acceleration (PGA), PGV, PSA, and macroseismic intensity levels, including amplification due to local site effects.
The SED ShakeMap framework is updated regularly and employs Swiss-specific ground-motion models, ground-motion-to-intensity conversion equations, and site amplification models (which are the same as those used in ERM-CH23; “Seismic hazard and risk in Switzerland” section) that allow for accurate and reliable ground shaking estimates across the Swiss alpine and northern foreland regions (Cauzzi et al., 2015). The SED maintains an archive of instrumental ShakeMaps for events with a magnitude larger than 2.5 that have occurred since 1991 and an atlas of large historical ShakeMaps. There are plans to include rapid finite-fault information in the SED ShakeMaps in the near future (Böse et al., 2012).
ShakeMaps are an important tool for earthquake response and recovery efforts. At the SED, ShakeMaps serve multiple purposes. They are used (i) to inform the Swiss public about the severity of ground shaking and affected areas (see example in Fig. 7); (ii) to estimate the likelihood of earthquake-triggered mass movements for significant events, following a set of geospatial susceptibility proxies and PGA (Cauzzi et al., 2018b); and (iii) to rapidly assess the potential damage caused by ground shaking as part of the SED RIA system (Sect. 3.4).
3.4 Rapid impact assessment (RIA)
Rapid impact assessment (RIA) involves the gathering and analysis of information to quickly assess the damage and impact of an earthquake (or other) disaster. RIA systems shall provide decision-makers with timely and accurate information to guide their response and recovery efforts. The RIA processing chain involves (i) the assessment of the extent and severity of the damage, (ii) the evaluation of the needs of the affected population, and (iii) the identification of priority areas for response. RIA efforts in Switzerland currently focus on the first step.
The SED RIA system uses OpenQuake's scenario calculator (Pagani et al., 2014) and Swiss ShakeMaps (Sect. 3.3). Once an earthquake's location and magnitude are determined, a ShakeMap is created and the RIA calculator activated. Monte Carlo simulations are then used to generate multiple ground-motion field realisations at the location of the building assets in the ERM-CH23 exposure model. Damage and loss estimates are derived using the vulnerability functions associated with each asset and the simulated ground-motion values. The SED RIA system estimates various types of losses (damage, economic loss, injuries, deaths, and shelter needs) at the national, cantonal, and municipal levels. These estimates are compiled in a standard format (Sect. 4.2), which includes a map of ground shaking and visualisations of losses – along with associated uncertainties – at different scales (see example in Fig. 8). In the future, the SED RIA system will become fully integrated and synchronised with the Swiss Seismic Network operations and perform near-real-time calculations for every earthquake with magnitude M>3.0 within a specified radius around Switzerland. For now, the RIA results are shared internally at the SED for verification but will soon be made available to the public.
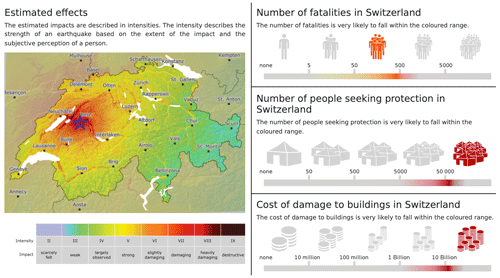
Figure 8Example rapid impact assessment (RIA; Source: Swiss Seismological Service at ETH Zurich) output (here national level estimate) for a hypothetical Mw 6.0 earthquake near Bern. See http://www.seismo.ethz.ch/static/ERM-CH23/scenario/Bern_M6_0_en.pdf (last access: February 2024) for full report. Damage cost given in CHF.
3.5 Seismic hazard web platform and services
Among other dynamic and operational earthquake-related services, the SED is actively involved in the development, maintenance, and hosting of a web platform that grants access to a wide range of earthquake hazard datasets, input models, results, documentation, and information at both the national and regional levels. This web platform, accessible at http://hazard.efehr.org (last access: February 2024), is an integral part of the European Facilities of the Earthquake Hazard and Risk (EFEHR) network of federated services. Moreover, the earthquake-related hazard data, products, and services are designed to be interoperable with the newly developed EPOS ICS-C platform (Haslinger et al., 2022).
The hazard platform comprises three individual web applications that enable users to interactively explore and retrieve hazard curves, hazard spectra, and hazard maps. Through a user-friendly interface, users can access hazard data and related metadata. The platform streamlines the retrieval of hazard maps, which can be disseminated to users through multiple avenues, including customised services offering ASCII data, file downloads featuring compressed ESRI shapefiles, and adherence to the OGC standards, which facilitate the distribution of projected map images.
The EFEHR web portal serves as a gateway to various seismic hazard models, including the 1999 Global Hazard Map of the Global Seismic Hazard Assessment Program (GSHAP, Giardini, 1999), the 2013 European Seismic Hazard Model (ESHM13, Woessner et al., 2015), the 2014 Earthquake Model of the Middle East (EMME14, Giardini et al., 2018), the 2015 Swiss Hazard Model (SUIhaz15; Wiemer et al., 2016), and the 2020 European Seismic Hazard Model (ESHM20; Danciu et al., 2021). Furthermore, this platform will be the principal repository for results and datasets related to the ERM-CH23 (Wiemer et al., 2023).
3.6 Structural health monitoring (SHM)
Due to slow retrofit and replacement rates of existing buildings, slow uptake of modern earthquake resistance standards, and the intensity of extreme events, earthquakes pose a significant threat to the built environment. Post-earthquake inspections are necessary to assess the damage to buildings and ensure safe shelter for the population. Current expert-conducted visual inspections suffer from possible subjectivity and delay recovery. However, recent advances in sensor development offer reliable and cost-effective sensing hardware, making broad monitoring of multiple conventional buildings realistic. Structural health monitoring (SHM) provides tools to analyse these sensor data and to translate vibration data into meaningful information about the structural state of a building. Damage-sensitive features (DSFs) can be extracted from continuous measurements and contribute to the detection and localisation of earthquake-induced damage (e.g. Reuland et al., 2023a).
Several approaches to overcome the scarcity of real-world dynamic monitoring data of both healthy and damaged structures have been developed at the IBK: (i) SHM-based fragility functions relate probabilities of a structure to reach a given damage state to DSFs and can provide near-real-time damage tags (Reuland et al., 2021, 2023b); (ii) a machine-learning methodology relying on domain adaptation has been successfully used to transfer a damage-state classification from simulated training data to real measurements from experimentation (Martakis et al., 2023); and (iii) a framework for automated detection of malfunctioning sensors has also been developed to ensure that sensors are functional and record valuable data during earthquakes (Martakis et al., 2022). Furthermore, monitoring data from buildings can contribute to earthquake preparedness by reducing uncertainty and regional variability in capacity curves used to derive fragility functions (Martakis et al., 2022). After successful testing on individual buildings, SHM-based rapid loss assessment was recently integrated into a regional demonstrator (Nievas et al., 2023). Integrating monitoring data and engineering models into a robust framework will pave the way to making SHM-based real-time building tagging operational in Switzerland and elsewhere in the future.
3.7 Recovery and rebuilding efforts (RRE)
Recovery and rebuilding efforts (RRE) refer to the process of restoring a community or region to its pre-disaster condition after a natural or man-made disaster. The recovery phase begins immediately after the event and focuses on providing immediate assistance to affected people, restoring critical infrastructure such as power, water, and transportation systems, and providing temporary housing for those displaced by the disaster. The rebuilding phase involves longer-term efforts to repair or replace damaged infrastructure, such as roads, bridges, and buildings, and to help affected individuals and communities recover from the economic and social impact of the disaster.
Resilient communities have the ability to quickly recover from extreme events, and retrofitting measures can help decrease the risk of earthquakes and reduce repair efforts. Still, RRE are crucial to restoring community functions and minimising negative social and economic impacts. Recovery models and resilience assessment tools can simulate recovery trajectories and guide decision-makers towards effective actions. The iRe-CoDeS (interdependent Resilience Compositional Demand and Supply) framework developed at ETH Zurich (Blagojević et al., 2022) can perform such analyses and has been integrated with OpenQuake software for regional hazard and risk assessment.
Early loss assessment is often incomplete and imprecise, which hinders response efforts. To improve decision-making, a dynamic update of regional post-earthquake damage estimates is proposed in iRe-CoDeS. Gaussian process inference models are used to fuse early inspection data with a pre-existing earthquake risk model (such as ERM-CH23; Bodenmann et al., 2023), reducing uncertainty and improving regional building damage estimates. By combining regional recovery and resilience assessment tools with this framework, uncertainty in recovery trajectories can be reduced, and real-time what-if analyses can inform decision-makers on the state of the community during recovery and optimal resource deployment. The iRe-CoDeS model can be updated with early inspection information after an earthquake, providing recommendations for recovery efforts and remaining recovery time.
4.1 Operation
Providing operational services demands a high level of service availability. To achieve this, the SED provides appropriate hardware solutions, invests in professional software engineering, and provides for IT on-call duty backup. The seismic processing data centre at the SED is the operational service with the longest history and most mature setup, and it provides the template for new services as they are added to the operational ecosystem. High availability services are achieved by operating two identical software versions on fully redundant and physically separated hardware, a primary and backup system. If any issues arise on the primary system, the backup system can immediately become primary. A third server is also supported for development and prototyping. Databases are also fully replicated and backed-up, and when database information is provided to the public, it is accessed only via replicated databases to remove the possibility of external loads compromising the operational systems.
To react to operational and seismic crises, the SED operates three on-call teams, dedicated to technical IT-related issues, immediate review of all seismic events with M>2.5, and handling inquiries from authorities, media, and the public for Swiss and international events. To provide internal and external seismic alerts, an in-house developed alarm system enables the duty seismologists to take prompt action when an earthquake occurs. The seismic alerts are automatically activated when an earthquake above a specific magnitude is detected within or in proximity to Switzerland. Web portals enable the public distribution of products generated by these operational services via direct access or APIs.
The SED conforms to international community standards in data formats, metadata, and dissemination services where possible (such as FDSN mseed, stationXML, and web services), and indeed it is at the cutting edge in developing now standards; for example, the SED curates the quakeML (Schorlemmer et al., 2011) data model, ensuring that earthquake information is easily accessible and shareable. The integration of harmonised data and processes is at the heart of effective dynamic earthquake risk management and mitigation strategies.
4.2 Communication and societal perspective
As a federal agency, the SED is responsible for informing the public, authorities, and media about earthquakes affecting Switzerland, and providing warnings when needed. For this purpose, the SED monitors ground shaking in Switzerland and neighbouring countries (Sect. 2.1). Details (including time, location, magnitude, and possible impacts) of a detected earthquake are published on the SED website within 90 s. Federal and cantonal authorities are informed automatically if the magnitude is 2.5 or larger. A team of on-call duty seismologists assesses every recorded earthquake and takes further actions if needed and is available for media requests. The SED also engages in science communication during quiet times to transfer knowledge about earthquakes and related topics. To ensure effective communication, the SED interacts with societal stakeholders and co-develops and evaluates various information products, including those presented in this article. The SED also contributes to the training of future earthquake experts through teaching efforts at ETH Zurich and beyond.
Recently, the SED has been shifting from hazard to risk communication, which should increase society's preparedness and disaster resilience. To ensure effectiveness, it is important that communication products are designed by an interdisciplinary expert group and then tested with the relevant end-users before releasing them publicly. In preparation for the ERM-CH23 release in March 2023, the SED tested various output formats for risk products with professional stakeholders of society and the general public.
-
Marti et al. (2023) showed that people and professionals consider RIA reports and risk scenarios to be very important, although they appeared similarly challenged to correctly interpret the information provided. To represent the uncertainties in the model estimates, the simplest visualisation using ranges was the most understandable and the most popular (see Fig. 8).
-
Regarding EEW systems, a public survey conducted by the SED in Switzerland (Dallo et al., 2022a) revealed that the Swiss public wants to receive EEW alerts for all felt events (even if they are not damaging) and their preferences align with those in other countries. EEW alerts with pictograms have the strongest effect in motivating people to take action, even if it is not necessarily what they like best.
-
The SED has collaborated with the Winton Centre at the University of Cambridge to test OEF communications with the general public in Italy, Switzerland, and California in the US. A survey of Dryhurst et al. (2022) found that people in all three countries provided similar answers. Maps representing OEF probabilities as different coloured isoline compartments could mislead the public. The best information combination for OEF communication is a geographical map showing the forecast area, textual information about the current absolute chance of an earthquake, and a risk ladder to provide context.
-
Dallo et al. (2022b) conducted three online surveys with various experiments and virtual focus groups to improve communication of earthquake information on multi-hazard platforms, such as MeteoSwiss and Alertswiss (Sect. 3.2). The results indicated that people prefer a combination of visual and textual information, pictorial and textual behavioural recommendations, interactive features, consideration of data privacy issues, messages with time indication and action keywords, and clearly distinguishable icons of the epicentre and the person's location (Valenzuela Rodríguez, 2021).
When designing information campaigns, it is important to consider people's personal factors, which can influence their interpretation of the information provided, their design preferences, and their perceived usefulness. To achieve successful campaigns, key factors to consider include regular communication, context, channel choice, risk communicator training, and community-based approaches (Marti et al., 2020). A significant challenge is to provide personalised notifications to end-users while still addressing their concerns about data privacy.
Earthquake hazard and risk are often assumed to be constant over time. However, because seismicity is spatially and temporally clustered, and because individual exposure can change rapidly, both hazard and risk are often strongly time-dependent on different timescales. In the dynamic-risk framework outlined in this paper, seismic activity is continuously monitored by a regional seismic network, such as the one operated by the SED, and risk assessments are dynamically adapted in response to the latest data. We believe that this dynamic concept provides a more accurate and timely means of identifying potential seismic hazards and assessing risks, thereby enabling more efficient mitigation strategies and improving overall safety outcomes.
As described in this paper, we have developed key operational services of a dynamic earthquake risk framework for Switzerland. These include earthquake monitoring, ShakeMaps, EEW, OEF, RIA, and computational infrastructure. The seismic network and the ShakeMap system are currently the most mature of these services in Switzerland, followed by EEW and RIA; the OEF service is currently in a demonstration phase (Table 1). Furthermore, the SED has a well-established communication network to provide rapid earthquake information to the public over multiple channels.
Integration and interoperability are important aspects of our dynamic risk framework. Integration means that the framework must be able to accommodate different components while also remaining adaptable enough to function even when specific components are not connected. For example, some countries interested in establishing similar services may prioritise ShakeMaps and RIA while opting not to invest in EEW. Interoperability, on the other hand, entails that the various products and services within the framework should share common models and databases, thus eliminating redundancy in processing and ensuring efficient utilisation of resources. For example, the Swiss EEW system uses the same ground-motion models as ShakeMaps, and the Swiss RIA system uses the same ShakeMaps and the same site amplification layers derived for the national risk models, and calculates impacts on people and buildings based on national databases of buildings and their vulnerability.
During the development of our framework, we came across several key findings. First, the foundation of this framework is highly dependent on the existence of a robust seismic monitoring network and a high-quality data processing system and infrastructure. These components play a key role as they serve as the primary data sources for various downstream risk-related products. Secondly, the quality and effectiveness of the underlying models and methodologies are critically dependent on the incorporation of the latest scientific advances and the availability of computational infrastructure. Ensuring that the framework is kept up to date with the latest research is of paramount importance and may even be the greatest challenge in the long term. Thirdly, it is essential to involve stakeholders and target audiences at an early stage of development to ensure that products and services meet their expectations and understanding.
In the dynamic risk context, earthquake risk is assessed in a consistent and harmonised way for the next few seconds and for the next 5 decades. This not only offers great potential for synergy but also means for comparative cost–benefit analysis (CBA). While traditional CBA is useful for evaluating EEW systems (e.g. Papadopoulos et al., 2023a) or OE(L)F-based alerting systems (Van Stiphout et al., 2010; Hermann et al., 2016), alternative methods such as multi-criteria decision analysis (MCDA) have proven useful for decision-making when non-economic factors are important (e.g. Guarini et al., 2018). The flexibility and transparency of MCDA allows for the consideration of a wider range of criteria beyond economic costs and benefits, including model bias, model uncertainty, time gain in emergency response, and information gain, making it a valuable tool for assessing the cost-effectiveness of different dynamic risk products. Ongoing research aims to assess the wider benefits of these dynamic risk products for earthquake risk reduction, incorporating surveys and expert opinion to facilitate a dialogue with decision-makers and the public.
The SED continues to advance its seismic observational capabilities and risk products, including double-difference earthquake catalogues, extending 3D crustal velocity models, enhancing magnitude determination, and exploring new visualisation and distribution methods. It aims to provide short-term earthquake probabilities and associated seismic hazards and losses, to provide rapid earthquake information and EEW to the Swiss public, and to integrate the RIA system into the seismic network operations for near-real-time calculations for earthquakes in and around Switzerland above magnitude 3.0. In addition, research is ongoing to determine how best to communicate earthquake forecasts and support the translation of probabilities into actions.
We expect our framework to improve over time as individual components are improved. For example, in the near future, simulation-based approaches, such as physics-based ground motion modelling, or so-called digital twin components, may replace certain elements of the framework. Embracing the conceptual framework of dynamic risk inherently signifies a comprehensive and interdisciplinary approach to the assessment, reduction, and resilience of earthquake risks. Furthermore, this framework can be easily extended to a multi-risk framework, which offers significant advantages in addressing a variety of risk reduction challenges.
Future work on our framework could include (i) transitioning demonstration services to become operational; (ii) continuing the development and testing of the proposed services and risk framework in a wider range of countries, both in Europe and globally, exploring collaborations with interested stakeholders, and advancing the implementation of European-level services within the European Plate Observing System (EPOS); (iii) widening the scope of the proposed exposure and loss models to encompass not only buildings but also critical infrastructure, such as transportation, water, or energy, as well as high-risk industries, which play an important role in disaster risk management and emergency response efforts; and (iv) continuing and expanding the use of promising techniques like AI and DAS.
We would like to emphasise that while we believe that the dynamic and user-centric risk framework outlined here is valuable and can contribute to earthquake risk reduction, it should not detract from a strong focus on earthquake engineering efforts. Building to modern seismic standards has proven to be the most valuable means of reducing financial and human losses in future earthquake disasters and must remain a priority.
As data, models, and computing resources increase, dynamic and operational earthquake-related services will become increasingly available and important for earthquake risk assessment and mitigation. This paper may serve as a reference guide for countries wishing to establish similar tools and services in the context of dynamic risk. Links to publicly available components of our framework are provided in Table 1.
Information on all codes and datasets used in this paper can be found in Table 1.
We use the CRediT Contributor Roles Taxonomy to categorise author contributions. Methodology and investigation: hazard and risk: LD, AP, PR, SW, PB, DF, FH, BMC, DG; seismic monitoring (network and processing): JC, TD, CC, FM, FH, DF, AF, MB, FG, LH, PJ, DJ, FL, TL, MAM, AO, MS, LS, AS, SW, PK; OE(L)F: LM, SW, MH, LD, AP, PR; EEW: MB, FM, JC, DJ, CC; ShakeMaps: CC, JC, MB, PB, DF; RIA: LD, AP, PR, NS, SW; SHM: YR, EC, LB, PM, BS, NB; RRE: YR, EC, LB, PM, BS, NB. Operations and communication: JC, LD, PK, ID, MM, NV, LD, AP, PR. Writing (original draft): MB. Writing (review and editing): all. Project investigators and funding: DG, SW.
The contact author has declared that none of the authors has any competing interests.
Publisher's note: Copernicus Publications remains neutral with regard to jurisdictional claims made in the text, published maps, institutional affiliations, or any other geographical representation in this paper. While Copernicus Publications makes every effort to include appropriate place names, the final responsibility lies with the authors.
This article is part of the special issue “Harmonized seismic hazard and risk assessment for Europe”. It is not associated with a conference.
The authors used OpenAI to improve readability and language in some parts of the paper. We are grateful for the constructive feedback of three anonymous referees and Associate Editor Helen Crowley.
This article was partially funded by the European Union's Horizon 2020 research and innovation programme (grant no. 821115) “Real-time earthquake rIsk reduction for a reSilient Europe (RISE)” (http://www.rise-eu.org, last access: February 2024). Opinions expressed in this paper solely reflect the authors' view; the EU is not responsible for any use that may be made of the information it contains.
This paper was edited by Helen Crowley and reviewed by three anonymous referees.
Ajo-Franklin, J., Dou, S., Lindsey, N., Monga, I., Tracy, C., Robertson, M., Rodriguez Tribaldos, V., Ulrich, C., Freifeld, B., Daley, T., and Li, X.: Distributed acoustic sensing using dark fiber for near-surface characterisation and broadband seismic event detection, Sci. Rep., 9, 1328, https://doi.org/10.1038/s41598-018-36675-8, 2019.
Al Atik, L., Abrahamson, N., Bommer, J. J., Scherbaum, F., Cotton, F., and Kuehn, N.: The Variability of Ground-Motion Prediction Models and Its Components, Seismol. Res. Lett., 81, 794–801, https://doi.org/10.1785/gssrl.81.5.794, 2010.
Allen, R. M., Gasparini, P., Kamigaichi, O., and Böse, M.: The status of earthquake early warning around the world: an introductory overview, Seismol. Res. Lett., 80, 682–693, https://doi.org/10.1785/gssrl.80.5.682, 2009.
Armbruster, D., Mesimeri, M., Kästli, P., Diehl, T., Massin, F., and Wiemer, S.: SCDetect: Near real-time computationally efficient waveform cross-correlation based earthquake detection during intense earthquake sequences, EGU General Assembly 2022, Vienna, Austria, 23–27 May 2022, EGU22-12443, https://doi.org/10.5194/egusphere-egu22-12443, 2022.
Bayliss, K., Naylor, M., Kamranzad, F., and Main, I.: Pseudo-prospective testing of 5-year earthquake forecasts for California using inlabru, Nat. Hazards Earth Syst. Sci., 22, 3231–3246, https://doi.org/10.5194/nhess-22-3231-2022, 2022.
Bear, M. and Kradolfer, U.: An automatic phase picker for local and teleseismic events, Bull. Seismol. Soc. Am., 77, 1437–1445, 1987.
Bergamo, P., Hammer, C., and Fäh, D.: Correspondence between Site Amplification and Topographical, Geological Parameters: Collation of Data from Swiss and Japanese Stations, and Neural Networks-Based Prediction of Local Response, Bull. Seismol. Soc. Am., 112, 1008–1030, 2021.
Bergamo, P., Fäh, D., Panzera, F., Cauzzi, C., Glueer, F., Perron, V., Wiemer, S.: A site amplification model for Switzerland based on site-condition indicators and incorporating local response as measured at seismic stations, Bull. Earthq. Eng., 21, 5831–5865, https://doi.org/10.1007/s10518-023-01766-z, 2023.
Blagojević, N., Hefti, F., Henken, J., Didier, M., and Stojadinović, B.: Quantifying disaster resilience of a community with interdependent civil infrastructure systems, Struct. Infrastruct. Eng., 19, 1696–1710, https://doi.org/10.1080/15732479.2022.2052912, 2022.
Bodenmann, L., Reuland, Y., and Stojadinović, B.: Dynamic post-earthquake updating of regional damage estimates using Gaussian processes, Reliabil. Eng. Syst. Safe., 234, 109201, https://doi.org/10.1016/j.ress.2023.109201, 2023.
Bogris, A., Nikas, T., Simos, C., Simos, I., Lentas, K., Melis, N. S., Fichtner, A., Bowden, D., Smolinski, K., Mesaritakis, C., and Chochliournos, I.: Sensitive seismic sensors based on microwave frequency fiber interferometry in commercially deployed cables, Sci. Rep., 12, 14000, https://doi.org/10.1038/s41598-022-18130-x, 2022.
Böse, M., Heaton, T. H., and Hauksson, E.: Real-time Finite Fault Rupture Detector (FinDer) for large earthquakes, Geophys. J. Int., 191, 803–812, https://doi.org/10.1111/j.1365-246X.2012.05657.x, 2012.
Böse, M., Papadopoulos, A. N., Danciu, L., Clinton, J. F., and Wiemer, S.: Loss-Based Performance Assessment and Seismic Network Optimization for Earthquake Early Warning, Bull. Seismol. Soc. Am., 112, 1662–1677, https://doi.org/10.1785/0120210298, 2022.
Blagojević, N. and Stojadinović, B.: pyrecodes: an open-source library for regional recovery simulation and disaster resilience assessment of the built environment (v0.1.0), Chair of Structural Dynamics and Earthquake Engineering, ETH Zurich, Zurich, https://doi.org/10.5905/ethz-1007-700, 2023.
Burjánek, J., Gischig, V., Moore, J. R., and Fäh, D.: Ambient vibration characterization and monitoring of a rock slope close to collapse, Geophys. J. Int., 212, 297–310, 2018.
Cattania, C., Werner, M. J., Marzocchi, W., Hainzl, S., Rhoades, D., Gerstenberger, M., Liukis, M., Savran, W., Christophersen, A., and Helmstetter, A.: The forecasting skill of physics-based seismicity models during the 2010–2012 Canterbury, New Zealand, earthquake sequence, Seismol. Res. Lett., 89, 1238–1250, 2018.
Cauzzi, C. and Clinton, J.: A High- and Low-Noise Model for High-Quality Strong-Motion Accelerometer Stations, Earthq. Spectra, 29, 85–102, https://doi.org/10.1193/1.4000107, 2013.
Cauzzi, C., Edwards, B., Fäh, D., Clinton, J., Wiemer, S., Kästli, P., Cua, G., and Giardini, D.: New predictive equations and site amplification estimates for the next-generation Swiss ShakeMaps, Geophys. J. Int., 200, 421-438, https://doi.org/10.1093/gji/ggu404, 2015.
Cauzzi, C., Behr, Y., Le Guenan, T., Douglas, J., Auclair, S., Woessner, J., Clinton, J., and Wiemer, S.: Earthquake early warning and operational earthquake forecasting as real-time hazard information to mitigate seismic risk at nuclear facilities, Bull. Earthq. Eng., 14, 2495–2512, https://doi.org/10.1007/s10518-016-9864-0, 2016a.
Cauzzi, C., Sleeman, R., Clinton, J., Ballesta, J. D., Galanis, O., and Kästli, P.: Introducing the European Rapid Raw Strong-Motion Database, Seismol. Res. Lett., 87, 977–986, https://doi.org/10.1785/0220150271, 2016b.
Cauzzi, C., Clinton, J., Faenza, L., Heimers, S., Koymans, M. R., Lauciani, V., Luzi, L., Michelini, A., Puglia, R., and Russo, E.: Introducing a European integrated ShakeMap system, Seismol. Res. Lett., 89, 926, https://doi.org/10.1785/0220180082, 2018a.
Cauzzi, C., Fäh, D., Wald, D. J., Clinton, J., Losey, S., and Wiemer, S.: ShakeMap-based prediction of earthquake-induced mass movements in Switzerland calibrated on historical observations, Nat. Hazards, 92, 1211–1235, https://doi.org/10.1007/s11069-018-3248-5, 2018b.
Cauzzi, C. V., Clinton, J., Kaestli, P., Fäh, D., Bergamo, P., Böse, M., Haslinger, F., and Wiemer, S.: Swiss Shakemap at Fifteen: Distinctive Local Features and International Outreach, in: Seismological Society of America Annual Meeting (SSA 2022), Seismol. Res. Lett., 93, 1315–1316, https://doi.org/10.1785/0220220087, 2022.
Chiaraluce, L., Festa, G., Bernard, P., Caracausi, A., Carluccio, I., Clinton, J., Stefano, R., Elia, L., Evangelidis, C., Ergintav, S., Jianu, O., Kaviris, G., Marmureanu, A., Sebela, S., and Sokos, E.: The Near Fault Observatory community in Europe: a new resource for faulting and hazard studies, Ann. Geophys., 65, DM316, https://doi.org/10.4401/ag-8778, 2022.
Clinton, J., Cauzzi, C., Fäh, D., Michel, C., Zweifel, P., Olivieri, M., Cua, G., Haslinger, F., and Giardini, D.: The current state of strong motion monitoring in Switzerland, in: Earthquake Data in Engineering Seismology. Geotechnical, Geological, and Earthquake Engineering, vol. 14, edited by: Akkar, S., Gülkan, P., and van Eck, T., Springer, Dordrecht, https://doi.org/10.1007/978-94-007-0152-6_15, 2011.
Console, R. and Di Giovambattista, R.: Local earthquake relative location by digital records, Phys. Earth Planet. Inter., 47I, 43–49, https://doi.org/10.1016/0031-9201(87)90065-3, 1987.
Cremen, G. and Galasso, C.: Earthquake early warning: Recent advances and perspectives, Earth-Sci. Rev., 205, 103184, https://doi.org/10.1016/j.earscirev.2020.103184, 2020.
Crowley, H., Dabbeek, J., Despotaki, V., Rodrigues, D., Martins, L., Silva, V., Romão, X., Pereira, N., Weatherill, G., and Danciu, L.: European seismic risk model (ESRM20), EFEHR Technical Report, 2, EFEHR, https://doi.org/10.3929/ethz-b-000590388, 2021.
Cua, G. B.: Creating the Virtual Seismologist: Developments in Ground Motion Characterization and Seismic Early Warning, PhD dissertation, California Institute of Technology, https://doi.org/10.7907/M926-J956, 2005.
Dahmen, N. L., Clinton, J. F., Meier, M. A., Stähler, S. C., Ceylan, S., Kim, D., Stott, A. E., and Giardini, D.: MarsQuakeNet: A more complete marsquake catalog obtained by deep learning techniques, J. Geophys. Res.-Planets, 127, e2022JE007503, https://doi.org/10.1029/2022JE007503, 2022.
Dallo, I., Marti, M., Clinton, J., Böse, M., Massin, F., and Zaugg, S.: Earthquake early warning in countries where damaging earthquakes only occur every 50 to 150 years – The societal perspective, Int. J. Disast. Risk Reduct., 83, 103441, https://doi.org/10.1016/j.ijdrr.2022.103441, 2022a.
Dallo, I., Stauffacher, M., and Marti, M.: Actionable and understandable? Evidence-based recommendations for the design of (multi-)hazard warning messages, Int. J. Disast. Risk Reduct., 74, 102917, https://doi.org/10.1016/j.ijdrr.2022.102917, 2022b.
Danciu, L., Nandan, S., Reyes, C., Basili, R., Weatherill, G., Beauval, C., Rovida, A., Vilanova, S., Sesetyan, K., Bard, P.-Y., Cotton, F., Wiemer, S., and Giardini, D.: The 2020 update of the European Seismic Hazard Model: Model Overview, ETH Zurich, Zurich, https://doi.org/10.12686/a15, 2021.
Danciu, L., Weatherill, G., Rovida, A., Basili, R., Bard, P.-Y., Beauval, C., Nandan, S., Pagani, M., Crowley, H., Sesetyan, K., Villanova, S., Reyes, C., Marti, M., Cotton, F., Wiemer, S., and Giardini, D.: The 2020 European Seismic Hazard Model: Milestones and Lessons Learned, in: Progresses in European Earthquake Engineering and Seismology, ECEES 2022, Springer Proceedings in Earth and Environmental Sciences, Cham, https://doi.org/10.1007/978-3-031-15104-0_1, 2022.
Diehl, T., Husen, S., Kissling, E., and Deichmann, N.: High-resolution 3-D P-wave model of the Alpine crust, Geophys. J. Int., 179, 1133–1147, https://doi.org/10.1111/j.1365-246X.2009.04331.x, 2009.
Diehl, T., Kraft, T., Kissling, E., and Wiemer, S.: The induced earthquake sequence related to the St. Gallen deep geothermal project (Switzerland): Fault reactivation and fluid interactions imaged by microseismicity, J. Geophys. Res.-Solid, 122, 7272–7290, https://doi.org/10.1002/2017JB014473, 2017.
Diehl, T., Kissling, E., Herwegh, M., and Schmid, S.: Improving Absolute Hypocenter Accuracy with 3-D Pg and Sg Body-Wave Inversion Procedures and Application to Earthquakes in the Central Alps Region, J. Geophys. Res.-Solid, 126, e2021JB022155, https://doi.org/10.1029/2021jb022155, 2021a.
Diehl, T., Clinton, J., Cauzzi, C., Kraft, T., Kaestli, P., Deichmann, N., Massin, F., Grigoli, F., Molinari, I., and Böse, M.: Earthquakes in Switzerland and surrounding regions during 2017 and 2018, Swiss J. Geosci., 106, 543–558, https://doi.org/10.1007/s00015-013-0154-4, 2021b.
Diehl, T., Madritsch, H., Schnellmann, M., Spillmann, T., Brockmann, E., and Wiemer, S.: Seismotectonic evidence for present-day transtensional reactivation of the slowly deforming Hegau-Bodensee Graben in the northern foreland of the Central Alps, Tectonophysics, 846, 229659, https://doi.org/10.1016/j.tecto.2022.229659, 2023.
Dryhurst, S., Mulder, F., Dallo, I., Kerr, J. R., McBride, S. K., Fallou, L., and Becker, J. S.: Fighting misinformation in seismology: Expert opinion on earthquake facts vs. fiction, Front. Earth Sci., 10, 937055, https://doi.org/10.3389/feart.2022.937055, 2022.
Edwards, B., Allmann, B., Fäh, D., and Clinton, J.: Automatic computation of moment magnitudes for small earthquakes and the scaling of local to moment magnitude, Geophys. J. Int., 183, 407–420, https://doi.org/10.1111/j.1365-246X.2010.04743.x, 2010.
Edwards, B., Michel, C., Poggi, V., and Fäh, D.: Determination of Site Amplification from Regional Seismicity: Application to the Swiss National Seismic Networks, Seismol. Res. Lett., 84, 611–621, 2013.
Edwards, B., Kraft, T., Cauzzi, C., Kastli, P., and Wiemer, S.: Seismic monitoring and analysis of deep geothermal projects in St Gallen and Basel, Switzerland, Geophys. J. Int., 201, 1022–1039, https://doi.org/10.1093/gji/ggv059, 2015.
Faenza, L. and Michelini, A.: Regression analysis of MCS intensity and ground motion parameters in Italy and its application in ShakeMap, Geophys. J. Int., 180, 1138–1152, 2010.
Faenza, L. and Michelini, A.: Regression analysis of MCS intensity and ground motion spectral accelerations (SAs) in Italy, Geophys. J. Int., 186, 1415–1430, 2011.
Fäh, D., and Huggenberger, P.: INTERREG III Projekt: Erdbebenmikrozonierung am südlichen Oberrhein, Zusammenfassung, ETH Zurich, https://doi.org/10.3929/ethz-a-006412199, 2006.
Fäh, D., Giardini, D., Kästli, P., Deichmann, N., Gisler, M., Schwarz-Zanetti, G., Alvarez-Rubio, S., Sellami, S., Edwards, B., Allmann, B., Bethmann, F., Wössner, J., Gassner-Stamm, G., Fritsche, S., and Eberhard, D.: ECOS-09 Earthquake Catalogue of Switzerland Release 2011 Report and Database, Public catalogue, 17.4.2011, Report SED/RISK/R/001/20110417, Swiss Seismological Service ETH Zurich, http://www.seismo.ethz.ch/static/ecos-09/ECOS-2009_Report_final_WEB.pdf (last access: February 2024), 2011.
Fäh, D., Moore, J. R., Burjanek, J., Iosifescu, I., Dalguer, L., Dupray, F., Michel, C., Woessner, J., Villiger, A., Laue, J., Marshall,I., Gischig, V., Loew, S., Marin, A., Gassner, G., Alvarez, S., Balderer, W., Kästli, P., Giardini, D., Iosifescu, C., Hurni, L., Lestuzzi, P., Karbassi, A., Baumann, C., Geiger, A., Ferrari, A., Laloui, L., Clinton, J., and Deichmann, N.: Coupled seismogenic geohazards in Alpine regions, Bollettino di geofisica teorica ed applicata, 53, 485–508, https://doi.org/10.4430/bgta0048, 2012.
Fritsche, S., Fäh, D., and Schwarz-Zanetti, G.: Historical intensity VIII earthquakes along the Rhone valley (Valais, Switzerland): primary and secondary effects, Swiss J. Geosci., 105, 1–18, 2012.
Giardini, D.: The global seismic hazard assessment program (GSHAP) – 1992/1999, Ann. Geophys., 42, https://doi.org/10.4401/ag-3780, 1999.
Giardini, D., Wiemer, S., Fäh, D., and Deichmann, N.: Seismic hazard assessment of Switzerland, 2004, Publication Series of the Swiss Seismological Service, ETH Zurich, Zurich, 91 pp., http://www.seismo.ethz.ch/export/sites/sedsite/knowledge/.galleries/pdf_knowledge/SUIhaz2015_final-report_16072016_2.pdf_2063069299.pdf (last access: February 2024), 2004.
Giardini, D., Danciu, L., Erdik, M., Sesetyan, K., Demircioglu Tümsa, M. B., Akkar, S., Gülen, L., and Zare, M.: Seismic hazard map of the Middle East, Bull. Earthquake. Eng., 16, 3567–3570, https://doi.org/10.1007/s10518-018-0347-3, 2018.
Glueer, F., Häusler, M., Gischig, V., and Fäh, D.: Coseismic Stability Assessment of a Damaged Underground Ammunition Storage Chamber Through Ambient Vibration Recordings and Numerical Modelling, Front. Earth Sci., 9, 773155, https://doi.org/10.3389/feart.2021.773155, 2021.
Grigoli, F., Scarabello, L., Böse, M., Weber, B., Wiemer, S., and Clinton, J. F.: Pick- and waveform-based techniques for real-time detection of induced seismicity, Geophys. J. Int., 213, 868–884, https://doi.org/10.1093/gji/ggy019, 2018.
Guarini, M. R., Battisti, F., and Chiovitti, A.: A Methodology for the Selection of Multi-Criteria Decision Analysis Methods in Real Estate and Land Management Processes, Sustainability, 10, 507, https://doi.org/10.3390/su10020507, 2018.
Hammer, C., Ohrnberger, M., and Fäh, D.: Classifying seismic waveforms from scratch: a case study in the alpine environment, Geophys. J. Int., 192, 425–439, https://doi.org/10.1093/gji/ggs036, 2013.
Harte, D. S.: Evaluation of earthquake stochastic models based on their real-time forecasts: a case study of Kaikoura 2016, Geophys. J. Int., 217, 1894–1914, 2019.
Haslinger, F., Basili, R., Bossu, R., Cauzzi, C., Cotton, F., Crowley, H., Custodio, S., Danciu, L., Locati, M., Michelini, A., Molinari, I., Ottemöller, L., and Parolai, S.: Coordinated and interoperable seismological data and product services in Europe: the EPOS thematic core service for seismology, Ann. Geophys., 65, DM213, https://doi.org/10.4401/ag-8767, 2022.
Häusler, M., Gischig, V., Thöny, R., Glueer, F., and Donat, F.: Monitoring the changing seismic site response of a fast-moving rockslide (Brienz/Brinzauls, Switzerland), Geophys. J. Int., 229, 299–310, 2022.
Hengl, T., Heuvelink, G. B. M., and Rossiter, D. G.: About regression-kriging: From equations to case studies, Computers & Geosciences, 33(10), 1301–1315, 2007.
Hermann, M., Zechar, J. D., and Wiemer, S.: Communicating time-varying seismic risk during an earthquake sequence, Seismol. Res. Lett., 87, 301–312, https://doi.org/10.1785/0220150168, 2016.
Hetényi, G., Molinari, I., Clinton, J., Bokelmann, G., Bondár, I., Crawford, W. C., Dessa, J.-X., Doubre, C., Friederich, W., and Fuchs, F.: The AlpArray seismic network: a large-scale European experiment to image the Alpine Orogen, Surv. Geophys., 39, 1009–1033, https://doi.org/10.1007/s10712-018-9472-4, 2018.
Hillers, G., Husen, S., Obermann, A., Planès, T., Larose, E., and Campillo, M.: Noise-based monitoring and imaging of aseismic transient deformation induced by the 2006 Basel reservoir stimulation, Geophysics, 80, KS51–KS68, 2015.
Hobiger, M., Bergamo, P., Imperatori, W., Panzera, F., Lontsi, A. M., Perron, V., C., M., J., B., and Fäh, D.: Site Characterization of Swiss Strong-Motion Stations: The Benefit of Advanced Processing Algorithms, Bull. Seismol. Soc. Am., 111, 1713–1739, 2021.
Husen, S., Kissling, E., Deichmann, N., Wiemer, S., Giardini, D., and Baer, M.: Probabilistic earthquake location in complex three-dimensional velocity models: Application to Switzerland, J. Geophys. Res.-Solid, 108, 2077, https://doi.org/10.1029/2002JB001778, 2003.
Janusz, P., Bonilla, L. F., and Fäh, D.: URBASIS Deliverable: A case study on non-linear soil response in urban areas, ETC Zurich, Zurich, https://doi.org/10.3929/ethz-b-000575536, 2022.
Jousset, P., Currenti, G., Schwarz, B., Chalari, A., Tilmann, F., Reinsch, T., Zuccarello, L., Privitera, E., and Krawczyk, C. M.: Fibre optic distributed acoustic sensing of volcanic events, Nat. Commun., 13, 1753, https://doi.org/10.1038/s41467-022-29184-w, 2022.
Jozinović, D., Massin, F., Böse, M., and Clinton, J.: Combining earthquake early warning solutions from different algorithms: application to Switzerland, in: 2023 SSA Annual Meeting, 17–20 April 2023, San Juan, Puerto Rico, abstract 9451, 2023.
Klaasen, S., Paitz, P., Lindner, N., Dettmer, J., and Fichtner, A.: Distributed Acoustic Sensing in volcano-glacial environments – Mount Meager, British Columbia, J. Geophys. Res., 126, e2021JB022358, https://doi.org/10.1029/2021JB022358, 2021.
Kleinbrod, U., Burjánek, J., and Fäh, D.: Ambient vibration classification of unstable rock slopes: A systematic approach, Eng. Geol., 249, 198–217, 2019.
Kremer, K., Fabbri, S. C., Evers, F. M., Schweizer, N., and Wirth, S. B.: Traces of a prehistoric and potentially tsunamigenic mass movement in the sediments of Lake Thun (Switzerland), Swiss J. Geosci., 115, 1–20, 2022.
Lagomarsino, S. and Giovinazzi, S.: Macroseismic and mechanical models for the vulnerability and damage assessment of current buildings, Bull. Earthq. Eng., 4, 415–443, https://doi.org/10.1007/s10518-006-9024-z, 2006.
Lagomarsino, S., Cattari, S., and Ottonelli, D.: The heuristic vulnerability model: fragility curves for masonry buildings, Bull. Earthq. Eng., 19, 3129–3163, https://doi.org/10.1007/s10518-021-01063-7, 2021.
Lanza, F., Diehl, T., Deichmann, N., Kraft, T., Nussbaum, C., Schefer, S., and Wiemer, S.: The Saint-Ursanne earthquakes of 2000 revisited: evidence for active shallow thrust-faulting in the Jura fold-and-thrust belt, Swiss J. Geosci., 115, 1–24, https://doi.org/10.1186/s00015-021-00400-x, 2022.
Lestuzzi, P., Podestà, S., Luchini, C., Garofano, A., Kazantzidou-Firtinidou, D., and Bozzano, C.: Validation and improvement of Risk-UE LM2 capacity curves for URM buildings with stiff floors and RC shear walls buildings, Bull. Earthq. Eng., 15, 1111–1134, https://doi.org/10.1007/s10518-016-9981-9, 2017.
Lee, T.: The transition between Western and Central Alps: New seismotectonic insights from high-resolution earthquake catalogs and tomography, PhD thesis, Diss. No. 29073, ETH Zurich, Zurich, https://doi.org/10.3929/ethz-b-000613262, 2023.
Lee, T., Diehl, T., Kissling, E., and Wiemer, S.: New insights into the Rhône–Simplon fault system (Swiss Alps) from a consistent earthquake catalogue covering 35 yr, Geophys. J. Int., 232, 1568–1589, https://doi.org/10.1093/gji/ggac407, 2023.
Lindsey, N., Rademacher, H., and Ajo-Franklin, J.: On the broadband instrument response of fiber-optic (DAS) arrays, J. Geophys. Res., 125, e2019JB018145, https://doi.org/10.1029/2019JB018145, 2020.
Lomax, A., Virieux, J., Volant, P., and Berge, C.: Probabilistic earthquake location in 3D and layered models: Introduction of a Metropolis-Gibbs method and comparison with linear locations, in: Advances in Seismic Event Location, edited by: Thurber, C. H. and Rabinowitz, N., Kluwer, Amsterdam, 101–134, 2000.
Maranò, S., Reller, C., Loeliger, H.-A., and Fäh, D.: Seismic waves estimation and wave field decomposition: Application to ambient vibrations, Geophys. J. Int., 191, 175–188, 2012.
Marra, G., Clivati, C., Luckett, R., Tampellini, A., Kronjäger, J., Wright, L., Mura, A., Levi, F., Robinson, S., Xuereb, A., Baptie, B., and Calonico, D.: Ultrastable laser interferometry for earthquake detection with terrestrial and submarine cables, Science, 361, 486–490, 2018.
Martakis, P., Movsessian, A., Reuland, Y., Pai, S. G., Quqa, S., Garcia Cava, D., Tcherniak, D., and Chatzi, E.: A semi-supervised interpretable machine learning framework for sensor fault detection, Smart Struct. Syst. Int. J., 29, 251–266, 2022.
Martakis, P., Reuland, Y., Stavridis, A., and Chatzi, E.: Fusing damage-sensitive features and domain adaptation towards robust damage classification in real buildings, Soil Dynam. Earthq. Eng., 166, 107739, https://doi.org/10.1016/j.soildyn.2022.107739 2023.
Marti, M., Stauffacher, M., and Wiemer, S.: Anecdotal evidence is an insufficient basis for designing earthquake preparedness campaigns, Seismol. Res. Lett., 91, 1929–1935, https://doi.org/10.1785/0220200010, 2020.
Marti, M., Dallo, I., Roth, P., Papadopoulos, A. N., and Zaugg, S.: Illustrating the impact of earthquakes: Evidence-based and user-centered recommendations on how to design earthquake scenarios and rapid impact assessments, Int. J. Disast. Risk Reduct., 90, 103674, https://doi.org/10.1016/j.ijdrr.2023.103674, 2023.
Marzocchi, W., Lombardi, A. M., and Casarotti, E.: The establishment of an operational earthquake forecasting system in Italy, Seismol. Res. Lett., 85, 961–969, 2014.
Massin, F., Clinton, J., and Böse, M.: Status of Earthquake Early Warning in Switzerland, Front. Earth Sci., 9, 707654, https://doi.org/10.3389/feart.2021.707654, 2021.
Meier, M.-A., Ross, Z. E., Ramachandran, A., Balakrishna, A., Nair, S., Kundzicz, P., Li, Z., Andrews, J., Hauksson, E., and Yue, Y.: Reliable real-time seismic signal/noise discrimination with machine learning, J. Geophys. Res.-Solid, 124, 788–800, https://doi.org/10.1029/2018JB016661, 2019.
Mesimeri, M., Armbruster, D., Kästli, P., Scarabello, L., Diehl, T., Clinton, J., and Wiemer, S.: SCDetect: A SeisComP Module for Real‐Time Waveform Cross‐Correlation‐Based Earthquake Detection, Seismol. Res. Lett., https://doi.org/10.1785/0220230164, in press, 2024.
Michel, C., Edwards, B., Poggi, V., Burjánek, J., and Fäh, D.: Assessment of Site Effects in Alpine Regions through Systematic Site Characterization of Seismic Stations, Bull. Seismol. Soc. Am., 104, 2809–2826, 2014.
Michel, C., Fäh, D., Edwards, B., and Cauzzi, C. V.: Site amplification at the city scale in Basel (Switzerland) from geophysical site characterization and spectral modelling of recorded earthquakes, Phys. Chem. Earth, 98, 27–40, 2017.
Michelini, A., Faenza, L., Cauzzi, C., Lauciani, V., Clinton, J., Kästli, P., Haslinger, F., Wiemer, S., Melis, N., Theodoulidis, N., Böse, M., Weatherill, G., Cotton, F., and Giardini, D.: ShakeMap-EU: an update on the shakemap service in Europe, EGU General Assembly 2023, Vienna, Austria, 23–28 April 2023, EGU23-5937, https://doi.org/10.5194/egusphere-egu23-5937, 2023.
Mitchell-Wallace, K., Jones, M., Hillier, J., and Foote, M.: Natural catastrophe risk management and modelling, in: Natural catastrophe risk management and modelling: A practitioner's Guide, 1st Edn., John Wiley & Sons, Hoboken, NJ, https://doi.org/10.1002/9781118906057, 2017.
Mizrahi, L.: Towards Next Generation Time-Dependent Earthquake Forecasting, Doctoral dissertation, ETH Zurich, Zurich, https://doi.org/10.3929/ethz-b-000584646, 2022.
Mizrahi, L., Irina, D., and Kuratle, L. D.: D3.5 Development, Testing, and Communicating Earthquake Fore-casts: Current Practices and Expert Recommendations, https://www.research-collection.ethz.ch/bitstream/handle/20.500.11850/637239/3/Supplement_Developingtestingandcommunicatingearthquakeforecasts.pdf (last access: February 2024), 2023.
Molinari, I., Obermann, A., Kissling, E., Hetényi, G., Boschi, L., and AlpArray-Easi Working: 3D crustal structure of the Eastern Alpine region from ambient noise tomography, Results Geophys. Sci., 1–4, 100006, https://doi.org/10.1016/j.ringps.2020.100006, 2020.
Mousavi, S. M. and Beroza, G. C.: Deep-learning seismology, Science, 377, eabm4470, https://doi.org/10.1126/science.abm4470, 2022.
Nakata, N., Gualtieri, L., and Fichtner, A. (Eds.): Seismic ambient noise, Cambridge University Press, ISBN 9781108271745, 2019.
Nandan, S., Kamer, Y., Ouillon, G., Hiemer, S., and Sornette, D.: Global models for short-term earthquake forecasting and predictive skill assessment, Eur. Phys. J. Spec. Top., 230, 425–449, 2021.
Nievas, C., Crowley, C., Reuland, Y., Weatherill, G., Baltzopoulos, G., Bayliss, K., Chatzi, E., Chioccarelli, E., Gueìguen, P., Iervolino, I., Marzocchi, W., Naylor, M., Orlacchio, M., Pejovic, J., Popovic, N., Serafini, F., Serdar, N.: RISE deliverable 6.1: Integration of RISE Innovations in the Fields of OELF, RLA and SHM, http://static.seismo.ethz.ch/rise/deliverables/Deliverable_6.1.pdf (last access: February 2024), 2023.
Obermann, A., Planès, T., Larose, E., and Campillo, M.: Imaging preeruptive and coeruptive structural and mechanical changes of a volcano with ambient seismic noise, J. Geophys. Res.-Solid, 118, 6285–6294, 2013.
Obermann, A., Froment, B., Campillo, M., Larose, E., Planès, T., Valette, B., Chen, J. H., and Liu, Q. Y.: Seismic noise correlations to image structural and mechanical changes associated with the Mw 7.9 2008 Wenchuan earthquake, J. Geophys. Res.-Solid, 119, 3155–3168, 2014.
Obermann, A., Lupi, M., Mordret, A., Jakobsdóttir, S. S., and Miller, S. A.: 3D-ambient noise Rayleigh wave tomography of Snæfellsjjökull volcano, Iceland, J. Volancol. Geoth. Res., 317, 42–52, https://doi.org/10.1016/j.jvolgeores.2016.02.013, 2016.
Obermann Kraft, T., Larose, E., and Wiemer, S.: Potential of ambient seismic noise techniques to monitor the St. Gallen geothermal site (Switzerland), J. Geophys. Res.-Solid, 120, 4301–4316, 2015.
Ogata, Y.: Statistical models for earthquake occurrences and residual analysis for point processes, J. Am. Stat. Assoc., 83, 9–27, https://doi.org/10.1080/01621459.1988.10478560v, 1988.
Ogata, Y., Katsura, K., Falcone, G., Nanjo, K., and Zhuang, J.: Comprehensive and topical evaluations of earthquake forecasts in terms of number, time, space, and magnitude, Bull. Seismol. Soc. Am., 103, 1692–1708, 2013.
Pagani, M., Monelli, D., Weatherill, G., Danciu, L., Crowley, H., Silva, V., Henshaw, P., Butler, L., Nastasi, M., and Panzeri, L.: OpenQuake engine: An open hazard (and risk) software for the global earthquake model, Seismol. Res. Lett., 85, 692–702, https://doi.org/10.1785/0220130087, 2014.
Paitz, P., Edme, P., Gräff, D., Walter, F., Doetsch, J., Chalari, A., Schmelzbach, C., and Fichtner, A.: Empirical investigations of the instrument response for distributed acoustic sensing (DAS) across 17 octaves, Bull. the Seismol. Soc. Am., 111, 1–10, 2021.
Paitz, P., Lindner, N., Edme, P., Huguenin, P., Hohl, M., Sovilla, B., Walter, F., and Fichtner, A.: Phenomenology of avalanche recordings from distributed acoustic sensing, J. Geophys. Res., 128, e2022JF007011, https://doi.org/10.1029/2022JF007011, 2023.
Panzera, F., Bergamo, P., and Fäh, D.: Canonical Correlation Analysis Based on Site- Response Proxies to Predict Site- Specific Amplification Functions in Switzerland, Bull. Seismol. Soc. Am., 111, 1905–1920, 2021.
Panzera, F., Alber, J., Imperatori, W., Bergamo, P., and Fäh, D.: Reconstructing a 3D model from geophysical data for local amplification modelling: The study case of the upper Rhone valley, Switzerland, Soil Dynam. Earthq. Eng., 155, 107163, https://doi.org/10.1016/j.soildyn.2022.107163, 2022.
Papadopoulos, A. N., Böse, M., Danciu, L., Clinton, J., and Wiemer, S.: A framework to quantify the effectiveness of earthquake early warning in mitigating seismic risk, Earthq. Spectra, 39, 938–961, https://doi.org/10.1177/87552930231153424, 2023a.
Papadopoulos, A. N., Roth, P., Danciu, L., Bergamo, P., Panzera, F., Fäh, D., Cauzzi, C., Duvernay, B., Khodaverdian, A., Lestuzzi, P., Odabaşi, Ö., Fagà, E., Bazzurro, P., Marti, M., Valenzuela, N., Dallo, I., Schmid, N., Kästli, P., Haslinger, F., and Wiemer, S.: The Earthquake Risk Model of Switzerland ERM-CH23, EGUsphere [preprint], https://doi.org/10.5194/egusphere-2023-1504, 2023b.
Perron, V., Bergamo, P., and Fäh D.: Site Amplification at High Spatial Resolution from Combined Ambient Noise and Earthquake Recordings in Sion, Switzerland, Seismol. Res. Lett., 93, 2281–2298, 2022.
Poggi, V., Edward, B., and Fäh, D.: A comparative analysis of site-specific response spectral amplification models, Phys. Chem. Earth, 98, 16–26, https://doi.org/10.1016/j.pce.2016.09.001, 2017.
Racine, R., Cauzzi, C., Clinton, J., Roman, R.,. Cauzzi, C. V., Clinton, J. F., Fäh, D., Edwards, B., Diehl, T., Heimers, S., Deichmann, N., Kästli, P., Haslinger, F., and Wiemer, S.: Updated determination of earthquake magnitudes at the Swiss Seismological Service, EGU General Assembly 2020, 4–8 May 2020, online, https://doi.org/10.5194/egusphere-egu2020-8273, 2020.
Reuland, Y., Martakis, P., and Chatzi, E.: Damage-sensitive features for rapid damage assessment in a seismic context, in: 10th International Conference on Structural Health Monitoring of Intelligent Infrastructure, 30 June–2 July 2021, Porto, Portugal, 613–619, 2021.
Reuland, Y., Martakis, P., and Chatzi, E.: A Comparative Study of Damage-Sensitive Features for Rapid Data-Driven Seismic Structural Health Monitoring, Appl. Sci., 13, 2708, https://doi.org/10.3390/app13042708, 2023a.
Reuland, Y., Khodaverdian, A., Crowley, H., Nievas, C., Martakis, P., and Chatzi, E.: Monitoring-Driven Post-earthquake Building Damage Tagging, in: Experimental Vibration Analysis for Civil Engineering Structures, EVACES 2023, Lecture Notes in Civil Engineering, vol. 433, edited by: Limongelli, M. P., Giordano, P. F., Quqa, S., Gentile, C., and Cigada, A., Springer, Cham, https://doi.org/10.1007/978-3-031-39117-0_56, 2023b.
Roten, D., Fäh, D., and Bonilla, L. F.: Quantification of cyclic mobility parameters in liquefiable soils from inversion of vertical array records, Bull. Seismol. Soc. Am., 104, 3115–3138, 2014.
Sägesser, R. and Mayer-Rosa, D: Erdbebengefährdung in der Schweiz, Schweizerische Bauzeitung Heft 7, https://www.e-periodica.ch/digbib/view?pid=sbz-002:1978:96::78#1074 (last access: February 2024), 1978.
Sánchez-Pastor, P., Obermann, A., Schimmel, M., Weemstra, C., Verdel, A., and Jousset, P.: Short-and long-term variations in the Reykjanes geothermal reservoir from seismic noise interferometry, Geophys. Res. Lett., 46, 5788–5798, https://doi.org/10.1029/2019GL082352, 2019.
Savran, W. H., Werner, M. J., Marzocchi, W., Rhoades, D. A., Jackson, D. D., Milner, K., Field, E., and Michael, A.: Pseudoprospective Evaluation of UCERF3‐ETAS Forecasts during the 2019 Ridgecrest Sequence, Bull. Seismol. Soc. Am., 110, 1799–1817, https://doi.org/10.1785/0120200026, 2020.
Scarabello, L. and Diehl, T.: swiss-seismological-service/scrtdd, Zenodo [code], https://doi.org/10.5281/zenodo.5337361, 2021.
Scarabello, L., Diehl, T., Kästli, P., Clinton, J., and Wiemer, S.: Towards Real-Time Double-Difference Hypocenter Relocation of Natural and Induced Seismicity, EGU General Assembly 2020, Online, 4–8 May 2020, EGU2020-13058, https://doi.org/10.5194/egusphere-egu2020-13058, 2020.
Schorlemmer, D., Euchner, F., Kästli, P., Saul, J., and Group, Q. W.: QuakeML: status of the XML-based seismological data exchange format, Ann. Geophys., 54, 59–65, https://doi.org/10.4401/ag-4874, 2011.
SED – Swiss Seismological Service At ETH Zurich: National Seismic Networks of Switzerland, Other/Seismic Network, ETH Zurich, Zurich, https://doi.org/10.12686/SED/NETWORKS/CH, 1983.
SED – Swiss Seismological Service At ETH Zurich: Temporary deployments in Switzerland associated with glacier monitoring, Other, ETH Zurich, Zurich, https://doi.org/10.12686/SED/NETWORKS/4D, 1985.
SED – Swiss Seismological Service At ETH Zurich: Temporary deployments in Switzerland associated with aftershocks and other seismic sequences, Other, ETH Zurich, Zurich, https://doi.org/10.12686/SED/NETWORKS/8D, 2005.
SED – Swiss Seismological Service at ETH Zurich: GEOBEST Baseline Seismic Monitoring Networks for Deep Geothermal Energy Projects in Switzerland, Other/Seismic network, ETH Zurich, Zurich, https://doi.org/10.12686/SED/NETWORKS/G2, 2006.
SED – Swiss Seismological Service At ETH Zurich: Temporary deployments in Switzerland associated with landslides; ETH Zurich. Other. https://doi.org/10.12686/SED/NETWORKS/XP, 2012.
SED – Swiss Seismological Service at ETH Zurich: The Site Characterization Database for Seismic Stations in Switzerland, Federal Institute of Technology, Zurich, https://doi.org/10.12686/sed-stationcharacterizationdb, 2015.
SED – Swiss Seismological Service at ETH Zurich: Bedretto Underground Laboratory for Geosciences and Geoenergies (BULGG) Seismic Network, Switzerland, Other/Seismic network, ETH Zurich, Zurich, https://doi.org/10.12686/SED/NETWORKS/8R, 2018a.
SED – Swiss Seismological Service At ETH Zurich: Stations deployed for Risk Model Switzerland, Other, ETH Zurich, Zurich, https://doi.org/10.12686/SED/NETWORKS/XY, 2018b.
SED – Swiss Seismological Service at ETH Zurich: Temporary Deployments operated by industry partners of the Swiss Seismological Serrvice, typically associated with geothermal monitoring projects, Other/Seismic Network, ETH Zurich, Zurich, https://doi.org/10.12686/SED/NETWORKS/5A, 2021.
Shynkarenko, A., Kremer, K., Stegmann, S., Bergamo, P., Lontsi, A. M., Roesner, A., Hammerschmidt, S., Kopf, A., and Fäh, D.: Geotechnical characterization and stability analysis of subaqueous slopes in Lake Lucerne (Switzerland), Nat. Hazards, 113, 475–505, 2022.
SIA 261: Norm 261, Einwirkungen auf Tragwerke, SIA – Schweizerischer Ingenieur- und Architektenverein, Zurich, http://shop.sia.ch/normenwerk/architekt/sia 261/d/2020/D/Product (last access: February 2023), 2020.
Silva, V., Amo-Oduro, D., Calderon, A., Costa, C., Dabbeek, J., Despotaki, V., Martins, L., Pagani, M., Rao, A., and Simionato, M.: Development of a global seismic risk model, Earthq. Spectra, 36, 372–394, 2020.
Spica, Z., Perton, M., Martin, E., Beroza, G., and Biondi, B.: Urban seismic site characterization by fiber-optic seismology, J. Geophys. Res., 125, e2019JB018656, https://doi.org/10.1029/2019JB018656, 2020.
Spica, Z. J., Castellanos, J. C., Viens, L., Nishida, K., Akuhara, T., Shinohara, M., and Yamada, T.: Subsurface imaging with ocean-bottom distributed acoustic sensing and water phases reverberations, Geophys. Res. Lett., 49, e2021GL095287, https://doi.org/10.1029/2021GL095287, 2022.
Strader, A., Schneider, M., and Schorlemmer, D.: Prospective and retrospective evaluation of five-year earthquake forecast models for California, Geophys. J. Int., 211, 239–251, 2017.
Strollo, A., Cambaz, D., Clinton, J., Danecek, P., Evangelidis, C. P., Marmureanu, A., Ottemöller, L., Pedersen, H., Sleeman, R., and Stammler, K.: EIDA: The European integrated data archive and service infrastructure within ORFEUS, Seismol. Soc. Am., 92, 1788–1795, 2021.
Strupler, M., Hilbe, M., Kremer, K., Danciu, L., Anselmetti, F. S., Strasser, M., and Wiemer, S.: Subaqueous landslide-triggered tsunami hazard for Lake Zurich, Switzerland, Swiss J. Geosci., 111, 353–371, 2018.
Toledo, T., Obermann, A., Verdel, A., Martins, J. E., Jousset, P., Mortensen, A. K., Erbas, K., and Krawczyk, C. M.: Ambient seismic noise monitoring and imaging at the Theistareykir geothermal field (Iceland), J. Volcanol. Geoth. Res., 429, 107590, https://doi.org/10.1016/j.jvolgeores.2022.107590, 2022.
Valenzuela Rodríguez, N.: Die aktuelle Erdbebensituation der Schweiz visualisieren – Eine Analyse der Erdbebenkarte der MeteoSchweiz-App hinsichtlich ihrer Verständlichkeit und ihres Verbesserungspotenzials, MS thesis, ZHAW – Zurich University of Applied Sciences, Zurich, https://www.polybox.ethz.ch/index.php/s/vaBmjfUr0AgaVtS (last access: February 2024), 2021.
Van der Elst, N. J., Hardebeck, J. L., Michael, A. J., McBride, S. K., and Vanacore, E.: Prospective and retrospective evaluation of the US Geological Survey Public aftershock forecast for the 2019–2021 Southwest Puerto Rico Earthquake and aftershocks, Seismol. Soc. Am., 93, 620–640, 2022.
Van Stiphout, T., Wiemer, S., and Marzocchi, W.: Are short-term evacuations warranted? Case of the 2009 L'Aquila earthquake, Geophys. Res. Lett., 37, 1–5, https://doi.org/10.1029/2009GL042352, 2010.
Waldhauser, F.: Near-real-time double-difference event location using long-term seismic archives, with application to Northern California, Bull. Seismol. Soc. Am., 99, 2736–2748, https://doi.org/10.1785/0120080294, 2009.
Waldhauser, F. and Ellsworth, W.: A Double-Difference Earthquake Location Algorithm: Method and Application to the Northern Hayward Fault, California, Bull. Seismol. Soc. Am., 90, 1353–1368, https://doi.org/10.1785/0120000006, 2000.
Walter, F., Gräff, D., Lindner, N., Paitz, P., Köpfli, M., Chmiel, M., and Fichtner, A.: Distributed Acoustic Sensing of microseismic sources and wave propagation in glaciated terrain, Nat. Commun., 11, 2436, https://doi.org/10.1038/s41467-020-15824-6, 2020.
Wiemer, S., Danciu, L., Edwards, B., Marti, M., Fäh, D., Hiemer, S., Wössner, J., Cauzzi, C., Kästli, P., and Kremer, K.: Seismic Hazard Model 2015 for Switzerland (SUIhaz2015), ETH Zurich, Zurich, https://doi.org/10.12686/a2, 2016.
Wiemer, S., Papadopoulos, A., Roth, P., Danciu, L., Bergamo, P., Fäh, D., Duvernay, B., Khodaverdian, A., Lestuzzi, P., Odabaşi, Ö., Fagà, E., Bazzuro, P., Cauzzi, C., Hammer, C., Panzera, F., Perron, V., Marti, M., N., V., Dallo, I., Zaugg, S., Fulda, D., Kästli, P., Schmid, N., and Haslinger, F.: Earthquake Risk Model of Switzerland (ERM-CH23), Swiss Seismological Service, ETH Zurich, Zurich, https://doi.org/10.12686/a20, 2023.
Woessner, J., Hainzl, S., Marzocchi, W., Werner, M. J., Lombardi, A. M., Catalli, F., Enescu, B., Cocco, M., Gerstenberger, M. C., and Wiemer, S.: A retrospective comparative forecast test on the 1992 Landers sequence, J. Geophys. Res.-Solid, 116, B05305, https://doi.org/10.1029/2010JB007846, 2011.
Woessner, J., Danciu, L., Giardini, D., Crowley, H., Cotton, F., Grünthal, G., Valensise, G., Arvidsson, R., Basili, R., Demircioglu, M. B., Hiemer, S., Meletti, C., Musson, R. W., Rovida, A., Sesetyan, K., and Stucchi, M.: The 2013 European Seismic Hazard Model: key components and results, Bull. Earthq. Eng., 13, 3553–3596, https://doi.org/10.1007/s10518-015-9795-1, 2015.
Worden, C. B., Thompson, E. M., Hearne, M., and Wald, D. J.: ShakeMap Manual Online: technical manual, user's guide, and software guide, US Geological Survey, https://doi.org/10.5066/F7D21VPQ, 2020.