the Creative Commons Attribution 4.0 License.
the Creative Commons Attribution 4.0 License.
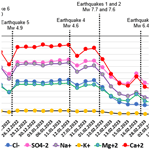
Spring water anomalies before two consecutive earthquakes (Mw 7.7 and Mw 7.6) in Kahramanmaraş (Türkiye) on 6 February 2023
Hasan Çetin
Nurettin Yakupoğlu
Understanding earthquake phenomena is always challenging. The search for reliable precursors of earthquakes is important but requires systematic and long-time monitoring employing multi-disciplinary techniques. In search of possible precursors, we obtained commercially bottled spring waters dated before and after the earthquakes of 6 February 2023. Hydrogeochemical precursors have been detected in commercially bottled natural spring waters (Ayran Spring and Bahçepınar Spring), which are at a distance of about 100 and 175 km from the epicenters of the Mw 7.7 and Mw 7.6 Kahramanmaraş (Türkiye) earthquakes of 6 February 2023, respectively. The available water samples cover the period from March 2022 to March 2023. The pre-earthquake anomaly is characterized by an increase in electrical conductivity and major ions (Ca2+, Mg2+, K+, Na+, Cl−, and SO) compared to the background for Ayran Spring water samples. The pre-earthquake anomaly lasted for at least 6 months. The anomaly in major ions sharply declined and the ion content approached the background values about 2 weeks after the earthquakes. Although only 6.5 km away from the Ayran Spring, the bottled water samples of the Bahçepınar Spring did not show any anomalies in electrical conductivity; therefore, the samples were not analyzed for ion content. Bahçepınar water is collected from shallow boreholes dug into alluvial deposits, which we believe are decoupled from the basement rocks, and this may be the reason for the lack of abnormal water chemistry prior to the earthquakes. This attests to the fact that sampling locations are very important in the detection of possible earthquake precursors. Results of the Ayran spring water samples indicate that spring water chemical anomalies of discrete samples may provide valuable information on pre-earthquake crustal deformation. Monitoring of spring waters, along with other monitoring techniques in a multidisciplinary network, and for a sufficiently long time, could potentially enable obtaining reliable proxy indicators of pre-earthquake crustal deformation.
- Article
(5464 KB) - Full-text XML
- BibTeX
- EndNote
Two devastating earthquakes (Mw 7.7 and Mw 7.6) struck the Kahramanmaraş area in southern Turkey on 6 February 2023; the earthquakes occurred about 9 h apart. The earthquakes caused devastation, causing more than 50 000 deaths and leaving behind thousands of injured and/or homeless. Earthquakes of destructive magnitudes (e.g., M > 7) are naturally expected to occur at plate boundary settings (Fig. 1), and Kahramanmaraş province is at the junction of the East Anatolian Fault System (EAFS) and the Dead Sea Fault System (DAFS). However, the reason why such natural events turn into disasters is mainly due to a lack of preparedness. Where buildings are not built to be sufficiently earthquake-resistant, monitoring of crustal deformation and searching for reliable pre-earthquake signals become more important. This is obviously a big challenge for earth scientists to overcome. Although there is still a long way to go on this front, the scientific literature is full of scattered but promising and encouraging cases.
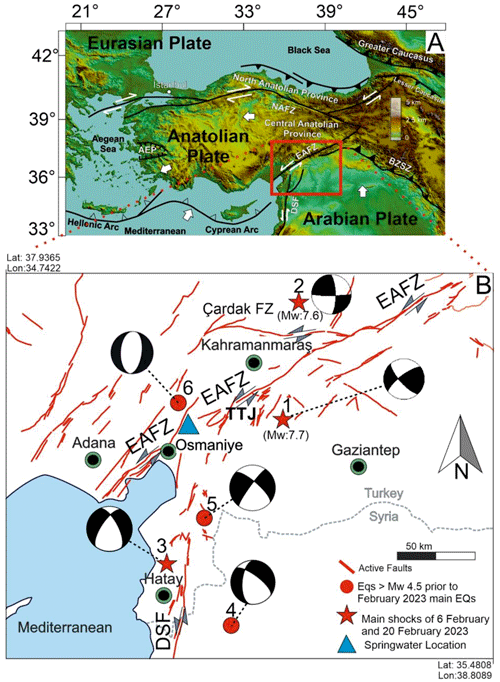
Figure 1(a) Neotectonics map of Türkiye and surroundings (compiled from Sengör and Yılmaz, 1981; Sengör et al., 1985; Hancock and Barka, 1987; Şaroğlu et al., 1992a; Barka and Reilinger, 1997; Bozkurt, 2001). (b) Active fault map of the region affected by the February 2023 earthquakes (Perinçek and Çemen, 1990; Şaroğlu et al., 1992a; Cetin et al., 2003). Red starts show the epicenters of the Mw 7.7 and Mw 7.6 Kahramanmaraş earthquakes of 6 February 2023 and Mw 6.4 Hatay earthquake of 20 February 2023. Filled red circles show the locations of the earthquakes (Mw > 4.5) that occurred in the area (circle area with a radius of 150 km from the location of the water spring) between September 2022 and 5 February 2023. Details of the earthquakes are given in Table 1. TTJ is Türkoğlu Triple Junction. Beach balls are fault plane solutions of earthquakes and were obtained from the Boğaziçi University Kandilli Observatory and Earthquake Research Institute (KOERI) of Turkey (http://www.koeri.boun.edu.tr/sismo/zeqdb/, last access: 20 July 2023).
Earthquakes are complex natural phenomena, and their predictions have been long viewed as difficult, if not impossible (e.g., Geller et al., 1997). Geochemical observations to identify earthquake precursors were initiated in the late 1960s (Rikitake, 1979; Wakita, 1996). Reviewing 20 years of relevant data Turcotte (1991) concluded that large earthquakes are not preceded by reliable seismic precursors. Moreover, Geller et al. (1997) claimed that earthquakes can never be predicted. However, for the last few decades, there have been numerous reports of ground-based anomalies (e.g., changes in soil gas, water chemistry and groundwater level, rock deformation detected by tiltmeters, electrical and electromagnetic fields) preceding major earthquakes (including but not limited to Rikitake, 1979; Dobrovolsky et al., 1979; Birchard and Libby, 1978; Hauksson, 1981; Wakita et al., 1988; Sultankhodhaev, 1984; Thomas et al., 1986; Rikitake, 1987; Martinelli and Ferrari, 1991; Etiope et al., 1997; Vallianatos and Tzanis, 1998; Bella et al., 1998; Virk and Singh, 1993; King et al., 1995; Planinic et al., 2004; Claesson et al., 2004; Vallianatos et al., 2004; Hartmann and Levy, 2006; Papadopoulos et al., 2006; Uyeda et al., 2008; İnan et al., 2008; Walia et al., 2009; İnan et al., 2010; İnan et al, 2012a, b, c; Skelton et al., 2014, 2019; Barberio et al., 2017; He and Singh 2019; Ouzounov et al., 2021; Gori and Barberio, 2022; Xiang and Peng, 2023). Compiling a review of claimed precursors, Cicerone et al. (2009) conducted a survey of published scientific literature on earthquake precursors and concluded that precursory anomalies seem to be recorded where there is modern instrumentation. İnan et al. (2010, 2012a) provided hints to select monitoring sites. Recently, Conti et al. (2021) provided a short review of ground-based observations before earthquakes
Hydro-geochemical anomalies observed prior to seismic events are generally interpreted to be related to the alteration of the groundwater circulating system by the changes in the crustal stress/strain before earthquakes and mixing of different aquifers (e.g., Scholz et al., 1973; Nur, 1974; Sibson et al., 1975; Sugisaki et al., 1996; Tsunogai and Wakita, 1995; Toutain et al., 1997; Claesson et al., 2004; Pérez et al., 2008; İnan et al., 2010; Grant et al., 2011; İnan et al., 2012c; Doglioni et al., 2014; Ingebritsen and Manga, 2014; Skelton et al., 2014, 2019; Barberio et al., 2017; Gori and Barberio, 2022; Xiang and Peng, 2023). However, another different approach based on “stress-activated positive hole currents” has been suggested to play a role in the development of physicochemical pre-earthquake stress indicators (Freund, 2011; Paudel et al., 2018)
As suggested by Nur (1974) and later by Rikitake (1987), precursory phenomena may have a common physical basis which Scholz et al. (1973) called the “dilatation and water diffusion (DWD) model”. Roeloffs (1996) noted that with respect to earthquake hydrology, mechanical and fluid-dynamic effects can be modeled using poroelasticity. More recently, the DWD model has been explained further (e.g., Doglioni et al., 2014; Wang and Manga, 2021). However, other authors have proposed a fundamentally different approach (Freund et al., 2006; Freund, 2008, 2011; Paudel et al., 2018) to study and evaluate physicochemical pre-earthquake stress indicators. Until the mechanism controlling pre-earthquake processes is fully understood, it is worth noting that the success of any pre-earthquake stress indicators may be compromised by the ever-present crustal heterogeneity, anisotropy, and/or crustal blocks (Areshidze et al., 1992; Tansi et al., 2005; Sol et al., 2007; İnan et al., 2012a; Yu et al., 2023). Microplate and/or block boundaries are obstacles to pre-earthquake strain to transfer from one block to the other (İnan et al., 2012a; Yu et al., 2023).
A multi-disciplinary earthquake observation network (GPS, seismology, soil radon, and spring water monitoring stations) was established in Kahramanmaraş and surrounding provinces along the fault zones (Adana, Hatay, Malatya, Elazığ, Bingöl) in 2007 under the scope of the TURDEP Project (İnan et al., 2007). In the Kahramanmaraş area, due to seismic gap interpretations, also borehole tilt monitoring stations were established. Continuous monitoring was continued until the middle of 2012, and valuable multi-disciplinary data were collected (İnan et al., 2010; 2012a, b, c). However, throughout these 5 years, no earthquake of significant magnitude (e.g., M > 6) occurred to test the usefulness of the monitoring network, and the project was terminated by the funding organization due mainly to a lack of vision. As a result, the earth science community was caught unprepared when two consecutive devastating earthquakes struck the area on 6 February 2023. No ground (except GPS and seismology) monitoring station data were available to detect possible pre-earthquake anomalies. However, following the Mw 7.7 and Mw 7.6 Kahramanmaraş earthquakes, we searched for bottled spring waters to analyze in search of possible pre-earthquake anomalies. This proved difficult as the water supply to the large community affected by the earthquakes was quite limited, and businesses providing bottled spring waters were also mostly shut down. Finally, we were able to obtain commercially bottled water samples (dated before and after the earthquake) from the Ayran and Bahçepınar springs, which are located within about a 6.5 km distance in the Osmaniye Province. The spring waters are about 100 and 175 km from the epicenter of the first (Mw 7.7) and the second (Mw 7.6) earthquakes, respectively (Fig. 1b). In this study, we conducted electrical conductivity (Ec) measurements on bottled waters, and based on the Ec results, we selected samples for analysis of major ions in water in search of pre-earthquake anomalies. The spring water samples cover the range from March 2022 to March 2023.
Kahramanmaraş region is located in the suture zone formed by the collision between Arabian and Anatolian plates during Late Miocene–Pliocene (Fig. 1a). After this collision, very important strike-slip fault zones were developed in the Anatolian plate due to the continuous northward movement of the Arabian plate and the resulting westward movement or escape of the Anatolian plate along two major fault zones, the North Anatolian Fault Zone (NAFZ) and the East Anatolian Fault Zone (EAFZ) (Ketin, 1948; McKenzie, 1972; Dewey and Şengör, 1979; Şengör and Yılmaz, 1981; Hempton, 1982; Şengör et al., 1985).
The East Anatolian Fault Zone (EAFZ) is an approximately 550 km long, northeast–southwest trending, sinistral strike-slip fault (Fig. 1a). It was first described by Allen (1969) and mapped by Arpat and Şaroğlu (1972). The EAFZ starts from Karlıova Triple Junction in the northeast, and it runs in the southwest direction, passes near the east–southeast of Kahramanmaraş, and joins another triple junction at Türkoğlu (TTJ in Fig. 1b). The EAFZ then continues to the Hatay in the south direction to merge into the Dead Sea Fault Zone (DSFZ) (Allen, 1969; Arpat and Şaroğlu, 1972; Dewey and Sengör, 1979; Rotstein, 1984; Şengör et al., 1985; Kelling et al., 1987; Şaroğlu et al., 1992a, b; Cetin et al., 2003; Yönlü et al., 2017). There are different interpretations, however, for the remainder of the fault zone after Türkoğlu Triple Junction (marked as TTJ in Fig. 1b). Some studies extend the fault zone southwesterly to the Mediterranean Sea (McKenzie, 1972; Dewey et al., 1973; Jackson and McKenzie, 1984; Barka and Kadinsky-Cade, 1988; Karig and Kozlu, 1990; Kempler and Garfunkel, 1991; Westaway and Arger, 1996), joining it with the Cyprian Arc, along which the convergence takes place between the African and Anatolian plates (McKenzie, 1976; Dewey and Şengör, 1979). Others think that the fault zone ends around the TTJ (Lovelock, 1984; Chorowicz et al., 1994). According to Muehlberger and Gordon (1987), the EAFZ becomes the northern branch of the DSFZ.
The seismicity of the study area is controlled by a complex interaction of the African, Arabian, and Eurasian plates (McKenzie, 1972). The seismicity of the EAFZ has been minimal for most of the last 100 years (Ambraseys, 1989). Historical earthquake records show that Kahramanmaraş and its surroundings were affected by two major earthquakes in AD 1114 and AD 1513 (Soysal et al., 1981; Ambraseys, 1989). There had been a long quiescence of more than 500 years in the Kahramanmaraş area before the Mw 7.7 and Mw 7.6 earthquakes struck on 6 February 2023. About 1 year before these earthquakes occurred, the area had been seismically quiet as suggested by only a few M > 4.5 earthquakes occurring in a circular area with a radius of 150 km, taking the Ayran spring water as the center (Fig. 1b and Table 1). The fault plane solutions (FPSs) for earthquakes nos. 3, 4, and 5 suggest mainly normal faulting, whereas, for others (earthquakes nos. 1, 2, and 6), FPSs suggest movement on dominantly left lateral strike-slip faults (Fig. 1b) as expected for left-lateral strike-slip nature of the EAFZ.
Table 1Earthquake times, magnitudes, and locations as received from http://www.koeri.boun.edu.tr/sismo/zeqdb/ (last access: 20 July 2023). Earthquakes 1, 2, and 3 are the earthquakes of February 2023. Earthquakes 4, 5, and 6 are those that have occurred in the circular area (with a radius of 150 km from the Ayran spring water location) between 1 September 2022 and 5 February 2023. The locations of these earthquakes are given on the map (Fig. 1b).
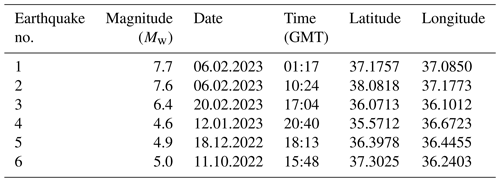
3.1 Spring water samples
The spring water samples were received in commercial polyethylene bottles and brought to Istanbul Technical University Laboratory for electrical conductivity measurements and major ion analyses. Some of the samples had been bottled up to several months before the analyses. However, this does not create any concern because much longer storage in this kind of bottle has been reported to be appropriate in terms of keeping reliable concentrations (Tsunogai and Wakita, 1995; İnan et al., 2012c; Rapti et al., 2023).
The spring water samples cover the range from March 2022 to March 2023. It is worth noting that the oldest sample predating the earthquakes was AYR 1 (dated 8 March 2022) from the Ayran Spring. Other bottled water samples we could obtain from both springs were dated between September 2022 and March 2023. In fact, we could not obtain any samples dated between 8 March and 14 September 2022. The samples from September, October, and November 2022 are limited but from December 2022 to January 2023, available samples are several per month (Table 2).
Table 2Ec and major ion analysis results for the Ayran (AYR) and the Ec analysis results for the Bahçepınar (BPN) bottled waters. The data for Ca2+, Mg2+, K+, Na+, Cl− and SO for the AYR samples are plotted in Figure 3B. Standard deviation (2σ) has been computed considering cations/anions contents of samples dated from 15 February to 31 March 2023: the period which is considered to nearly represent background concentrations of the water. These samples are marked in bold fonts. Italic fonts are for the values obtained from the analyses of the data.
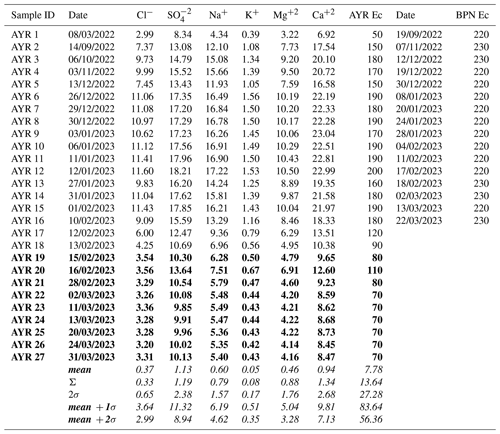
3.2 Spring water analysis
We first screened the bottled water samples by conducting electrical conductivity (Ec) measurements, and based on the results, we selected samples for analysis of major ions.
Samples of the AYR spring water were analyzed by ion chromatography as discussed by Zeyrek et al. (2010). Briefly, the samples were filtered at 0.45 µm and split into two portions before analysis using an ion chromatography instrument (Dionex ICS-1000). Sodium carbonate and methane–sulfonic acid were used as eluents for anion and cation analyses, respectively. For calibration, DIONEX Certified Reference Standards were used. Deionized water with a resistance better than 18.2 MΩ was used for the preparation of all eluents. Repeated measurements ascertained that the analytical uncertainties for all anions and cations were below 5 %. Electrical conductivity (Ec) measurements for the bottled Bahçepınar (BPN) spring water samples and both Ec and ion analysis results for the bottled Ayran spring waters are listed in Table 2.
3.3 Statistical analysis of the data
For the statistical treatment of the data on major ion contents of the water samples, we calculated the weighted average (weighted compared to the analytical error for each point) and computed the 2σ external error (2×αe) from the following equation:
where x is the average and σ the analytical error on each measured point. The 2σ external error (αe) considers the general variability of all datasets and the analytical error on each point; thus, we obtained the total error envelope for the samples that we consider representing background (from 15 February to 31 March 2023; see Table 2 and Fig. 3b).
3.4 Relation between earthquake magnitude, distance, and precursory duration
Slightly different relations between earthquake magnitude, duration of a precursory anomaly, and the distance of the monitoring site to the earthquake epicenter have been proposed. Dobrovolsky et al. (1979) proposed a theoretical relation between earthquake magnitude and maximum epicentral distance at which geochemical anomalies may be observed.
Relation (1) assumes a homogenous and isotropic crust, where M is the earthquake magnitude and D is the distance in kilometers to the earthquake epicenter (Dobrovolsky et al., 1979). Rikitake (1987) noted a slightly different relation:
where a and b are constants, T is the duration of anomaly and M is the magnitude of an earthquake (Rikitake, 1987). Moreover, Sultankhodhaev (1984) also reported a relation, between earthquake magnitude, the distance of the monitoring site to the earthquake epicenter and duration of precursory anomaly:
where D is the distance in kilometers, T is the duration of a precursory anomaly in days, and M is earthquake magnitude; b is a constant taken as 0.15 (Sultankhodhaev, 1984).
All of these three relations provide a helpful initial idea about what to expect of precursory anomalies in terms of duration and distance to the earthquake epicenter. The closer the epicenter of an earthquake of a given magnitude, the longer the duration of anomaly at a monitoring site.
Obviously, we should keep in mind that the relations proposed by Dobrovolsky et al. (1979), Sultankhodhaev (1984), and Rikitake (1987) assume homogenous and isotropic crust where there is pre-earthquake stress and resultant strain propagates in all directions. In fact, we know that this assumption is not totally correct as microplates and/or block boundaries hinder stress transfer (e.g., İnan et al., 2012b). This issue should be seriously considered and care should be exercised.
İnan et al. (2008, 2010) verified Dobrovolsky et al.'s (1979) relation for medium-size earthquakes (M < 5.3). Accordingly, for an earthquake of magnitude 4.5, the maximum distance for detection of possible geochemical anomalies in the Ayran spring water will be about 100 km. For contingency, we took a 150 km radius and listed in Table 1 the earthquakes with M > 4.5 occurring between September 2022 and 5 February 2023 in order to compare with the water geochemical data we obtained in this study.
The bottled water samples from the Bahçepınar (BPN) Spring did not show any meaningful (e.g., significant) variations in electrical conductivity (Ec) values, varying in a narrow range between 220 and 230 µS cm−1 (Table 2). Therefore, these samples were not analyzed for major ion content because change (increase/decrease) of major ions contents is expected to result in Ec variation (İnan et al., 2010, 2012c). However, the bottled water samples from the Ayran (AYR) Spring showed major variations in the Ec values, varying in range between 50 and 200 µS cm−1. Therefore, the AYR samples were analyzed for major ions. Possible reasons for not detecting any anomaly in the Ec measurements of the BPN spring water samples have been investigated. The investigation suggests that the reason may be the geological environment of the springs. The AYR spring water emanates from Middle–Upper Ordovician age metamorphic rocks (Kardere Formation) made up of quartzite, metasandstone, metasiltstone, and metashale (Usta et al., 2015, 2017), whereas the BPN spring water is collected from shallow boreholes dug into valley-filling Quaternary age alluvial deposits that are underlain by ophiolite (Fig. 2). The alluvial deposits reach a thickness of about 200 m, and the water reservoir within the alluvium deposit is fed by precipitation and a nearby Bekdemir stream flowing towards the alluvial deposit. It is interesting that the streams disappear to the south, suggesting that the stream (creek) water is captured by the alluvial deposit. Since the BPN water is collected from shallow boreholes (less than 100 m) dug into alluvial deposits, we believe that the alluvial deposits are decoupled from the basement rocks (which undergo pre-earthquake stress), and this may be the reason for the lack of anomaly in water chemistry prior to the earthquakes. This has testified again to the importance of adequate geological knowledge of the area before sampling discrete geochemical samples (water or soil gas) and/or continuous monitoring in search of pre-earthquake signals (İnan et al., 2008, 2010).
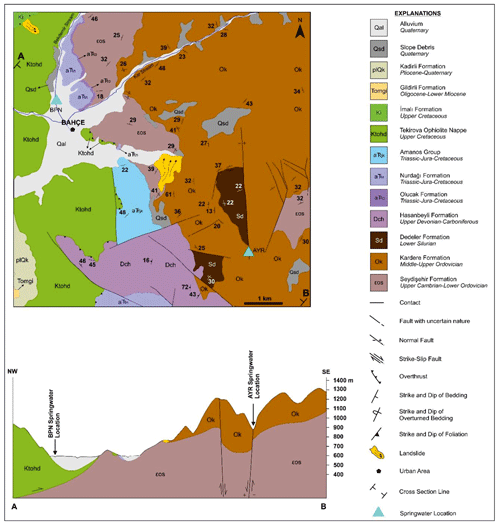
Figure 2Locations and local geology of the water springs (modified from Usta et al., 2015, 2017). The Ayran spring water emanates from a fault in the metamorphic Kardere Formation (blue triangle shown at the lower right in the map), whereas the Bahçepınar spring water is obtained from the Quaternary alluvium (blue triangle shown at the upper left in the map).
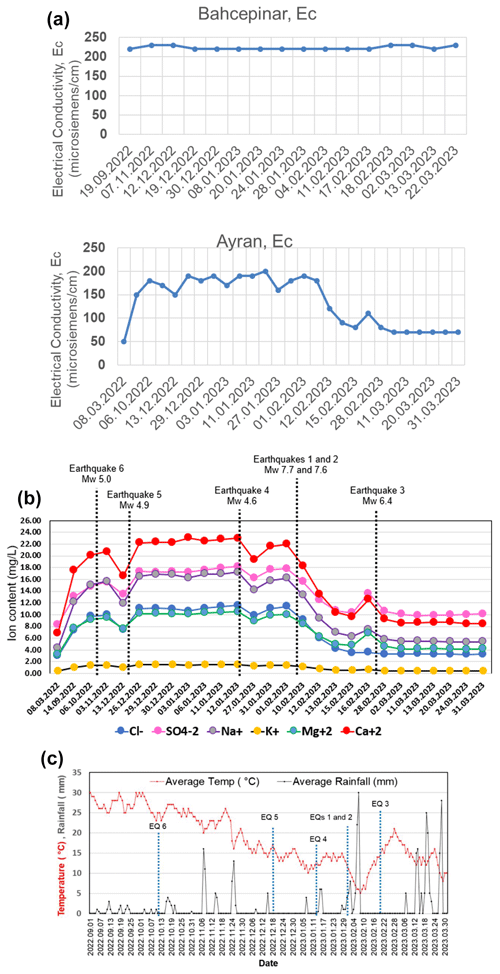
Figure 3Time variation graphs of Ec for the Ayran (AYR) and the Bahçepınar (BPN) bottled waters (a) and major ions for the AYR bottled waters (b). All data are listed in Table 2. Daily average air temperature and rainfall at the Osmaniye meteorology station (37∘07′ N, 36∘25′ E; 32 km SW of the Ayran Spring) between 1 September 2022 and 30 March 2023 (c) (https://www.meteoblue.com/tr/hava/historyclimate/weatherarchive/osmaniye_turkiye_303195, last access: 20 July 2023). Earthquakes 1 through 6 are the earthquakes listed in Table 1.
Variations of major ions in the AYR spring water samples were significant. It is clear that pre-earthquake anomalies have exceeded the αe (Fig. 3b and Table 2). Before any interpretation, we needed to make sure that geochemical time series are not affected by meteorological conditions. In this context, meteorological data were obtained from the Osmaniye Meteorology Station (located about 32 km SW of the AYR spring), and the daily average air temperature and rainfall are shown in Fig. 3c.
Air temperature gradually decreases from about 30 ∘C in September 2022 to less than 10 ∘C in February 2023 (Fig. 3c). Daily rainfall is noticeably present in November 2022 and March 2023. Normally, variations in air temperature are not expected to affect the chemical contents of the spring water (İnan et al., 2010, 2012), but the effect of rainfall on soil radon concentration is dominant (İnan et al., 2008, 2010, 2012b; Seyis et al., 2022). All earthquakes listed in Table 1 are plotted on the meteorology time series in Fig. 3c, and this shows that major and heavy rainfall took place right after the devastating earthquakes of 6 February 2023. Based on the relatively low EC and low major ion contents of the AYR spring water (Table 2) that is bottled and commercially distributed, it can be said that this water is of shallow origin (Di Luccio et al., 2018). A comparison of the geochemical time series and significant variations shown in Fig. 3b and the daily average rainfall data shown in Fig. 3c reveals no correlations. İnan et al. (2010, 2012) compared meteorological time series with hydrogeochemical time series and noted that meteorological conditions do not seem to play a role in water's major ion contents. In this study, we compare rainfall data and geochemical time series (Fig. 3) and, as there is no correlation, we conclude that the increase of major ion contents observed in AYR spring waters is not related to atmospheric variations (e.g., rainfall). Therefore, it is safe to conclude that the chemical changes recorded in the spring water must be related to crustal deformation associated with earthquake stress buildup.
As shown in Fig. 3b, changes in the concentration of the major ionic species dissolved in the AYR spring water were observed. Positive anomalies are recorded in the Ca2+, Mg2+, K+, Na+, Cl−, and SO contents (mg L−1) before the 6 February Mw 7.7 and Mw 7.6 Kahramanmaraş earthquakes (Fig. 3b; Table 2). These positive anomalies (increase in dissolved ion content) started as early as September 2022, suggesting a pre-earthquake anomaly of nearly 6 months. Considering Sultankhodhaev's (1984) relation (log (DT) = ) between earthquake magnitude, precursory anomaly duration, and the distance of the earthquake epicenter to the monitoring site, such a long duration (6 months) of a precursory anomaly we report in this study is very likely because the magnitudes of the 7.7 and 7.6 devastating earthquakes are sufficiently big to cause such a long precursory anomaly at a location about 100 km from the epicenter. Considering the relation proposed by Sultankhodhaev (1984), such a magnitude of the earthquake theoretically should lead to a months-long precursory anomaly in the geochemical parameters at locations hundreds of kilometers away from the epicentral area. For instance, in western Turkey, İnan et al. (2010) reported 32 d of chemical anomaly at a water monitoring site located 32 km from the epicenter of a Mw 4.2 earthquake.
In regard to changes in the dissolved ions in the AYR spring water, the following changes are prominent. The Ca2+ and Na+ contents increase (for the period between September 2022 to 15 February 2023) above the background by about 14 and 10 mg L−1, respectively, and reach up to 22 and 16 mg L−1, respectively. This increase started about 6 months before the 6 February earthquakes (earthquakes 1 and 2). Since we could not obtain samples between 8 March and 14 September 2022, the anomaly could have possibly started even earlier (any time between March and August 2022), so the positive anomaly (e.g., increase) in the major ions started at least 6months before the 6 February 2023 earthquakes. The Mg2+ content also increased from about 4 to 10 mg L−1 in the period from September 2022 to 15 February 2023. Similar major increases were also detected in Cl−, and SO contents. Water samples are relatively poor in K+ content. Therefore, the increase, due to the scale of the graph, is not very obvious in Fig. 3b. However, the values given in Table 2 clearly indicate about 4 times an increase in the K+ content compared to the background concentrations (post-seismic samples collected between February 15 and 31 March 2023).
The pre-earthquake anomaly in the AYR water samples is characterized by an increase of up to 400 % for the Ec and also major ions, namely Ca2+, Mg2+, K+, Na+, Cl−, and SO before the 6 February 2023 Mw 7.7 and Mw 7.6 earthquakes (Fig. 3b). It is worth noting that the Ec values and major ion concentrations for the Ayran spring waters show similar trends. Post-earthquake samples show decreasing trends in all major ions. Analysis results of the post-earthquake dated samples show that the spring water has had chemical stability since mid-February–early March 2023, just 2–3 weeks after the earthquakes (Fig. 3b). We have also obtained a chemical analysis report on AYR water submitted with the business license application of the company dated 29 August 2012. The chemical analysis data of the samples collected more than 10 years ago include values only for Na+, Cl−, and SO as 3.86, 3.12, and 8.37 mg L−1, respectively. These values are very close to the analysis result of the AYR water sample dated 8 March 2022 (AYR 1, which is the oldest sample in our data set) and the AYR water samples collected after 15 February (Table 2), confirming that these samples represent background values for the AYR spring water.
Immediately after the earthquake, the values started to decrease suggesting a reversible chemical change (Fig. 3b; Table 2). It is worth mentioning that the broad positive anomaly detected in the AYR water chemistry (Fig. 3b) that lasted for about 6 months before the Mw 7.7 and Mw 7.6 earthquakes shows some transient decreases (around mid-December 2022 and toward the end of January 2023). Following each transient decrease, an increase in ion contents is observed and the broad positive anomaly (starting from September 2022) is sustained until the date of the major earthquakes of 6 February 2023. The observations of sudden decrease and rebound in the major ion contents of the water samples (taking place in mid-December 2022 and end of January 2023) may suggest sudden and short-lived crustal stress release related to smaller earthquakes (e.g., earthquakes 4 and 5). Soon after the major earthquakes (earthquakes 1 and 2), the major ion contents of the water samples show a sharp decline, almost approaching the background values as early as 15 February 2023. One single positive anomaly after the major earthquakes (earthquakes 1 and 2) is detected in the sample dated 16 February 2023. The further increase of the ion contents of this sample seems to suggest a short-term stress buildup prior to earthquake 3 (Mw 6.4) that occurred about 120 km to the south of the Ayran spring water location (Fig. 1b). Considering Dobrovolsky et al.'s (1979) theoretical relation (R= 100.43⋅M), an increase in major ion contents of the Ayran spring water is very likely to take place due to an earthquake of magnitude 6.4 occurring at 120 km distance.
We have shown and discussed the reliable precursory anomalies in the major ions of the bottled AYR spring water prior to the Mw 7.7 and Mw 7.6 earthquakes that occurred in the Kahramanmaraş region on 6 February 2023. However, the process(es) leading to the buildup of geochemical anomalies related to the earthquake cannot be inferred with certainty. However, some inferences based on previous observations can be made. For instance, Sibson (1992) suggested that extensive hydro-fracture dilatancy might develop prior to failure leading to the earthquake. Development of fractures probably enhances water circulation and mixing of different reservoirs leading to pre-earthquake anomalies (Italiano et al., 2004; Federico et al., 2008; İnan et al., 2010, 2012c; Skelton et al., 2014; Ingebritsen and Manga, 2014; Doglioni et al., 2014; Barberio et al., 2017; Skelton et al., 2019; Wang and Manga, 2021; Gori and Barberio, 2022). Although the process(es) responsible for chemical anomalies detected in the Ayran spring waters prior to the 6 February 2023 earthquakes cannot be suggested with any certainty at this stage, two immediate mechanisms emerge: (1) a simple increase in fluid flow in the surrounding of the future epicenter and selective dissolution of some K–Mg–Ca-rich rocks (Federico et al., 2008) or (2) “electro-corrosion” whereby the dissolution of rocks is accelerated by the flow of stress-activated positive hole currents (Balk et al., 2009; Freund, 2011; Paudel et al., 2018). Following the second mechanism, the increased content of major ions in water could be related to the oxidation of water to hydrogen peroxide at the rock–water interface (Balk et al., 2009; Paudel et al., 2018). Freund (2011) suggested that with the positive hole current flowing, the “corrosion” of the rock is accelerated releasing into the water major cations and anions. Further work to be conducted in this area may enable us to suggest the process(es) responsible for the pre-earthquake geochemical anomalies we have discussed in the AYR spring water.
Hydrogeochemical precursors have been detected in commercially bottled water samples of natural springs (Ayran Spring and Bahçepınar Spring) emanating from a location about 100 km away from the epicenter of the Mw 7.7 Kahramanmaraş earthquake of 6 February 2023. The pre-earthquake anomaly is characterized by an increase in Ca2+, Mg2+, K+, Na+, Cl−, and SO content in the bottled water samples of the Ayran Spring. Samples that are dated after the earthquakes (covering about 2 months after the earthquake) show decreasing trends in all major ions. About 3 weeks after the earthquake, the major ion contents of the spring water attained stability. At least 6 months of pre-earthquake anomaly (increase) in the major ions content of the Ayran spring water is prominent. It is worth noting that the Bahçepınar spring water samples did not show any anomalies in electrical conductivity. Therefore, the samples were not analyzed for ion content. Bahçepınar water is collected from shallow boreholes dug into alluvial deposits which, we believe, are decoupled from the basement rocks, and this may be the reason for the lack of any significant change in the water chemistry prior to the earthquakes. Here, we remind the reader that geological knowledge of the investigated area and the sampling site is of paramount importance in sampling discrete samples for geochemical analysis and/or conducting continuous monitoring. The results of this study suggest that spring water chemical anomalies may be monitored as proxy indicators of pre-earthquake crustal deformation. The physical mechanisms of the observed precursors are still impossible to explain with certainty at this stage. In order to be able to suggest the mechanism(s) leading to the reported pre-earthquake geochemical anomalies, more work needs to be conducted; especially multi-disciplinary (seismological, geodetical, geochemical) and continuous earthquake monitoring networks must be established and run for a sufficiently long time.
No data sets were used in this article.
SI and HC conceived the project; HC collected the samples; NY coordinated laboratory analysis, compiled seismic events, and prepared the figures; SI was the primary interpreter of the data. SI and HC were writers of the manuscript with contributions from NY.
The contact author has declared that none of the authors has any competing interests.
Publisher’s note: Copernicus Publications remains neutral with regard to jurisdictional claims made in the text, published maps, institutional affiliations, or any other geographical representation in this paper. While Copernicus Publications makes every effort to include appropriate place names, the final responsibility lies with the authors.
We appreciate all the technical help we have received on ion chromatography analyses from Sevde Korkut at the Istanbul Technical University MEM-TEK laboratory. We thank Asen Sabuncu (Istanbul Technical University) and Emre Pınarcı (Çukurova University) for help in drafting the figures. We also thank Tülay İnan and Yağmur Güneş Develioğlu for help in conducting electrical conductivity measurements of the bottled water samples. We thank Filippos Vallianatos for editorial handling. We also appreciate constructive reviews by Giovanni Martinelli, Vivek Valia, and three anonymous reviewers, which helped us improve the manuscript. This work has been partially supported by Istanbul Technical University Scientific Research Fund (ITU BAPSIS) project no. 44774.
This research has been supported by the Istanbul Technical University, Scientific Research Fund (ITU BAP) (grant no. 44774).
This paper was edited by Filippos Vallianatos and reviewed by Giovanni Martinelli and three anonymous referees.
Allen, C. R.: Active faulting in northern Turkey, Division of Geological Sciences, California Institute of Technology, Contribution No. 1577, 32 pp., https://authors.library.caltech.edu/74136/1/Allen_1969p1.pdf (last access: 15 July 2023), 1969.
Ambraseys, N. N.: Temporary seismic quiescence: SE Turkey, Geophys. J. Int., 96, 311–331, https://doi.org/10.1111/j.1365-246X.1989.tb04453.x, 1989.
Areshidze, G., Bella, F., Biagi, P. F., Caputo, M., Della Monica, G., Ermini, A., Manjgaladze, P., Melikazdze, G., Sgrigna, V., and Zilpimani, D.: No preseismic evidence from hydrogeochemical parameters on the occasion of April 29, 1991 Georgian Earthquake, Caucasus, Tectonophysics, 213, 353–358, https://doi.org/10.1016/0040-1951(92)90463-G, 1992.
Arpat, E. and Şaroğlu, F.: Dogu Anadolu fayıile ilgili bazıgözlem ve düşünceler (Some observations and thoughts on the East Anatolian fault). Bulletin of the General Directorate of Mineral Research and Exploration of Turkey (MTA) 73, 44–50, 1972.
Balk, M., Bose, M., Ertem, G., Rogoff, D. A., Rothschild, L. J., and Freund, F. T.: Oxidation of water to hydrogen peroxide at the rock-water interface due to stress-activated electric currents in rocks, Earth Planet Sc. Lett., 283, 87–92, https://doi.org/10.1016/j.epsl.2009.03.044, 2009.
Barberio, M. D., Barbieri, M., Billi, A., Doglioni, C., and Petitta, M.: Hydrogeochemical changes before and during the 2016 Amatrice-Norcia seismic sequence (central Italy), Sci. Rep.-UK, 7, 11735, https://doi.org/10.1038/s41598-017-11990-8, 2017.
Barka, A. A. and Kadinsky-Cade, K.: Strike-slip fault geometry in Turkey and its influence on earthquake activity, Tectonics, 7, 663–684, https://doi.org/10.1029/TC007i003p00663, 1988.
Barka, A. A. and Reilinger, R.: Active tectonics of the Eastern Mediterranean region deduced from GPS, neotectonic and seismicity data, Ann. Geophys.-Italy, 40, 587–610, http://hdl.handle.net/2122/1520 (last access: 20 July 2023), 1997.
Bella, F., Biagi, P. F., Caputo, M., Cozzi, Della Monica, G., Ermini, A., Gordeez, E. I., Khatkevich, Y. M., Martinelli, G., Plastino, W., Scandone, R., Sgrigna, V., and Zilpimiani, D.: Hydrogeochemical anomalies in Kamchatka (Russia), Phys. Chem. Earth., 23, 921–925, https://doi.org/10.1016/S0079-1946(98)00120-7, 1998.
Birchard, G. F. and Libby, W. F.: Earthquake associated radon anomalies possible mechanisms, Eos T. AGU, 59, 329, 1978.
Bozkurt, E.: Neotectonics of Turkey – a synthesis, Geodin. Acta, 14, 3–30, https://doi.org/10.1080/09853111.2001.11432432, 2001.
Cetin, H., Guneyli, H., and Mayer, L.: Paleoseismology of the Palu–Lake Hazar segment of the East Anatolian Fault Zone, Turkey, Tectonophysics 374, 163–197, https://doi.org/10.1016/j.tecto.2003.08.003, 2003.
Chorowicz, J., Luxey, P., Lyberis, N., Carvalho, J., Parrot, J. F., Yurur, T., and Gundogdu, N.: The Maras Triple Junction (southern Turkey) based on digital elevation model and satellite imagery interpretation, J. Geophys. Res., 99, 20225–20242, https://doi.org/10.1029/94JB00321, 1994.
Cicerone, R. D., Ebel, J. E., and Britton, J.: A systematic compilation of earthquake precursors, Tectonophysics, 476, 371–396, https://doi.org/10.1016/j.tecto.2009.06.008, 2009.
Claesson, L., Skelton, A., Graham, C., Dietl, C., Mörth, M., Torssander, P., and Kockum, I.: Hydrogeochemical changes before and after a major earthquake, Geology, 32, 641–644, https://doi.org/10.1130/G20542.1, 2004.
Conti, L., Picozza, P., and Sotgiu, A.: A critical review of ground-based observations of earthquake precursors, Front. Earth Sci., 9, 1–30, https://doi.org/10.3389/feart.2021.676766, 2021.
Dewey, J. F. and Şengör, A. M. C.: Aegean and surrounding regions: complex multi-plate and continuum tectonics in a convergent zone, Geol. Soc. Am. Bull., 90, 84–92, https://doi.org/10.1130/0016-7606(1979)90<84:AASRCM>2.0.CO;2, 1979.
Dewey, J. F., Pitman, W. C., Ryan, W. B. F., and Bonnin, J.: Plate tectonics and the evolution of the Alpine system, Geol. Soc. Am. Bull., 84, 3137–3180, https://doi.org/10.1130/0016-7606(1973)84<3137:PTATEO>2.0.CO;2, 1973.
Di Luccio, F., Chiodini, G., Caliro, S., Cardellini, C., Convertito, V., Pino, N.A., Tolomei, C., and Ventura, G.: Seismic signature of active intrusions in mountain chains, Science Advances, 4, e1701825, https://doi.org/10.1126/sciadv.1701825, 2018.
Dobrovolsky, I. P., Zubkov, S. I., and Miachkin, V. I.: Estimation of the size of earthquake preparation zones, Pure Appl. Geophys., 117, 1025–1044, https://doi.org/10.1007/BF00876083, 1979.
Doglioni, C., Barba, S., Carminati, E., and Riguzzi, F.: Fault on–off versus coseismic fluids reaction, Geosci. Front., 5, 767–780, https://doi.org/10.1016/j.gsf.2013.08.004, 2014.
Etiope, G., Calcara, M., and Quattrocchi, F.: Seismogeochemical algorithms for earthquake prediction: a review, Ann. Geophys.-Italy, 40, 1483–1492, http://hdl.handle.net/2122/1524 (last access: 20 July 2023), 1997.
Federico C., Pizzino, L., Cinti, D., De Gregorio, S., Favara, R., Galli, G., Giudice, G., Guerrieri, S., Quattrocchi, F., and Voltattorni, N.: Inverse and forward modeling of groundwater circulation in a seismically active area (Monferrato, Piedmont, NW Italy): insights into stress-induced variations in water chemistry, Chem. Geol., 248, 14–39, https://doi.org/10.1016/j.chemgeo.2007.10.007, 2008.
Freund, F. T.: Earthquake probabilities and pre-earthquake signals, Curr. Sci., 94, 311-313, https://www.jstor.org/stable/24100338 (last access: 20 July 2023), 2008.
Freund, F. T.: Pre-earthquake signals: Underlying physical processes, J. Asian Earth Sci., 41, 383–400, https://doi.org/10.1016/j.jseaes.2010.03.009, 2011.
Freund, F. T., Takeuchi, A., and Lau, B. W. S.: Electric currents streaming out of stressed igneous rocks – A step towards understanding pre-earthquake low frequency EM emissions, Phys. Chem. Earth, 31, 389–396, https://doi.org/10.1016/j.pce.2006.02.027, 2006.
Gori, F. and Barberio, M. D.: Hydrogeochemical changes before and during the 2019 Benevento seismic swarm in central-southern Italy, J. Hydrol., 604, https://doi.org/10.1016/j.jhydrol.2021.127250, 2022.
Grant, R. A., Halliday, T., Balderer, W. P., Leuenberger, F., Newcomer, M., Cyr, G., and Freund, F. T.: Groundwater chemistry changes before major earthquakes and possible effects on animals, Int. J. Environ. Res. PU, 1936–1956, https://doi.org/10.3390/ijerph8061936, 2011.
Geller, R. J., Jackson, D. D., Kagan, Y. Y., and Mulargia, F.: Earthquake cannot be predicted, Science, 275, 1616–1617, https://doi.org/10.1126/science.275.5306.1616, 1997.
Hancock, P. L. and Barka, A. A.: Kinematic indicators on active normal faults in western Turkey, J. Struct Geol., 9, 415–430, https://doi.org/10.1016/0191-8141(87)90142-8, 1987.
Hartmann, J. and Levy, J. K.: The influence of seismotectonic on precursory changes in groundwater composition for the 1995 Kobe earthquake, Japan, Hydrol. J., 14, 1307–1318, https://doi.org/10.1007/s10040-006-0030-7, 2006.
Hempton, M. R.: The North Anatolian fault and complexities of continental escape, J. Struct Geol., 4, 502–504, https://doi.org/10.1016/0191-8141(82)90041-4, 1982.
Hauksson, E.: Radon content of groundwater as an earthquake precursor: Evaluation of worldwide data and physical basis, J. Geophys. Res., 86, 9397–9410, https://doi.org/10.1029/JB086iB10p09397, 1981.
He, A. and Singh, R. P.: Groundwater level response to the Wenchuan earthquake of May 2008, Geomat. Nat. Haz. Risk, 10, 336-352, https://doi.org/10.1080/19475705.2018.1523236, 2019.
İnan, S., Ergintav, S., Saatçılar, R., Tüzel, B., and Iravul, Y.: Turkey makes major investments in earthquake research, EOS Transactions, 88, 333–334, https://doi.org/10.1029/2007EO340002, 2007.
İnan, S., Akgül, T., Seyis, C., Saatçılar, R., Baykut, S., Ergintav, S., and Bas, M.: Geochemical monitoring in the Marmara Region (NW Turkey): A search for precursors of seismic activity, J. Geophys, Res., 113, B03401, https://doi.org/10.1029/2007JB005206, 2008.
İnan, S., Ertekin, K., Seyis, C., Şimşek, Ş., Kulak, F., Dikbaş, A., Tan, O., Ergintav, S., Çakmak, R., Yörük, A., Çergel, M., Yakan, H., Karakuş, H., Saatçılar, R., Akçiğ, Z., Iravul, Y., and Tüzel, B.: Multi-disciplinary earthquake researches in Western Turkey: Hints to select sites to study geochemical transients associated to seismicity, Acta Geophys., 58, 767–813, https://doi.org/10.2478/s11600-010-0016-7, 2010.
İnan, S., Pabuçcu, Z., Kulak, F., Ergintav, S., Tatar, O., Altunel, E., Akyüz, S., Tan, O., Seyis, C., Çakmak, R., Saatçılar, R., and Eyidogan, H.: Microplate boundaries hindering ¢ pre-earthquake strain transfer (Western Turkey): inferences from continuous geochemical monitoring, J. Asian Earth Sci., 48, 56–71, https://doi.org/10.1016/j.jseaes.2011.12.016, 2012a.
İnan, S., Kop, A., Çetin, H., Kulak, F., Pabuçcu, Z., Seyis, C., Ergintav, S., Tan, O., Saatçılar, S., and Bodur, M. N.: Seasonal variations in soil radon emanation: Long term continuous monitoring in light of seismicity, Nat. Hazards, 62, 575–591, https://doi.org/10.1007/s11069-012-0096-6, 2012b.
İnan, S., Balderer, W. P., Leuenberger-West, F., Yakan, H., Özvan, A., and Freund, F. T.: Springwater chemical anomalies prior to the Mw = 7.2 Van Earthquake (Turkey), Geochem. J., 46, e11–e16, https://doi.org/10.2343/geochemj.1.0159, 2012c.
Ingebritsen, S. E. and Manga, M.: Earthquakes: hydrogeochemical precursors, Nat. Geosci., 7, 697–698, https://doi.org/10.1038/ngeo2261, 2014.
Italiano, F., Martinelli, G., and Rizzo, A.: Geochemical evidence of seismogenic-induced anomalies in the dissolved gases of thermal waters: A case study of Umbria (Central Apennines, Italy) both during and after the 1997–1998 seismic swarm, Geochem. Geophy. Geosy., 5, Q11001, https://doi.org/10.1029/2004GC000720, 2004.
Jackson, J. and McKenzie, D. P.: Active tectonics of the Alpine–Himalayan Belt between western Turkey and Pakistan, Geophys. J. Roy. Astr. S., 77, 185–264, https://doi.org/10.1111/j.1365-246X.1984.tb01931.x, 1984.
Karig, D. E. and Kozlu, H.: Late Palaeogene – Neogene evolution of the triple junction region near Maras, south-central Turkey, J. Geol. Soc. London, 147, 1023–1034, https://doi.org/10.1144/gsjgs.147.6.1023, 1990.
Kelling, G., Gökçen, S. L., Floyd, P. A., and Gökçen, N.: Neogene tectonics and plate convergence in the Eastern Mediterranean: new data from southern Turkey, Geology, 15, 425–429, https://doi.org/10.1130/0091-7613(1987)15<425:NTAPCI>2.0.CO;2, 1987.
Kempler, D. and Garfunkel, Z.: Northeast Mediterranean triple junction from a plate kinematics point of view, Bulletin of the Technical University of Istanbul, 44, 425–454, 1991.
Ketin, I.: Über die tektonisch-mechanischen Folgerungen aus den grossen anatolischen Erdbeben des letzten Dezenniums, Geol. Rundsch., 36, 77–83, https://doi.org/10.1007/BF01791916, 1948.
King, J. Y., Koizumi, Y., and Kitagawa, Y.: Hydrogeochemical anomalies and the 1995 Kobe Earthquake, Science, 269, 38–39, https://doi.org/10.1126/science.269.5220.38, 1995.
Lovelock, P. E. R.: A review of the tectonics of the northern Middle East region, Geol. Mag., 121, 577–587, 1984.
Martinelli, G. and Ferrari, G.: Earthquake forerunners in a selected area of Northern Italy: recent developments in automatic geochemical monitoring, Tectonophysics, 193, 397–410, https://doi.org/10.1016/0040-1951(91)90348-V, 1991.
McKenzie, D. P.: Active tectonics of the Mediterranean Region, Geophys. J. Int., 30, 109–185, https://doi.org/10.1111/j.1365-246X.1972.tb02351.x, 1972.
McKenzie, D. P.: The East Anatolian fault: a major structure in eastern Turkey, Earth Pl. Sc. Lett., 29, 189–193, https://doi.org/10.1016/0012-821X(76)90038-8, 1976.
Muehlberger, W. B. and Gordon, M. B.: Observations on the complexity of the East Anatolian fault, Turkey, J. Struct Geol., 9, 899–903, https://doi.org/10.1016/0191-8141(87)90091-5, 1987.
Nur, A.: Matsushiro, Japan, earthquake swarm: Confirmation of the dilatancy-fluid diffusion model, Geology, 2, 217–221, https://doi.org/10.1130/0091-7613(1974)2<217:MJESCO>2.0.CO;2, 1974.
Ouzounov, D., Pulinets, S., Davidenko, D., Rozhnoi, A., Solovieva, M., Fedun, V., Dwivedi, B. N., Rybin, A., Kafatos, M., and Taylor, P.: Transient effects in atmosphere and ionosphere preceding the 2015 M7.8 and M7.3 Gorkha-Nepal earthquakes, Front. Earth Sci., 9, https://doi.org/10.3389/feart.2021.757358, 2021.
Papadopoulos, G. A., Latoussakis, I., Daskalaki, E., Diakogianni, G., Fokaefs, A., Kolligri, M., Liadopoulou, K., Orfanogiannaki, K., and Pirentis, A.: The East Aegean Sea strong earthquake sequence of October–November 2005: lessons learned for earthquake prediction from foreshocks, Nat. Hazards Earth Syst. Sci., 6, 895–901, https://doi.org/10.5194/nhess-6-895-2006, 2006.
Paudel, S. R., Banjara, S. P., Wagle, A., and Freund, F. T.: Earthquake chemical precursors in groundwater: a review, J. Seismol., 22, 1293–1314, https://doi.org/10.1007/s10950-018-9739-8, 2018.
Pérez, N. M., Hernández, P. A., Igarashi, G., Trujillo, I., Nakai, S., Sumino, H., and Wakita, H.: Searching and detecting earthquake geochemical precursors in CO2-rich groundwaters from Galicia, Spain, Geochem J., 42, 75–83, https://doi.org/10.2343/geochemj.42.75, 2008.
Perinçek, D. and Çemen, I.: The structural relationship between the East Anatolian and Dead Sea fault zones in southeastern Turkey, Tectonophysics, 172, 331–340, https://doi.org/10.1016/0040-1951(90)90039-B, 1990.
Planinić, J., Vuković, B., and Radolić, V.: Radon time variations and deterministic chaos, J. Environ. Radioactiv., 75, 35–45, 2004.
Rapti, D., Martinelli, G., Zheng, G., and Vincenzi, C.: Bottled Mineral Waters as Unconventional Sampling in Hydro-Geological Research, Water, 15, 3466, https://doi.org/10.3390/w15193466, 2023.
Rikitake, T.: Classification of earthquake precursors, Tectonophysics, 54, 293–309, https://doi.org/10.1016/0040-1951(79)90372-X, 1979.
Rikitake, T.: Earthquake Precursors in Japan: Precursor Time and Detectability, Tectonophysics, 136, 265–282, https://doi.org/10.1016/0040-1951(87)90029-1, 1987.
Roeloffs, E.: Poroelastic techniques in the study of earthquake-related hydrologic phenomena, Adv. Geophys., 37, 135–195, https://doi.org/10.1016/S0065-2687(08)60270-8, 1996.
Rotstein, Y.: Counterclockwise rotation of the Anatolian block, Tectonophysics, 108, 71–91, https://doi.org/10.1016/0040-1951(84)90155-0, 1984.
Şaroğlu, F., Emre, O., and Kuscu, I.: Active Fault map of Turkey (1:2 000 000 scale), General Directorate of Mineral Research and Exploration (MTA), Ankara, Turkey, 1992a.
Şaroğlu, F., Emre, O., and Kuscu, I.: The East Anatolian fault zone of Turkey, Annales Tectonicae: International Journal of Structural Geology and Tectonics, 6, 99–125, 1992b.
Scholz, R., Sykes, L. R., and Aggarwal, Y. P.: Earthquake prediction: A physical basis, Science, 181, 803–810, https://doi.org/10.1126/science.181.4102.803, 1973.
Sengör, A. M. C. and Yılmaz, Y.: Tethyan evolution of Turkey: A plate tectonic approach, Tectonophysics, 75, 181–241, https://doi.org/10.1016/0040-1951(81)90275-4, 1981.
Şengör, A. M. C., Görür, N., and Şaroğlu, F.: Strike-slip faulting and related basin formation in zones of tectonic escape: Turkey as a case study, in: Strike-slip Deformation, Basin Formation, and Sedimentation, edited by: Biddle, K. T. and Christie-Blick, N., Soc. Econ. Pa, 37, 227–264, 1985.
Seyis, C., İnan, S., and Yalçın, M. N.: Major factors affecting soil radon emanation, Nat. Hazards, 114, 2139–2162, https://doi.org/10.1007/s11069-022-05464-y, 2022.
Sibson, R. H.: Implications of fault-valve behavior for rupture nucleation and recurrence, Tectonophysics, 211, 283–293, https://doi.org/10.1016/0040-1951(92)90065-E, 1992.
Sibson, R. H., Moore, J. M. M., and Rankin, A. H.: Seismic pumping – a hydrothermal fluid transport mechanism, J. Geol. Soc. London, 131, 653–659, https://doi.org/10.1144/gsjgs.131.6.0653, 1975.
Skelton, A., Andrén, M., Kristmannsdóttir, H., Stockmann, G., Mörth, C.-M., Sveinbjörnsdóttir, A., Jónsson, S., Sturkell, E., Guðrúnardóttir, H. R., Hjartarson, H., Siegmund, H., and Kockum, I.: Changes in groundwater chemistry before two consecutive earthquakes in Iceland, Nat. Geosci., 7, 752–756, https://doi.org/10.1038/NGEO2250, 2014.
Skelton, A., Liljedahl-Claesson, L., Wästeby, N., Andrén, M., Stockmann, G., Sturkell, E., Mörth, C., Stefansson, A., Tollefsen, E., Seigmund, H., Keller, N., Kjartansdottir, R., Hjartarson, H., and Kockum, I.: Hydrochemical changes before and after earthquakes based on long-term measurements of multiple parameters at two sites in northern Iceland – a review, J. Geophys. Res-Sol. Ea., 124, 2702–2720, https://doi.org/10.1029/2018JB016757, 2019.
Sol, S., Meltzer, A., Bürgmann, R., van der Hilst, R. D., King, R., Chen, Z., Koons, P. O., Lev, E., Liu, Y. P., Zeitler, P. K., Zhang, X., Zhang, J., and Zurek, B.: Geodynamics of the southern Tibetan Plateau from seismic anisotropy and geodesy, Geology, 35, 563–566, https://doi.org/10.1130/G23408A.1, 2007.
Soysal, H., Sipahioğlu, S., Kolçak, D., and Altınok, Y.: Historical earthquake catalogue of Turkey and surrounding area (2100 B.C.–1900 A.D.), The Scientific and Technological Research Council of Turkey Technical Report, TBAG-341, 1981.
Sugisaki, R., Ito, T., Nagamine, K., and Kawabe, I.: Gas geochemical changes at mineral springs associated with the 1995 southern Hyogo earthquake (M= 7.2), Japan, Earth Planet Sc. Lett., 139, 239–249, https://doi.org/10.1016/0012-821X(96)00007-6, 1996.
Sultankhodhaev, G. A.: International symposium on earthquake prediction, UNESCO, Paris 1979, 181–191, https://unesdoc.unesco.org/ark:/48223/pf0000063090 (last access: 22 July 2023), 1984.
Tansi, C., Tallarico, A., Iovine, G., Folino Gallo, M., and Falcone, G.: Interpretation of radon anomalies in seismotectonic and tectonic-gravitational settings: the southeastern Crati graben (Northern Calabria, Italy), Tectonophysics, 396, 181–193, https://doi.org/10.1016/j.tecto.2004.11.008, 2005.
Thomas, D. M., Cuff, K. E., and Cox, M. E.: The association between ground gas radon variations and geologic activity in Hawaii, J. Geophys. Res.-Sol. Ea., 91, 12186–12198, https://doi.org/10.1029/JB091iB12p12186, 1986.
Toutain, J. P., Munoz, M., Poitrasson, F., and Lienard, F.: Springwater chloride ion anomaly prior to a ML = 5.2 Pyrenean earthquake, Earth Planet Sc. Lett., 149, 113–119, https://doi.org/10.1016/S0012-821X(97)00066-6, 1997.
Tsunogai, U. and Wakita, H.: Precursory chemical changes in groundwater: Kobe earthquake, Japan, Science, 269, 61–63, https://doi.org/10.1126/science.269.5220.61, 1995.
Turcotte, W. L.: Earthquake prediction, Annu. Rev. Earth Pl. Sc., 19, 263–281, https://doi.org/10.1146/annurev.ea.19.050191.001403, 1991.
Usta, D., Ateş, Ş., Beyazpirinç, M., Kanar, F., Yıldız, H., Uçar, L., Akça, İ., Tufan, E., and Örtlek, A. T.: New data on 90 the stratigraphy of the central and northern Amonous mountains (Osmaniye – Gaziantep – Kahramanmaraş), Bulletin of the Turkish Association of Petroleum Geologists, 27, 57–98, 2015.
Usta, D., Ateş, Ş., Kanar, F., Beyazpirinç, M., Uçar, L., Yıldız, H., Tufan, E., Akça, İ., and Örtlek, A. T.: Doğu Toroslar'ın jeolojisi ve jeodinamik evrimi projesi (K.Maras, Osmaniye, Gaziantep, Adana, Hatay), General Directorate of Minerals and Exploration of Turkey (MTA) unpublished Report No. 13568, 494 pp., https://eticaret.mta.gov.tr/index.php?route=product/product&product_id=18289 (last access: 10 July 2023), Ankara, 2017 (in Turkish).
Uyeda, S., Nagao, T., and Kamogawa, M.: Short-term earthquake prediction: Current status of seismo-electromagnetics, Tectonophysics, 470, 205–213, https://doi.org/10.1016/j.tecto.2008.07.019, 2008.
Wakita, H.: Geochemical challenge to earthquake prediction, P. Natl. Acad. Sci. USA, 93, 9, 3781–3786, https://doi.org/10.1073/pnas.93.9.3781, 1996.
Wakita, H., Nakamura, Y., and Sano, Y.: Short-term and intermediate-term geochemical precursors, Pure Appl. Geophys., 125, 267–278, https://doi.org/10.1007/BF00878999, 1988.
Walia, V., Yang, T. F., Hong, W. L., Lin, S. J., Fu, C. C., Wen, K. L., and Chen, C. H.: Geochemical variation of soil–gas composition for fault trace and earthquake precursory studies along the Hsincheng fault in NW Taiwan, Appl. Radiat. Isotopes, 67, 1855–1863, https://doi.org/10.1016/j.apradiso.2009.07.004, 2009.
Wang, C.-Y. and Manga, M.: Water and Earthquakes. Lecture Notes in Earth System Sciences, Springer, 307 pp., https://doi.org/10.1007/978-3-030-64308-9, 2021.
Westaway, R. and Arger, J.: The Gölbaşıbasin, southeastern Turkey: a complex discontinuity in a major strike-slip fault zone, J. Geol. Soc. London, 153, 729–744, https://doi.org/10.1144/gsjgs.153.5.0729, 1996.
Xiang, Y. and Peng, S.: Hydrochemical and stable isotopes del2H and del18O) changes of groundwater from a spring induced by local earthquakes, Northwest China, Front. Earth Sci., 10, https://doi.org/10.3389/feart.2022.1100068, 2023.
Vallianatos, F. and Tzanis, A.: Electric current generation associated with the deformation rate of a solid: Preseismic and coseismic signals, Phys. Chem. Earth Parts A/B/C, 23, 933–938, https://doi.org/10.1016/S0079-1946(98)00122-0, 1998.
Vallianatos, F., Triantis, D., Tzanis, A., Anastasiadis, C., and Stavrakas, I.: Electric earthquake precursors: from laboratory results to field observations, Phys. Chem. Earth Parts A/B/C 29, 339–351, https://doi.org/10.1016/j.pce.2003.12.003, 2004.
Virk, H. S. and Singh, B.: Radon anomalies in soil gas and groundwater as earthquake precursor phenomena, Tectonophysics, 227, 215–224, https://doi.org/10.1016/0040-1951(93)90096-3, 1993.
Yönlü, Ö., Altunel, E., and Karabacak, V.: Geological and geomorphological evidence for the southwestern extension of the East Anatolian Fault Zone, Turkey, Earth Planet Sc. Lett., 469, 1–14, https://doi.org/10.1016/j.epsl.2017.03.034, 2017.
Yu, H., Liu, L., Ma, Y., Yan, R., Liu, J., Ma, Y., Li, Z., Zhang, X., Zhao, J., and Yu, C.: Observed hydrological changes associated with active tectonic blocks before three consecutive earthquakes in Qinghai, China, Sci. Rep., 13, 8988, https://doi.org/10.1038/s41598-023-36274-2, 2023.
Zeyrek, M., Ertekin, K., Kacmaz, S., Seyis, C., and İnan, S.: An ion chromatography method for the determination of major anions in geothermal water samples, Geostand. Geoanal. Res., 34, 67–77, https://doi.org/10.1111/j.1751-908X.2009.00020.x, 2010.