the Creative Commons Attribution 4.0 License.
the Creative Commons Attribution 4.0 License.
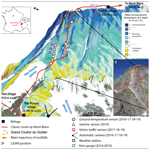
Multi-method monitoring of rockfall activity along the classic route up Mont Blanc (4809 m a.s.l.) to encourage adaptation by mountaineers
Jacques Mourey
Pascal Lacroix
Pierre-Allain Duvillard
Guilhem Marsy
Marco Marcer
Emmanuel Malet
Ludovic Ravanel
There are on average 35 fatal mountaineering accidents per summer in France. On average, since 1990, 3.7 of them have occurred every summer in the Grand Couloir du Goûter, on the classic route up Mont Blanc (4809 m a.s.l.). Rockfall is one of the main factors that explain this high accident rate and contribute to making it one of the most accident-prone areas in the Alps for mountaineers. In this particular context, the objective of this study is to document the rockfall activity and its triggering factors in the Grand Couloir du Goûter in order to disseminate the results to mountaineers and favour their adaptation to the local rockfall hazard. Using a multi-method monitoring system (five seismic sensors, an automatic digital camera, three rock subsurface temperature sensors, a traffic sensor, a high-resolution topographical survey, two weather stations and a rain gauge), we acquired a continuous database on rockfalls during a period of 68 d in 2019 and some of their potential triggering factors (precipitation, ground and air temperatures, snow cover, frequentation by climbers). At the seasonal scale, our results confirm previous studies showing that rockfalls are most frequent during the snowmelt period in permafrost-affected rockwalls. Furthermore, the unprecedented time precision and completeness of our rockfall database at high elevation thanks to seismic sensors allowed us to investigate the factors triggering rockfalls. We found a clear correlation between rockfall frequency and air temperature, with a 2 h delay between peak air temperature and peak rockfall activity. A small number of rockfalls seem to be triggered by mountaineers. Our data set shows that climbers are not aware of the variations in rockfall frequency and/or cannot/will not adapt their behaviour to this hazard. These results should help to define an adaptation strategy for climbers. Therefore, we disseminated our results within the mountaineering community thanks to the full integration of our results into the management of the route by local actors. Knowledge built during this experiment has already been used for the definition and implementation of management measures for the attendance in summer 2020.
- Article
(7423 KB) - Full-text XML
- BibTeX
- EndNote
Despite a growing body of scientific literature, several international entities such as the World Meteorological Organization (WMO), the Intergovernmental Panel on Climate Change (IPCC) and the Mountain Research Initiative (MRI) agree that there is a profound lack of knowledge of the vulnerability of socio-economic activities to climate change in mountain areas. Echoing this observation, a review of studies by McDowell et al. (2019) on adaptation actions in glaciated mountain areas showed that the majority of the adaptation strategies are implemented in direct reaction to stimuli and do not respond to well-thought-out plans that are based on scientific knowledge and take into account all the interacting socio-economic factors as well as the future effects of climate change. According to the authors, this results in a significant lack of medium- and long-term efficiency of adaptation strategies.
Mountaineering is an emblematic activity that was recently inscribed by UNESCO onto the Representative List of the Intangible Cultural Heritage of Humanity (Debarbieux, 2020) and is strongly affected by climate change (Ritter et al., 2011; Temme, 2015; Pröbstl-Haider et al., 2016; Purdie and Kerr, 2018; Mourey et al., 2019). Mountaineers are now forced to adapt, in particular to growing hazards. The climbing parameters – technical difficulty, level of exposure to natural hazards and optimal periods for climbing – of the classic mountaineering route up Mont Blanc (4809 m a.s.l., Mont Blanc massif – MBM – France), undoubtedly one of the most popular in the world (200 000 passages per year; Mourey and Ravanel, 2017), have significantly changed because of the effects of climate change (Mourey et al., 2019). This evolution is mostly related to an increasing number of rockfalls on the west face of the Aiguille du Goûter (3863 m a.s.l.), which includes the crossing of the Grand Couloir du Goûter and the ascent of the Goûter rock ridge (“arête du Goûter”) leading to the Goûter refuge located at 3835 m a.s.l. (Fig. 1). According to Magnin et al. (2015), this sector is located in a context of permafrost – any lithospheric material with a temperature remaining below or at 0 ∘C for at least 2 years (NRCC, 1988; Van Everdingen, 1998; Fig. 1).
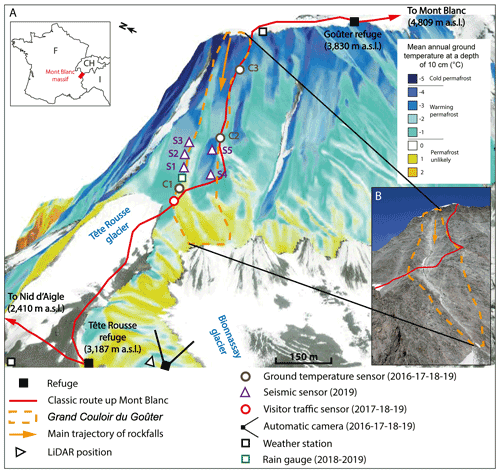
Figure 1Study site and multi-method monitoring system at the Grand Couloir du Goûter. (a) Permafrost map from the modelling of Magnin et al. (2015) (aerial photography © Microsoft) showing the locations of the classic route up Mont Blanc on the west face of the Aiguille du Goûter (in red) and the instruments set up in the area. The years in which the sensors were present are indicated. (b) Picture of the couloir taken by the automatic camera (4 August 2019).
There are on average 35 fatal mountaineering accidents per summer in France (Soulé et al., 2014). On average, since 1997, 3.7 of those fatal accidents have occurred in the Grand Couloir du Goûter (Mourey et al., 2018), hence its reputation in the media as the “couloir of death”. Rockfalls directly explain 29 % of the accidents and are partly involved in 50 % of the accidents due to a fall of a climber in the couloir (Mourey et al., 2018). Rockfalls are therefore one of the main factors that explain this high accident rate and contribute to making it one of the most accident-prone areas in the Alps for mountaineers.
Despite the number of mountaineers and the high accident rate in the Goûter area, before the summer of 2020, no management measures were initiated by the actors in charge of the route, and only a few scientific studies were carried out about the occurrence of rockfalls and their triggering factors. Based on 42 d of in situ visual observations during the day (08:00–06:00 LT) in the summer of 2011, Alpes Ingé (2012) showed that a rockfall occurred on average every 28 min in summertime. However, that study had many limitations: rockfalls were not measured over the whole season, nor during periods of bad weather (rain, fog, thunderstorms), nor at the end of the day, night and early morning. Lemarechal (2011) proposed, on the basis of a trajectography study, various possibilities for securing the crossing of the couloir, such as purging the face, installing block nets, protecting the crossing with a concrete structure or installing a footbridge. However, until now, no continuous observation of rockfalls has been available to better characterise their occurrence and their triggering factors (temperature, rainfall, snow cover, human activity).
Our study was motivated by the high number of accidents in the Goûter area and the fact that rockfalls are increasingly frequent there (Mourey et al., 2019). Therefore, we aimed at better characterising the rockfall hazard and its origins in the Goûter area through an integrated approach of data acquisition and dissemination within the mountain community to promote the implementation of efficient adaptive behaviours and to avoid an increase in the number of accidents due to rockfalls, or even to reduce that number. To meet our objective, we quantitatively documented the climber traffic, the occurrence of rockfalls and their potential triggering factors in the Grand Couloir du Goûter. Comparing rockfall occurrences and environmental parameters enabled us to identify the most favourable conditions for rockfall, while an analysis of the traffic showed whether it had adapted to the local rockfall hazard or a change in traffic was required.
In the context of permafrost in high Alpine environments, rockfall occurrence is preconditioned by the structural and lithological characteristics of the rock (especially its degree of fracturing) and the topography of the rockwalls (Krautblatter et al., 2013; McColl and Draebing, 2019). Meteorological factors and ground characteristics such as the presence/absence of snow, precipitation, and air and ground temperatures will initiate thermomechanical processes involved in rockfall triggering. In a steep permafrost slope such as the west face of the Aiguille du Goûter, these processes can be operating and, depending on the conditions, can favour the stability or, on the contrary, the instability of the slope. Draebing et al. (2014) proposed a conceptual model to understand these interactions and their role. On a seasonal scale, two periods particularly conducive to rockfalls are identified: early summer and autumn. In early summer, the infiltration of liquid water from melting snow accelerates the deepening of the active layer – the subsurface horizon that thaws during the summer period – along the cracks (Gruber and Haeberli, 2007) and causes the melting of the ice in the cracks (Hasler et al., 2011). As a result, the shear strength of the rock is reduced by the thawing of the active layer while the hydrostatic pressure increases, favouring the initiation of rockfalls (McColl and Draebing, 2019). In autumn, the cooling of the rock leads to the freezing of water and an increase in cryostatic pressure. Ravanel et al. (2017) also identify autumn as a favourable period for the occurrence of large-scale destabilisations (V>10 000 m3), as deep warming of the terrain reaches its maximum at this time of the year. Thus, the triggering processes of rockfalls in high alpine environments are relatively well identified on a seasonal scale. However, they are much less quantified on a daily and hourly scale due to the lack of data on rockfall activity at high altitudes at these fine temporal scales. Presently, only seismic measurements can be used to obtain a continuous record of the rockfall activity during days and nights, independently of the weather conditions (Helmstetter and Garambois, 2010; Hibert et al., 2011, 2017; DeRoin and Mc Nutt, 2012; Dietze et al., 2017a, b; Durand et al., 2018). All those studies, however, focused on un- or deglaciated mountain areas. Some authors have used seismic monitoring for the detection of large rockfalls at regional scales (Dussauge et al., 2003; Dammeier et al., 2011; Manconi et al., 2016; Hibert et al., 2019), and have thus not focused on permafrost conditions. To our knowledge, only Guillemot et al. (2020) have provided a rockfall database for a rock glacier area in Switzerland. They showed a direct correlation at the daily scale between rockfall activity and air temperature, caused by increased meltwater production.
In order to quantify the frequency of rockfalls and their triggering factors in the local context of the Grand Couloir du Goûter, a multi-method monitoring system was set up in 2016 and became fully operational in 2019. As shown in Fig. 1, it is composed of (i) five seismic sensors to detect the impacts of rockfalls during the day and night, independently of weather conditions, and to estimate their intensities, (ii) an automatic digital camera to monitor the evolution of the snow-covered surfaces in the couloir, (iii) three subsurface temperature sensors installed 10 cm deep in the rock to analyse the presence/absence of the permafrost and its thermal regime; (iv) a pyroelectric sensor to record the number, time and direction of climbers crossing the couloir), (v) a high-resolution topographical survey performed by terrestrial laser scanning to define the topography of the couloir, (vi) two weather stations measuring the air temperature (one near the Tête Rousse glacier (3126 m a.s.l.) and the other close to the Goûter refuge (3817 m a.s.l.)) (Vincent et al., 2015) and (vii) a rain gauge positioned at the base of the couloir (3270 m a.s.l.) to measure rainfall. In this paper, we present this experiment and the novel results obtained on the rockfall activity at the hourly and daily scale during the 2019 season, together with an analysis of the mountaineers' behaviour regarding the rockfall hazard.
The MBM is located in the north-western Alps, between Switzerland, Italy and France (Fig. 1), and covers ∼550 km2. About 30 % of its surface is covered with ice (Gardent et al., 2014) comprising 121 glaciers (Paul et al., 2020), including the Mer de Glace, the largest glacier in the French Alps, with an area of 30 km2. Twenty-eight summits exceed 4000 m a.s.l., including Mont Blanc (4809 m a.s.l.), the highest summit of the European Alps. The Grand Couloir du Goûter (Fig. 1) is located on the west face of the Aiguille du Goûter (3863 m a.s.l.), located in the south-western part of the MBM. The classic route up Mont Blanc crosses this couloir in its lower part, at an altitude of 3270 m a.s.l., over a horizontal distance of about 70 m. The route then follows the left side of the couloir over an elevation gain of almost 500 m up to its summit at 3817 m a.s.l. This sector – the traverse of the couloir and the ascent of its left ridge – is one of the most difficult parts of the route because of its steepness (slope angle between 45 and 60∘), and is also the most dangerous because of rockfalls, which have increased in frequency and volume in recent decades (Mourey et al., 2019).
The ascent of Mont Blanc is classically done over 2 d (one night in a refuge), with two main possibilities for the choice of refuge and the preferred time to cross the Grand Couloir du Goûter. The first possibility is to spend the night at the Tête Rousse (3187 m a.s.l.) or Nid d'Aigle (2400 m a.s.l.) refuges located below the couloir (Fig. 1). The climbers can then cross the couloir twice on the second day; first very early in the morning on the way up, and again in the afternoon on the way down from the summit. The second possibility is to sleep at the Goûter refuge (3830 m a.s.l.; located on the summit ridge of the Aiguille du Goûter, Fig. 1). Climbers thus cross the couloir on the first day on the way up, generally at the end of the morning/beginning of the afternoon. They cross the couloir again on the way down from the summit, usually in the early afternoon. The timing of the crossing is therefore linked to the refuges and the climbing schedule chosen by the climbers, not to the rockfall hazard.
In 2015, due to two heat waves during which rockfalls were particularly frequent, climbing the couloir was strongly discouraged and the Goûter refuge was closed by prefectural decree from 15 to 31 July and then from 6 to 19 August, corresponding to 23 % of the traditional opening period of the refuge. These closures resulted in a drop in the number of overnight stays of 17 % for the Goûter refuge and 39 % for the Tête Rousse refuge compared to the average for the 3 previous years.
The topographical and geological characteristics of the Grand Couloir du Goûter are particularly favourable to rockfalls. The sector is formed from highly fractured gneiss and micaschists (Mennessier et al., 1977), with a slope angle of between 45 and 60∘ over an altitude difference of 700 m. Due to the fracturing of the rock in the area and previous rockfalls in the couloir, many rocks/blocks are susceptible to a secondary fall. Moreover, the west face of the Aiguille du Goûter is located in the altitudinal range where there is high degradation of the permafrost, with mean annual rock surface temperatures (MARSTs) mostly between −1 and −4 ∘C (Magnin et al., 2015), which is the most favourable temperature range observed for the occurrence of rockfalls in the MBM due to permafrost degradation (Legay et al., 2021).
Several factors potentially responsible for the triggering of rockfalls were studied and quantified, as well as the traffic along the route, through a multi-method monitoring system.
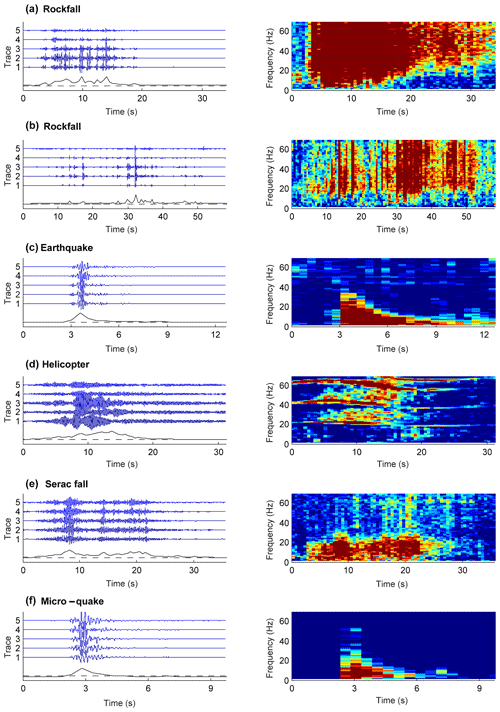
Figure 2Example of typical signals recorded by the seismic network on 28 June 2019: two rockfalls in the Grand Couloir du Goûter (a, b), a local earthquake (c), a helicopter (d), a serac fall from the north face of the Bionnassay (e) and a micro-quake (f). On the left, the seismic waveforms at each sensor (1 to 5) are represented in blue and the seismic envelope is shown in black. The right panels present the spectrograms at low frequencies (0–75 Hz) averaged over all the sensors. Red represents a higher energy than blue.
3.1 Rockfall detection and characterisation
Five autonomous seismic sensors (Zland3C nodes; short period, with a sampling frequency of 250 Hz) were installed on both sides of the couloir (Fig. 1) from 29 June to 4 September 2019 (68 d). This seismic network was used for the detection of the seismic signals generated by rockfalls and to characterise their energy. The detection was carried out following the method developed by Helmstetter and Garambois (2010), which involves isolating spectrogram peaks stacked in the frequency band 1–30 Hz that have amplitudes greater than 4 times the noise level (calculated over 30 s at the onset of the records). All peaks separated by less than 30 s are considered to be part of the same event. This method makes it possible to isolate a large number of signals, not all of which are rockfalls (earthquakes and anthropogenic noise, including helicopters; Fig. 2). A visual expert analysis was then used to classify these signals and identify those due to rockfalls. The visual expertise was trained by field comparison done 2 times during 2 h: once in July and once in August 2019. The rockfall signals in the couloir are characterised by many impacts, high-frequency content, and long signals (Fig. 2a and b). Some rockfall signals can be confused with serac falls in the north face of the Bionnassay, 800 m west of the couloir (Fig. 2e). However, the absence of high-frequency content due to the attenuation of these “far” signals is a decisive criterion for rejecting them. Some rockfalls are also characterised by one or few (<3) impacts, making them complex to identify and separate from local microquakes or anthropogenic noise due to mountaineers in the couloir. Those signals correspond to the smallest rockfalls. Therefore, we decided to keep only the largest signals, i.e. when the rockfall origin was known. This corresponded to about only 20 % of the detected signals. Consequently, the smallest rockfalls or those that did not have a marked impact on the couloir – yet were potentially fatal for climbers – were not necessarily detected in our study, which rejected all the signals from uncertain sources (∼80 % of the total number of signals). The detection of small rockfalls in the seismic records could be improved using machine learning algorithms (e.g. Hibert et al., 2017) trained on sample signals checked in the field.
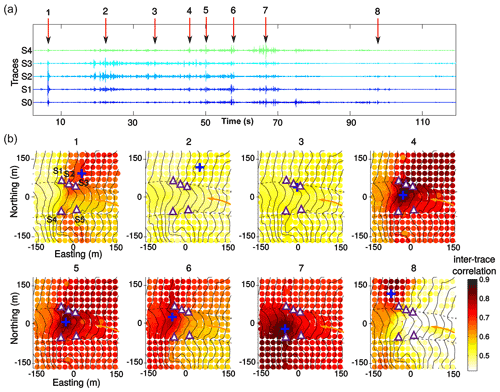
Figure 3(a) Seismic waveforms of multiple successive rockfalls that occurred on 2 August 2019, as recorded by the five sensors (denoted S1–S5 and indicated by triangles on the maps of subplots b). The main impacts (denoted 1–8) are automatically located by the beam-forming method. (b) Spatial distribution of the inter-trace correlation for each of the eight impacts. The optimum location is indicated by a blue cross. The elevation contours are displayed every 25 m. The yellow arrow indicates the downward propagation of the rockfalls in the couloir.
Finally, the most energetic peaks of each signal were located by a beam-forming method (Lacroix and Helmstetter, 2011) that consisted of searching for the source location and mean seismic wave velocity in the media that maximise the inter-trace correlation shifted in time by the respective source/sensor propagation time (Fig. 3). Signals with the majority of the peaks located in the couloir were kept (Fig. 3). The others were considered to have come from outside the couloir. This strategy made it possible to obtain a rockfall inventory for the couloir that included precise rockfall times. It should be noted that rockfall signals with low peaks, which are related to either small rocks/blocks that slide down without impacting the ground or snow-damped rocks/blocks, were thus not necessarily recorded. The results presented in the following sections therefore underestimate the amount of rockfalls, especially the smallest ones.
Each rockfall can be characterised by properties of its seismic signal: its duration, maximum amplitude and energy, which is obtained by integrating the mean seismic envelope between all traces over the whole signal duration. This energy is corrected using the gain of the seismic sensors. These characteristics can also be used to derive volumes (Dietze et al., 2017b; Hibert et al., 2017; Le Roy et al., 2019), which are also a function of the distance to the source, the type of ground impacted (rock, soft soil, snow) and the drop height. That is why the quantification of volumes requires the calibration of the seismic data with an independent source (e.g. a comparison of digital terrain models – DTMs – acquired two years apart; Durand et al., 2018). Such a calibration was not possible here, as only one DTM was acquired by terrestrial laser scanning (TLS, a method based on lidar: light detection and ranging; see Sect. 3.2). However, as the rockfall sources all originated from similar locations, the signal energy could, to the first order, be considered proportional to the volume of the rockfall. It should also be noted that this volume tends to be underestimated when there is snow in the couloir, as this absorbs the impacts.
3.2 Photographic monitoring of the snow cover
In order to study the evolution of the snow cover in the couloir, an automatic camera (Canon EOS Digital Rebel XS, F/13, ISO 200) was installed in June 2016 (Fig. 1). It took 4 photos per day of the couloir over the whole summer period (photos from the camera are presented in Fig. 8). The photos were processed in three steps. First, the images suitable for studying snow (absence of clouds, fog and shadows) were selected manually. Second, in each photo, the pixels associated with snow were detected and isolated. The detection was performed automatically based on the blue channel of the photo (Fedorov et al., 2016), in which the histogram of blue intensities presents two peaks. The first peak corresponds to snow-free pixels and the second peak to snow pixels. The threshold between the two peaks was calculated using the Isodata algorithm (Ridler and Calvard, 1978) and was used to segment the photo into two classes: snow pixels and snow-free pixels. Third, the snow pixels were converted into an area in m2 by a monoplotting technique. The intrinsic (sensor size, focal length, optical centre, distortion) and extrinsic (position and orientation in space) camera parameters were estimated by nonlinear optimisation based on 11 correspondences between real-world coordinates and photo points (Hartley and Zisserman, 2003). Then ray tracing on a DTM was carried out for each pixel in the photo in order to convert the snow pixel extension into metres (Flöry et al., 2020). The DTM was generated with the software CloudCompare (with 1 m accuracy) from a point cloud acquired on 12 September 2016 – with the couloir completely free of snow – by TLS (using an ILRIS LR Optech) of the surroundings of the Tête Rousse refuge (Fig. 1).
The evolution of the snow cover in the couloir was only evaluated from snow-covered surface data, which give biased information on the quantity of liquid water available during melting: the snow-covered surfaces resulting from just a powdering of snow and a fall of several decimetres of snow on the whole couloir will be very similar, whereas the quantities of liquid water resulting from the melting of those surfaces are very different.
3.3 Characterisation of the permafrost thermal state
Continuously measuring the rock surface temperature (RST) for at least 2 full years (Gruber et al., 2004; Magnin et al., 2015) allows the presence/absence of permafrost to be verified. Three Geoprecision PT100.0 sensors (C1, C2 and C3 in Fig. 1) with M-Log5W loggers recording the temperature every 3 h with a resolution of 0.01 ∘C and an accuracy of ±0.1 ∘C were installed out of sunlight (10 cm deep in the rock) in July 2016. To ensure that there was no influence of the air temperature, a silicone seal was placed to block air circulation between the outside air and the hole in which the sensor was installed (Ravanel et al., 2017). The acquired data allowed the analysis of the annual thermal regime of the subsurface and attested the presence/absence of permafrost (Table 1).
3.4 Continuous monitoring of the climber traffic
The number of climbers following the route was continuously monitored from June to September in 2017, 2018 and 2019 using a pyroelectric sensor (Fig. 1; Mourey and Ravanel, 2017) installed at the side of the trail before the section that crosses the couloir. This type of sensor combines passive infrared technology with a high-precision lens to detect the heat emitted by the human body and determine the direction of travel, and has the crucial advantage of not being influenced by weather conditions. Thus, the number of persons that passed the sensor and their directions of travel were recorded continuously, with values produced in 15 min sequences. This means that the number of climbers and their walking directions were known for each quarter of an hour. The margin of error of the sensor was quantified by performing three manual counting sessions at the site each summer. It is important to point out that the sensor indicated the number of times a person passed the sensor and not the number of individuals who climbed Mont Blanc: a climber who climbed up Mont Blanc and came back down was counted twice by the sensor. Moreover, some of the climbers come from other routes, in particular from the Trois Monts Blanc route (via the Aiguille du Midi cable car and the Cosmiques refuge), from the Italian side of Mont Blanc, or from the Aiguille de Bionnassay, and use the Goûter route on the way down.
The implemented multi-method monitoring system led to the construction of one of the few available continuous (day and night, and regardless of the weather conditions) databases on rockfall activity in permafrost conditions, including data on snowfall, precipitation, air and ground surface temperatures and climber traffic. This database covers a continuous period of 68 d from 29 June to 4 September 2019.
4.1 Rockfalls in the Grand Couloir du Goûter during summer 2019
In 2019, 26 339 seismic signals were detected. 2648 were classified as rockfalls located inside the couloir. This gives an average of 39 events per day. A rockfall is thus recorded every 37 min on average, indicating significant geomorphic activity compared to other study sites in high Alpine environments (Ravanel et al., 2017; Hartmeyer et al., 2020). The number of events was lower in the second half of the season: 72 % of the events were recorded in July against 28 % in August.
The energy of an event measured in 2019 was 0.16 MJ on average. Among the events recorded, 88 % had energies lower than the average (Fig. 4). The top 1 % of the events in terms of energy (26 events with an energy > 2.8 MJ) mainly occurred at the end of the summer season. Nineteen of those 26 events occurred after 24 July, among which 14 occurred after 10 August (Fig. 4).
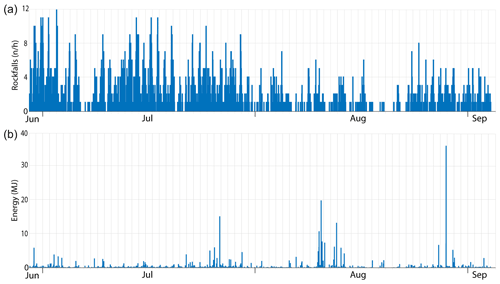
Figure 4Seismic records in 2019. (a) Number of rockfalls per hour. (b) Maximum energy (MJ) of each rockfall.
On average, on a daily time scale, rockfalls were distributed as follows (Fig. 5): the period with the lowest activity was in the morning between 14:00 and 00:00 LT (local time), with the minimum hourly activity occurring between 09:00 and 10:00 LT (1 event every 85 min). The activity then increased markedly between 00:00 and 20:00 LT, with the maximum frequency occurring between 18:00 and 09:00 LT (1 event every 17 min). Then, the frequency decreased progressively until 09:00 LT. The most energetic rockfalls occurred between 15:00 and 22:00 LT, when they were also the most frequent (Fig. 5).
4.2 Air temperature
According to semi-hourly measurements at the Tête Rousse meteorological station at 3126 m a.s.l., the mean temperature over the period 29 June to 4 September was 5.8 ∘C, the maximum temperature was 15 ∘C (24 July) and the minimum temperature was −2.9 ∘C (15 July). Over the whole period, there were only three short periods with negative air temperatures: one of 15 h (15 July), another of 17 h (13 August) and the last of 4 h (13 August). Over the same period, at the top of the couloir, at the level of the Goûter refuge (3817 m a.s.l.), the average temperature was 0.2 ∘C, the maximum temperature was 9.9 ∘C (25 July) and the minimum temperature was −15.9 ∘C (2 August).
4.3 Rock temperature evolution and permafrost distribution
In situ measurements of subsurface temperatures over 2 years (2018 and 2019) confirm the presence of permafrost in the Grand Couloir du Goûter. The lower part of the couloir is located near the lower permafrost limit with an annual mean temperature of −1.1 ∘C (sensor C1; 3345 m a.s.l.). The temperature is lower in the middle and upper parts of the couloir, where the annual mean temperature is −2.8 ∘C at C2 (3460 m a.s.l.) and −3.4 ∘C at C3 (3665 m a.s.l.).
Over the period of rockfall recordings, the number of effective freeze-thaw cycles (EFTCs; Matsuoka, 1990; Seto, 2010) at 10 cm in the rock was very limited. Two EFTCs were recorded by sensor C3 (upper part of the couloir; Fig. 6), while none were recorded by sensors C1 and C2 in the lower and middle parts of the couloir, respectively.
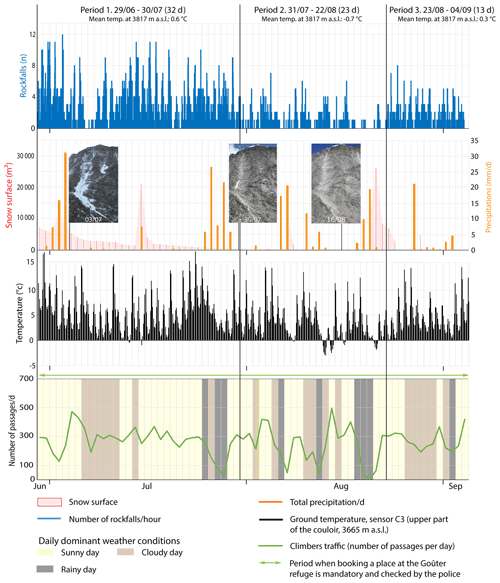
Figure 6Comparison between the number of rockfalls per hour, the evolution of the snow-covered surface, precipitation, ground temperature on the upper part of the couloir (sensor C3) and the daily variation in climber traffic during summer 2019. The images, which were taken with the automatic camera (Sect. 4.2), allowed the evolution of the snow cover in the couloir to be studied.
4.4 Evolution of the snow-covered area
During summer 2019, snow surfaces in the couloir were measured over the period 29 June to 4 September. They showed a progressive decrease between 29 June and 31 July. On the latter date, only a small residual snowpack was still present in the centre of the couloir (Fig. 6). From 1 August to 4 September, the couloir was completely free of snow except for the period 20–25 August, when ∼5 cm of snow fell, but this completely melted in 5 d. On 8 and 28 August, the upper part of the couloir was powdered with snow that melted in a few hours.
4.5 Climber traffic
The results of the climber traffic measurements are presented for the summer season of 2019 between 29 June and 4 September. Over this period, there were 17 768 (±7.2 %) passages on the route, among which 41.5 % were on the way up and 58.5 % on the way down. The daily mean number of climbers was slightly higher in July (271) than in August (248). Over the whole season, the number of climbers is very dependent on the weather. When the weather deteriorates, the number of persons decreases. Conversely, a window of 1 or 2 d of good weather is enough to increase, sometimes significantly, the number of climbers again (Fig. 6). For example, on 12 August, the weather was rainy and there were only 44 passages in the couloir. Only 2 d after (14 August), on the first sunny day following a period of bad weather, the maximum number of daily passages for the season (497) was reached.
On average, there were 261 passages per day, which were organised according to the time profile displayed in Fig. 8a. There are two initial peaks in traffic on the way up, which occur at 02:00–03:00 and 05:00–06:00 LT. These correspond to the two starts from the Tête Rousse refuge. Then there is a main peak between 10:00 and 15:00 LT, corresponding to all the climbers coming from the Mont Blanc tramway. The first train reaches the Nid d'Aigle (2410 m a.s.l.) at 08.30 LT at the earliest. Therefore, the first climbers reach the couloir at around 10:00 LT. On the way down, there is a peak between 07:00 and 15:00 LT, with a maximum between 09:00 and 10:00 LT, which corresponds to all the climbers coming down. As a result, the vast majority of climbers cross the couloir between 10:00 and 14:00 LT, with a peak occurring between 12:00 and 13:00 LT. In 1 h, 12.7 % of the daily total of climbers cross the couloir.
We can distinguish three main periods according to the daily occurrence of rockfalls (Fig. 6). Period 1 covers the first half of the season (29 June to 30 July, i.e. 32 d), which is characterised by the highest rockfall frequency (1916 events, i.e. 1 rockfall every 24 min). Then, Period 2 (31 July to 22 August, i.e. 23 d) presents a clear decrease in the rockfall frequency, with 353 events (i.e. 1 every 94 min). Finally, Period 3 (23 August to 4 September, i.e. 13 d) shows a slight increase in the frequency (379 events measured, i.e. 1 every 49 min). In this section, in order to easily designate a particular period during the season, the rockfall triggering factors during these three periods will be discussed.
5.1 Rockfall triggering factors in the Grand Couloir du Goûter
On a seasonal scale, rockfalls were very numerous during the snow-cover melting period (Figs. 6 and 7b, Period 1). Their frequency of occurrence then sharply and rapidly decreased when snow disappeared in the couloir or when only a small residual firn was still present in the lower part of the couloir at the level of the traverse (Figs. 6 and 7b, Period 2 and 3). Rockfalls were 2.6 times more frequent during Period 1 than during Periods 2 and 3. This finding is in agreement with previous observations made in high Alpine environments (Krautblatter et al., 2013; Draebing et al., 2014, 2017; Weber et al., 2018), which indicated that the first favourable period for rock instability after the cold season is the period of snowpack melt.
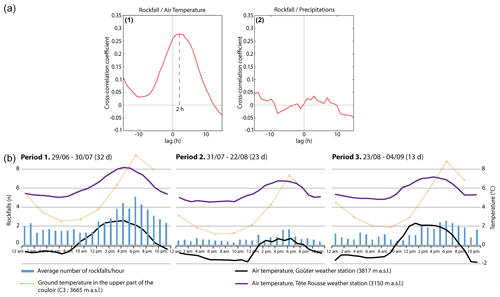
Figure 7(a) Cross-correlation functions for the hourly rate of rockfalls and (1) the hourly temperature recorded at the Goûter refuge (3809 m a.s.l.) and (2) hourly precipitation. The blue dashed line highlights the time delay of 2 h between the hourly temperature and rockfall triggering. (b) Evolution over 24 h, for Periods 1, 2 and 3, of (i) the average number of rockfalls per hour, (ii) the mean hourly ground temperature in the upper part of the couloir (C3), and (iii) the mean hourly air temperatures at the Tête Rousse and the Goûter weather stations.
The most energetic rockfalls occurred during the second part of the season (Periods 2 and 3; 16 out of 26 events; Fig. 4b). The snow potentially dampened the impacts of the blocks in early July. However, as the majority of the couloir was not covered by snow, it can be estimated that a large-scale event would have been measured as such anyway. This finding is in agreement with previous studies on rockfalls in the Mont Blanc massif (e.g. Ravanel and Deline, 2010; Ravanel et al., 2017), which indicate that active-layer thaw leads to an increase in rockfalls mobilising large volumes (rock collapse type, V>100 m3), mostly in sectors where the rock is highly fractured, and particularly at the end of the summer season when the penetration of the seasonal heat into the rock is already well advanced and the active layer is the deepest (Legay et al., 2021). According to those studies, the events mainly occur on slopes of between 40 and 60∘ and between 3400 and 3500 m a.s.l., an altitudinal range where the permafrost often reaches MARST between −2 and 0 ∘C at a depth of 10 cm. Also, the Grand Couloir du Goûter, which is located between 3200 and 3800 m a.s.l. (the lower part of the couloir corresponds to the lower limit of the permafrost; Fig. 1), has a slope angle of 45 to 60∘, is constituted by highly fractured gneiss, and has an active layer that reaches its maximum thickness in September (Pogliotti et al., 2015; Magnin et al., 2017a), brings together conditions that are particularly prone to the occurrence of large rockfalls due to active-layer deepening. Moreover, the most energetic rockfalls occurred mostly (23 out of 26 events) during rainfall events or following, on average, 8.6 mm of precipitation in the last 24 h. This again suggests the key role played by water infiltration in triggering rockfalls, including the largest ones. However, there was no correlation (0.03) between the rainfall quantity during the 24 h before an event and its energy.
In order to quantify the correlations between rockfalls and other parameters (rainfall, temperatures, frequentation) on an hourly scale, we computed the cross-correlation functions between the hourly rockfall rate (R) and the hourly rates of other parameters P, as defined below (Helmstetter and Garambois 2010):
The correlation that mostly explains the hourly rockfall rate is that with the air temperature measured at Goûter station (Fig. 7). The correlation is low (0.28) but significant (much higher than the peak of all the correlations). A time delay of 2 h is found between the temperature time series and the hourly rockfall rate. No clear correlation was found with precipitation at the hourly scale, showing that it was not the dominant mechanism during our period of measurements. The few days with significant rainfall did, however, show higher rockfall activity than on the previous days.
The number of rockfalls started to increase on average 3 h after the return of positive air temperatures at the top of the couloir, with the maximum occurring between 18:00 and 19:00 LT (Fig. 7a). Over the whole season, this maximum occurred, on average, 6 h after the warmest air temperature of the day at the top of the couloir and 2 h after the warmest temperature at Tête Rousse. Conversely, the time of the day with the lowest rockfall frequency was between 09:00 and 00:00 LT, after the coldest period of the day (between 23:00 and 07:00 LT). There was, therefore, a time lag of several hours between the maximum/minimum air temperatures and the rockfalls, which is probably linked to the thermal inertia of the snow and the rock surface and the topographic shading. Moreover, the number of events started to increase when the couloir gradually turned into the sun, which occurred between 12:00 and 13:00 LT at the beginning of the season and between 13;00 and 14:00 LT at the end of the season. The number of rockfalls only started to decrease after 20:00 LT, which corresponds to the moment when the air temperature at the top of the couloir falls below 0 ∘C. The nightly cooling and the daytime thawing at the top of the couloir thus seem to have an effect on the rockfall triggering.
It is likely that nocturnal refreezing (indicated by air temperatures at the Goûter station, and associated with radiative cooling of the surface) only has an impact on the first few centimetres below the rock surface. It probably leads to the cementation of the finest elements only, halting the melting of the snow present during Period 1 and the resulting processes and thus reducing the frequency of rockfalls. On the contrary, the progressive increase in temperature during the morning reactivates the melting of the snow and all the associated thermomechanical processes; in particular, the movement of liquid water into the cracks, which implies an increase in rockfall frequency. The clear link between air/rock temperatures and the rockfall frequency also suggests that some of the rockfalls may have been triggered by conductive expansion of the rock during the warmest hours of the day (Collins and Stock, 2016; Draebing et al., 2017).
According to our field observations, some rockfalls are triggered by the climbers themselves in the upper left part of the couloir. The data acquired do not allow us to precisely measure the anthropogenic part of the rockfalls. However, there are two time periods when the number of rockfalls increases slightly: during the night between 04:00 and 05:00 LT, and in the morning between 08:00 and 09:00 LT, i.e. 2 or 3 h after the first traffic peaks at the bottom of the couloir (at 02:00–03:00 and 05:00–06:00 LT, respectively; Fig. 8a). This time delay of 2–3 h between a peak in the traffic in the couloir and increased rockfall frequency corresponds to the time needed for climbers to reach the upper part of the couloir from the traverse, where they are most likely to trigger rockfalls. Thus, these slight increases in the number of events could be – at least partly – anthropogenic in origin. This observation is supported by the results for specific days when neither snow in the couloir nor precipitation trigger rockfalls. For instance, on 31 July, 7 rockfalls were recorded, and they clearly corresponded to the highest number of climbers, given that they took place mostly in the morning when no other triggering sources occurred (Fig. 8b).
5.2 Climber traffic and rockfalls
The number of climbers following the route did not vary according to the frequency of rockfalls. On a seasonal scale, there was no change in the number of climbers when rockfalls were frequent or – conversely – very rare. In 2019, there were almost as many passages in July (8408) as in August (7708), 2 months with the same number of days with good weather. However, rockfalls were 2.6 times more frequent in July. The number of climbers is mainly determined by the weather and probably by other socioeconomic factors, as well as the management of the route, such as the refuge booking procedures.
On an hourly scale, the traffic peak occurred between 11:00 and 14:00 LT, and the number of rockfalls significantly increases just before that (Fig. 8a). However, climbers, mainly on the way down, continue to frequent the Grand Couloir du Goûter sector until 18:00 LT, which is the time of the day when rockfalls are the most frequent.
These observations, especially the seasonal-scale observations, imply that climbers are not aware of the variations in rockfall frequency and/or that they cannot/will not adapt their behaviour to this hazard. This observation indicates that there is a need to (i) acquire knowledge on rockfalls and their triggering factors in the local context of the Grand Couloir du Goûter and (ii) disseminate this knowledge to mountaineers in order to encourage them to adapt.
5.3 Interest of the mountaineers in the newly acquired knowledge
The knowledge we have acquired, thanks to this study, about the periods of occurrence, the frequency and the triggering factors of rockfalls is key information that should enable mountaineers to better take into account and adapt to the rockfall hazard in the Grand Couloir du Goûter:
-
Results confirm that the crossing of the Grand Couloir du Goûter and the ascent of its left ridge are particularly exposed to rockfalls, with an event occurring every 37 min on average and every 17 min during the peaks in activity between 19:00 and 20:00 LT in 2019. Special attention must therefore be paid to this hazard.
-
The frequency of rockfalls increased on average 3 h after temperatures became positive at the top of the couloir.
-
Rockfalls are more frequent and larger when the couloir becomes exposed to the sun.
-
The time of day when rockfalls are least frequent is between 09:00 and 10:00 LT.
-
Rockfalls are most frequent during periods of snow melt. The presence of snow in the couloir is not a guarantee of safety.
-
The most voluminous rockfalls occur at the end of the summer season.
-
The presence of liquid water is an important factor to take into account when assessing the rockfall hazard in the Grand Couloir du Goûter sector. An increase in liquid water, either from melting snow or from rainfalls, makes rockfalls more likely.
The knowledge acquired through this study therefore allows climbers to better prepare their ascent by choosing the most favourable period to cross the Grand Couloir du Goûter and, once on the field, it enables them to better evaluate the rockfall hazard. This information is sufficiently precise to help climbers to adapt, while it is also accessible to nonspecialists. Moreover, this knowledge promotes effective adaptation based on scientific evidence, not only on personal experience. We can thus formulate two main recommendations for mountaineers. First, they should cross the couloir as early as possible; preferably before midday, when the west face of the Aiguille du Goûter is still in the shade, and not wait – as some climbers do – until the end of the afternoon or the beginning of the evening (for cooler air), which is the most dangerous period of the day, in order to avoid arriving too early at the refuge. Second, although mountaineers consider periods when there is snow to be the most favourable periods, the presence of snow is not a guarantee of safety in the local context of the Grand Couloir du Goûter, especially during melting periods.
In 2020, thanks to the initiative of the French Federation of Alpine and Mountain Clubs (FFCAM), which manages the three refuges located on this route (Goûter, Tête Rousse, and Nid d'Aigle), web cameras facing the couloir (one picture every minute) and air-temperature sensors were installed on the roofs of the Goûter and Tête Rousse refuges. Live data are available free on the websites of the refuges, and allow a better evaluation of the conditions in the couloir (https://montblanc.ffcam.fr/webcams-tete-rousse.html, last access: 6 August 2021).
5.4 Dissemination of the acquired knowledge to the mountain community and implementation of management measures for the route
In order to promote better knowledge of and adaptation to the danger from rockfalls in the Grand Couloir du Goûter among mountaineers to try to reduce the number of accidents in this sector, we already have widely disseminated the knowledge we have acquired from this study within the mountaineering community.
A study report has been published and is available for free in French and English on the Petzl Foundation website (https://www.petzl.com/fondation/projets/accidents-couloir-gouter?language=en, last access: 6 August 2021). It was presented during a webinar organised by the Petzl Foundation in June 2020, notably to the press and mountain stakeholders (Mountaineering Federation, Unions of Alpine Guides, Alpine Clubs, National School for Skiing and Mountaineering, journalists specialising in mountaineering, etc.; 70 people attended), which led to the publication of several papers. To accompany the publication of this report, a 4 min film (https://www.petzl.com/fondation/projets/accidents-couloir-gouter?language=en, last access: 6 August 2021) presenting the main results and the recommendations to climbers, and inviting them to better take into account and adapt to the local rockfall hazard, was produced in collaboration with the Petzl Foundation. It was presented at the Science and Mountain festival in Grenoble (shown twice, 60 000 spectators in total) and, in addition, has been seen more than 600 000 times on the internet (Petzl Foundation, personal communication, 2021).
Moreover, our results were used by local and regional authorities, the FFCAM and the military forces in charge of rescue operations to define and implement management measures for the attendance in summer 2020. The objectives of these measures were, among others, to reduce the number of accidents in the Grand Couloir du Goûter and to encourage the adaptation of mountaineers to the local rockfall hazard. These measures consist of making reservations in the refuges mandatory in order to better distribute the number of climbers over the entire season and to encourage an ascent of Mont Blanc in 3 d instead of 2 d, which enables the climbers to cross the couloir very early in the morning on the way up and on the way down. A 3 d ascent involves spending the first night either at the refuge of Tête Rousse or at the Nid d'Aigle and crossing the couloir on the way up very early in the morning of the second day, and then sleeping at the Goûter refuge on the way down from the summit and thus crossing the couloir on the way down very early in the morning of the third day. The knowledge acquired in this study is also taught during the training of French and Italian Alpine guides.
At this point, it is impossible to know how effective our work has been in changing climber behaviour, and how effective the route management measures have been in reducing the number of accidents. However, the fact that our results have been widely downloaded, that they have received considerable attention from the press, and that they have been used by the actors in charge of the management of the route shows that they respond to a need for knowledge and that they promote the implementation of concrete actions for adaptation. These adaptation measures are all the more important given that it has been estimated that, in the future, climate change will lead to an increased rockfall hazard in high Alpine environments.
The classic route up Mont Blanc (4809 m a.s.l.) is very popular (20 000 climbers per summer season), but the accident rate in the Grand Couloir du Goûter is also very important (3.7 deaths per year since 1990). Rockfalls, which are increasingly frequent in the couloir (Mourey et al., 2019), are one of the main factors that explain this high accident rate. This particular context justified the present study, which led to the acquisition of new knowledge of the rockfall activity and the triggering factors at this location, which is useful for encouraging mountaineers to adapt to the local rockfall hazard. Our results confirm previous studies that showed a seasonal time scale of rockfalls in permafrost-affected rockwall: events are more frequent during the snowmelt period. On a daily scale, there is a clear correlation between rockfall frequency and air temperature, with a 2 h delay between the peak in temperature and the peak in rockfall activity. Our data also confirmed field observations indicating that mountaineers can trigger rockfalls. These results are key information for identifying the best moment to cross the couloir. On a seasonal time scale, it is particularly important to be wary of the periods of snow melt and, on a daily scale, it is important to try to cross the couloir at the end of the night or beginning of the morning, when rockfalls are less frequent.
However, our results also showed that climbers are not aware of the variations in rockfall frequency and/or that they cannot/will not adapt their behaviour to this hazard. The results obtained have been disseminated to the mountain community and used to implement management measures for the route, which confirms the interest in/need for the knowledge acquired here to promote the adaptation of mountaineers. However, the effectiveness of these management measures, particularly in reducing the number of accidents, was not examined in this study.
This study is an example of the operational scope of scientific work and its capacity to promote adaptation behaviours. Similar case studies could be implemented for other sites with high stakes for mountaineering. One example is the emblematic and popular route of the Meije traverse (Écrins massif, southern Alps), which has become very dangerous since a rock collapse in 2018 and the gradual melting of an ice apron. A better understanding of the occurrence of rockfalls in and the future evolution of this sector, particularly in relation to the melting of the ice apron, is a major heritage and economic issue for the mountaineers in this sector. Another potential site should be the eastern ridge of the Aiguille du Midi (3842 m a.s.l., MBM), which is one of the main accesses to the high mountains in the MBM.
All calculations on seismic signals were performed using MatLab. The used code is described in the reference Lacroix and Helmstetter (2011) and is available from the corresponding author upon request. The code used to analyse the evolution of snow cover from images was developed for the private company Tenevia. It is therefore not publicly available. For more information, contact the company Tenevia (https://www.tenevia.com/en/, last access: 14 January 2022).
Air temperature data from the Goûter meteorological station were acquired by the private company REQUEA. The data are therefore not publicly accessible. For more information, contact the company REQUEA (https://www.requea.com, last access: 14 January 2022).
The other data used in this article (number of rockfalls, climber traffic, air temperature at Tête Rousse, pluviometry, ground temperature and snow surface) are available at https://doi.org/10.5281/zenodo.6074472 (Mourey et al., 2022).
JM, PL and PAD conceived the study. JM, PL and PAD realised most of the field missions and analysed the data from different sensors (JM: weather stations and traffic sensor; PL: seismic sensors; PAD: ground-temperature sensors and lidar processing). JM analysed the full set of data and wrote the article with contributions to the analysis from PL, PAD and LR. GM and MM participated in some of the field missions and performed the analysis of the snow-covered surfaces. LR acquired the DTM on the field and contributed to the funding of the study. EM participated in most of the field missions, designed the automatic camera system, and his technical skills were indispensable for the installation and maintenance of all the measurement systems and data storage.
The contact author has declared that neither they nor their co-authors have any competing interests.
Publisher's note: Copernicus Publications remains neutral with regard to jurisdictional claims in published maps and institutional affiliations.
The authors warmly thank Olivier Moret and the Petzl Foundation for their support, Didier Hantz (ISTerre) and Florence Magnin (EDYTEM) for their help in analysing the data, Pierre Dubois (REQUEA) for giving us access to data from their weather station, Antoine Rabatel (IGE) for lending the OSUG long-range lidar, Emmanuel Thibert (INRAE) for access to the data from the Tête Rousse weather station, and Andreas Aspass (UiO) for the English proofreading. We also thank Marc Whatelet, Axel Jung, Sandrine Roussel and Laurent Métral (ISTerre) for their help in the field and in setting up the seismometers, and Bertrand Guillet for lending us the seismic measuring equipment. We would also like to thank the town of Saint-Gervais for its support in carrying out this work, the Compagnie du Mont-Blanc for granting us free access to the site, and all the people who helped us in the field to carry and set up the equipment.
This research has been supported by the EU ALCOTRA AdaPT Mont-Blanc project and the Petzl Foundation.
This paper was edited by Sven Fuchs and reviewed by Arnaud Temme and three anonymous referees.
Alpes Ingé: Couloir du Goûter, Suivi et analyse des chutes de blocs et de la fréquentation pendant l'été 2011, Rapport final, Fondation Petzl, 37 pp., 2012.
Collins, B. and Stock, G.: Rockfall triggering by cyclic thermal stressing of exfoliation fractures, Nat. Geosci., 9, 345–500, https://doi.org/10.1038/NGEO2686, 2016.
Dammeier, F., Moore, J., Haslinger, F., and Loew, S.: Characterization of alpine rockslides using statistical analysis of seismic signals, J. Geophys. Res., 116, F04024, https://doi.org/10.1029/2011JF002037, 2011.
Debarbieux, B.: L'Unesco au mont Blanc, Chamonix, Guérin, France, https://archive-ouverte.unige.ch/unige:130712 (last access: 14 February 2022), 2020.
DeRoin, N. and Mc Nutt, S.: Rockfalls at Augustino Volcano, Alaska: The influence of eruption precursors and seasonal factors on occurrence patterns 1997–2009, J. Volcanol. Geoth. Res., 211–212, 61–75, https://doi.org/10.1016/j.jvolgeores.2011.11.003, 2012.
Dietze, M., Mohadjer, S., Turowski, J., Ehlers, T., and Hovius, N.: Seismic monitoring of small alpine rockfalls – validity, precision and limitations, Earth Surf. Dynam., 5, 653–668, https://doi.org/10.5194/esurf-5-653-2017, 2017a.
Dietze, M., Turowski, J., Cook, K., and Hovius, N.: Spatiotemporal patterns, triggers and anatomies of seismically detected rockfalls, Earth Surf. Dynam., 5, 757–779, https://doi.org/10.5194/esurf-5-757-2017, 2017b.
Draebing, D., Krautblatter, M., and Dikau, R.: Interaction of thermal and mechanical processes in steep permafrost rock walls: a conceptual approach, Geomorphology, 226, 226–235, https://doi.org/10.1016/j.geomorph.2014.08.009, 2014
Draebing, D., Krautblatter, M., and Hoffmann, T.: Thermo-cryogenic controls of fracture kinematics in permafrost rockwalls, Geophys. Res. Lett., 44, 3535–3544, https://doi.org/10.1002/2016GL072050, 2017.
Durand, V., Mangeney, A., Haas, F., Jia, X., Bonilla, F., Peltier, A., Hibert, C., Ferrazzini, V., Kowalski, P., Lauret, F., Brunet, C., Satriano, C., Wegner, K., Delorme, A., and Villeneuve, N.: On the link between external forcings and slope instabilities in the Piton de la Fournaise summit crater, Reunion island, J. Geophys. Res.-Earth, 123, 2422–2442, https://doi.org/10.1029/2017JF004507, 2018.
Dussauge, C., Grasso, J.-R., and Helmstetter, A.: Statistical analysis of rockfall volume distributions: implications for rockfall dynamics, J. Geophys. Res., 108, 2286, https://doi.org/10.1029/2001JB000650, 2003.
Fedorov, V., Arias, P., Facciolo, G., and Ballester, C.: Affine Invariant Self-similarity for exemplar-based Inpainting, in: 11th joint Conference on Computer Vision, Imag. Comput. Graph. Theory Appl., 3, 50–60, https://doi.org/10.5220/0005728100480058, 2016.
Flöry, S., Ressl, C., Hollaus, M., Pürcher, G., Piermattei, L., and Pfeifer, N.: “WEBSNOW”: Estimation Of Snow Cover From Freely Accessible Webcam Images In The Alps, ISPRS Ann. Photogramm. Remote Sens. Spatial Inf. Sci., V-2-2020, 695–701, https://doi.org/10.5194/isprs-annals-V-2-2020-695-2020, 2020.
Gardent, M., Rabatel, A., Dedieu, J. P., and Deline, P.: Multitemporal glacier inventory of the French Alps from the late 1960s to the late 2000s, Global Planet. Change, 120, 24–37, https://doi.org/10.1016/j.gloplacha.2014.05.004, 2014
Gruber, S. and Haeberli, W.: Permafrost in steep bedrock slopes and its temperature-related destabilization following climate change, J. Geophys Res., 112, F02S18, https://doi.org/10.1029/2006JF000547, 2007.
Gruber, S., Hoelzle, M., and Haeberli, W.: Permafrost thaw and destabilization of Alpine rock walls in the hot summer 2003, Geophys. Res. Lett., 31, L13504,, https://doi.org/10.1029/2004GL020051, 2004.
Guillermot, A., Helmstetter, A., Larose, E., Baillet., L., Garambois, S., Mayoraz, R., and Delaloye, R.: Seismic monitoring in the Gugla rock glacier (Switzerland): ambiant noise correlation, microseismicity and modelling, Geophys. J. Int., 221, 1719–1735, https://doi.org/10.1093/gji/ggaa097, 2020.
Hartley, R. and Zisserman, A.: Multiple view geometry in computer vision, Cambridge University Press, 48 pp., https://www.cambridge.org/ch/academic/subjects/computer-science/computer-graphics-image-processing-and-robotics/multiple-view-geometry-computer-vision-2nd-edition?format=PB&isbn=9780521540513 (last access: 14 February 2022), 2003.
Hartmeyer, I., Delleske, R., Keusching, M., Krautblatter, M., Lang, A., Schrott, L., and Otto, J.-C.: Current glacier recession causes significant rockfall increase: the immediate paraglacial response of defglaciating cirque walls, Earth Surf. Dynam., 8, 729–751, https://doi.org/10.5194/esurf-8-729-2020, 2020.
Hasler, A., Gruber, S., Font, M., and Dubois, A.: Advective heat transport in Frozen Rock Clefts: conceptual model, laboratory experiments and numerical simulation, Permafrost Periglac. Process., 22, 378–389, https://doi.org/10.1002/ppp.737, 2011.
Helmstetter, A. and Garambois, S.: Seismic monitoring of Séchilienne rockslide (French Alps): Analysis of seismic signals and their correlation with rainfalls, J. Geophys. Res., 15, F03016, https://doi.org/10.1029/2009JF001532, 2010.
Hibert, C., Mangeney, A., Grandjean, G., and Shapiro, N. M.: Slope instabilities in Dolomieu crater, Réunion Island: from seismic signals to rockfall characteristics, J. Geophys. Res., 116, F04032, https://doi.org/10.1029/2011JF002038, 2011.
Hibert, C., Malet, J.-P., Bourrier, F., Provost, F., Berger, F., Bornemann, P., Tardif, P., and Mermin, E.: Single-block rockfall dynamics inferred from seismic signal analysis, Earth Surf. Dynam., 5, 283–292, https://doi.org/10.5194/esurf-5-283-2017, 2017.
Hibert, C., Michéa, D., Provost, F., Malet, J.-P., and Geertsema, M.: Exploration of continuous seismic recordings with a machine learning approach to document 20 yr of landslide activity in Alaska, Geophys. J. Int., 219, 1138–1147, https://doi.org/10.1093/gji/ggz354, 2019.
Krautblatter, M., Funk, D., and Günzel, F. K.: Why permafrost rocks become unstable: a rock-ice-mechanical model in time and space, Earth Surf. Proc. Land., 38, 876–887, https://doi.org/10.1002/esp.3374, 2013.
Lacroix, P. and Helmstetter, A.: Location of Seismic Signals Associated with Microearthquakes and Rockfalls on the Séchilienne Landslide, French Alps, Bull. Seismol. Soc. Am., 101, 341–353, https://doi.org/10.1785/0120100110, 2011.
Legay, A., Magnin, F., and Ravanel L.: Rock temperature prior to failure: analysis of 209 rockfall events in the Mont Blanc massif (Western European Alps), Permafrost Periglac. Process., 32, 520–536, https://doi.org/10.1002/ppp.2110, 2021.
Lemarchal, D.: EURL Meije: Massif du Mont-Blanc, Traversée du Grand Couloir, Etude de faisabilité et avant-projet de sécurisation, Fondation Petzl, 87 pp., 2011.
Le Roy, G., Helmstetter, A., Amitrano, D., Guyoton, F., and Le Roux-Mallouf, R.: Seismic analysis of the detachment and impact phases of rockfalland application for estimating rockfall volume and free-fall height, J. Geophys. Res.-Earth, 124, 2602–2622, https://doi.org/10.1029/2019JF004999, 2019.
Magnin, F., Brenning, A., Bodin, X., Deline, P., and Ravanel, L.: Statistical modelling of rock wall permafrost distribution: application to the Mont Blanc massif, Geomorphologie, 21, 145–162, https://doi.org/10.4000/geomorphologie.10965, 2015.
Magnin, F., Josnin, J.-Y., Ravanel, L., Pergaud, J., Pohl, B., and Deline, P.: Modelling rock wall permafrost degradation in the Mont Blanc massif from the LIA to the end of the 21st century, The Cryosphere, 11, 1813–1834, https://doi.org/10.5194/tc-11-1813-2017, 2017a.
Manconi, A., Picozzi, M., Coviello, V., De Santis, F., and Elia, L.: Real time detection, location and characterization of rockslides using broadband regional seismic networks, Geophys. Res. Lett., 43, 6960–6967, https://doi.org/10.1002/2016GL069572, 2016.
Matsuoka, N.: Mechanisms of rock breakdown by frost action – an experimental approach, Cold Reg. Sci. Technol., 17, 253–270, https://doi.org/10.1016/S0165-232X(05)80005-9, 1990.
McColl, S. T. and Draebing, D.: Rock slope instability in the proglacial zone: State of the Art, in: Geomorphology of proglacial systems – Landform and sediment dynamics in recently deglaciated alpine landscapes, edited by: Heckmann, T. and Morche, D., Springer, Heidelberg, 119–141, https://doi.org/10.1007/978-3-319-94184-4_8, 2019.
McDowell, G., Stephenson, E., and Ford, J.: Adaptation to climate change in glaciated mountain regions, Climatic Change, 126, 77–91, https://doi.org/10.1007/s10584-014-1215-z, 2019.
Mennessier, G., Carme, F., Bellire, J., Dhellemes, R., Antoine, P., Dabrowski, H., and Meloux, J.: Notice explicative: Carte Géologique de la France à 1:50 000, Feuille ST-Gervais-les-Bains, http://ficheinfoterre.brgm.fr/Notices/0703N.pdf (last access: 14 February 2022), 1977.
Mourey, J. and Ravanel, L.: Measuring the attendance of access routes to high mountain in the Mont-Blanc massif using pyroelectric sensors, Collection EDYTEM SI Monitoring en milieux naturels, retours d'expériences en terrains difficiles, 263–270, https://doi.org/10.3406/edyte.2017.1394, 2017.
Mourey, J., Moret, O., Descamps, P., and Bozon, S.: Accidentology of the normal route up Mont Blanc between 1990 and 2017, Fondation Petzl, 20, https://www.petzl.com/fondation/projets/accidents-couloir-gouter?language=en (last access: 13 July 2021), 2018.
Mourey, J., Marcuzzi, M., Ravanel, L., and Pallandre, F.: Effects of climate change on high Alpine environments: evolution of mountaineering routes in the Mont Blanc massif (Western Alps) over half a century, Arct. Antarct. Res., 51, 176–189, https://doi.org/10.1080/15230430.2019.1612216, 2019.
Mourey, J., Lacroix, P., Duvillard, P.-A., Marsy, G., Marcer, M., Malet, E., and Ravanel, L.: Multi-method monitoring of rockfall activity along the classic route up Mont Blanc (4809 m a.s.l.) to encourage adaptation by mountaineers [data set], In Natural Hazards and Earth System Sciences, Zenodo [data set], https://doi.org/10.5281/zenodo.6074472, 2022.
NRCC (Permafrost Subcommittee): Glossary of permafrost and related ground-ice terms, Technical memorandum 142, National Research Council of Canada, 156 pp., https://globalcryospherewatch.org/reference/glossary_docs/permafrost_and_ground_terms_canada.pdf (last access: 14 February 2022), 1988.
Paul, F., Rastner, P., Azzoni, R. S., Diolaiuti, G., Fugazza, D., Le Bris, R., Nemec, J., Rabatel, A., Ramusovic, M., Schwaizer, G., and Smiraglia, C.: Glacier shrinkage in the Alps continues unabated as revealed by a new glacier inventory from Sentinel-2, Earth Syst. Sci. Data, 12, 1805–1821, https://doi.org/10.5194/essd-12-1805-2020, 2020.
Pogliotti, P., Guglielmin, M., Cremonese, E., Morra di Cella, U., Filippa, G., Pellet, C., and Hauck, C.: Warming permafrost and active layer variability at Cime Bianche, Western European Alps, The Cryosphere, 9, 647–661, https://doi.org/10.5194/tc-9-647-2015, 2015.
Pröbstl-Haider, U., Dabrowska, K., and Haider, W.: Risk perception and preferences of mountain tourists in light of glacial retreat and permafrost degradation in the Austrian Alps, J. Outdoor Recreat. Tourism, 13, 66–78, https://doi.org/10.1016/j.jort.2016.02.002, 2016.
Purdie, H. and Kerr, T.: Aoraki Mont Cook : Environmental change on an iconic mountaineering route, Mount. Res. Dev., 38, 364–379, https://doi.org/10.1659/MRD-JOURNAL-D-18-00042.1, 2018.
Ravanel, L. and Deline, P.: Climate influence on rockfalls in high-Alpine steep rockwalls: The north side of the Aiguilles de Chamonix (Mont Blanc massif) since the end of the `Little Ice Age', Holocene, 21, 357–365, https://doi.org/10.1177/0959683610374887, 2010.
Ravanel, L., Magnin, F., and Deline, P.: Impacts of the 2003 and 2015 summer heatwaves on permafrost-affected rock-walls in the Mont Blanc massif, Sci. Total Environ., 609, 132–143, https://doi.org/10.1016/j.scitotenv.2017.07.055, 2017.
Ridler, T. W. and Calvard, S.: Picture thresholding using an iterative selection method, IEEE Trans. Syst. Man Cybern., 8, 630–632, https://doi.org/10.1109/TSMC.1978.4310039, 1978.
Ritter, F., Fiebig, M., and Muhar, A.: Impacts of Global Warming on Mountaineering: A Classification of Phenomena Affecting the Alpine Trail Network, Mount. Res. Dev., 32, 4–15, https://doi.org/10.1659/MRD-JOURNAL-D-11-00036.1, 2011.
Seto, M.: Freeze thaw cycles on rock surfaces below the timberline in a montane zone: field measurements in Kobugahara, Northern Ashio Mountains, Central Japan, Catena, 82, 218–226, https://doi.org/10.1016/j.catena.2010.06.006, 2010.
Soulé, B., Lefèvre, B., Boutroy, E., Reynier, V., Roux, F., and Corneloup, J.: Accidentologie des sports de montagne, Etats des lieux et diagnostic, Fondation Petzl, https://www.petzl.com/fondation/s/accidentologie-des-sports-de-montagne?language=en_US (last access: 25 June 2021), 2014.
Temme, A. J. A. M.: Using Climber's Guidebooks to Assess Rock Fall Patterns Over Large Spatial and Decadal Temporal Scales: An Example from the Swiss Alps, Geograf. Ann. A, 97, 793–807, https://doi.org/10.1111/geoa.12116, 2015.
Van Everdingen, R.: Multi-language glossary of permafrost and related ground-ice terms, National Snow and Ice Data Center/World Data Center for Glaciology, Boulder, CO, USA, updated 2005, https://globalcryospherewatch.org/reference/glossary_docs/Glossary_of_Permafrost_and_Ground-Ice_IPA_2005.pdf (last access: 3 July 2021), 1998.
Vincent, C., Thibert, E., Gagliardini, O., Legchenko, A., Gilbert, A., Garambois, S., Condom, T., Baltassat, J. M., and Girard, J. F.: Mechanisms of subglacial cavity filling in Glacier de Tête Rousse, French Alps, J. Glaciol., 61, 609–623, https://doi.org/10.3189/2015JoG14J238, 2015.
Weber, S., Faillettaz, J., Meyer, M., Beutel, J., and Vieli, A.: Acoustic and Microseismic Characterization in Steep Bedrock Permafrost on Matterhorn (CH), J. Geophys. Res.-Earth, 123, 1363–1385, https://doi.org/10.1029/2018JF004615, 2018.