the Creative Commons Attribution 4.0 License.
the Creative Commons Attribution 4.0 License.
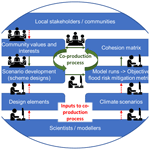
Invited perspectives: Managed realignment as a solution to mitigate coastal flood risks – optimizing success through knowledge co-production
Hannah L. Mossman
Harriet E. Moore
Elizabeth Christie
Joshua Kiesel
Nature-based solutions are increasingly suggested for mitigating coastal flood risks in the face of climate change. Managed realignment (MR), a coastal adaptation strategy that entails the landward realignment of coastal defences to restore coastal habitats (often salt marshes), plays a pivotal role in implementing nature-based solutions in the coastal zone. Across Europe, more than 130 sites have been implemented so far, often to harness their potential to mitigate coastal flood risks while restoring coastal habitats (ABPmer, 2021). However, local communities often oppose MR projects, not only because they are seen as returning hard-won land to the sea but also because their coastal protection function is less trusted than traditional hard engineering techniques. This scepticism has foundation. The proclaimed coastal protection function of MRs is based on a broad body of literature on the protective function of natural salt marshes. However, contrary to natural salt marshes, MRs are often semi-enclosed tidal basins with narrow breaches to the open sea/estuary. Recent studies indicate that MR-internal hydrodynamics may significantly reduce their coastal protection, depending on their engineering design. To successfully implement MR, a much-improved scientific knowledge base is needed, as well as a process for addressing community concerns and genuinely engaging stakeholders in decision-making beyond the usual obligatory consultancy approach. Here, we propose the co-production of scientific knowledge with local communities and stakeholders to optimize the success of coastal nature-based solutions and promote community acceptance.
-
Please read the editorial note first before accessing the article.
-
Article
(2190 KB)
-
Please read the editorial note first before accessing the article.
Global sea-level rise (SLR) is one of the most certain and long-lasting consequences of climate change; by 2300 it is expected that global sea levels will rise by 0.3 m in a best-case scenario and 16 m in a worst-case scenario (Fox-Kemper et al., 2021). Globally, coastal communities are suffering from the impacts of SLR, from both increased coastal erosion (Vousdoukas et al., 2020) and coastal flooding (Hinkel et al., 2014). At the same time, natural buffer zones such as salt marshes and mangroves have been lost on a large scale, mostly due to land reclamation and embankment construction (Gedan et al., 2009; Lotze et al., 2006). Nature-based solutions (NBSs) to climate change challenges are gaining in popularity amongst coastal managers due to their proclaimed cost effectiveness compared to traditional engineering solutions and their multiple co-benefits (MacDonald et al., 2020; van Zelst et al., 2021). Managed realignment (MR), for example, is a widespread type of NBS, where existing sea defences are realigned inland to create intertidal habitat, mostly salt marshes, in the intervening space (Esteves, 2014). They are widely praised for their role in compensating coastal wetlands for anthropogenically induced losses elsewhere (Morris, 2013) and for their ability to reduce coastal wave and storm surge heights and hence to enhance coastal protection levels and/or to reduce coastal protection costs (Möller, 2019; Roca and Villares, 2012; van Zelst et al., 2021; Wamsley et al., 2010). Available types of MR include the complete removal of the original sea defence, the punctual breaching of the original defence (one or several breaches) through either active management or accidental (unmanaged realignment), and regulated tidal exchange (where the tidal regime within the MR is controlled through sluices). So far, at least 22 000 ha of MR has been implemented globally, with an average scheme size of 161 ha, but there is a large variability between different regions (Fig. 1; ABPmer, 2021). Amongst these schemes, 36 (14 522 ha) are managed via/by regulated tidal exchange. In the UK, the country with the largest number of MRs and RTEs, by November 2021 a total of 77 schemes had been implemented with an average scheme size of 39 ha, whereas in China a total of 3 reported schemes had been implemented with an average size of 3079 ha (ABPmer, 2021).
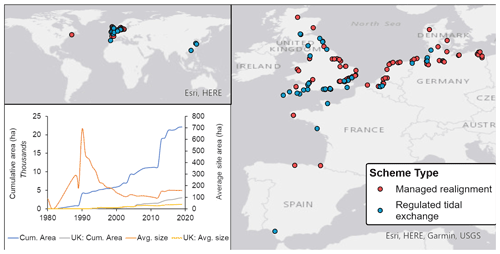
Figure 1Spatial distribution of managed realignments (red dots) and regulated tidal exchange schemes (blue dots) globally (upper left panel) and in Europe (right panel). Lower left panel: cumulative areas (ha) of coastal managed realignments and regulated tidal exchange globally (blue) and in the UK (grey) and average scheme sizes (ha) for sites globally (orange) and in the UK (yellow). Data source: ABPmer (2021).
While natural salt marshes on open NW European coasts have been shown to reduce wave heights of extreme storms (Möller et al., 2014), and natural salt marshes covering extensive areas (e.g. Mississippi Delta) have been shown to reduce storm surge inundations (Wamsley et al., 2010), the energy dissipating potential of salt marshes within MR sites is much less understood. This potential may differ from natural marshes due to the MRs' artificially semi-enclosed nature related to their specific scheme design (Kiesel et al., 2020). The few targeted studies on the effectiveness of MR to mitigate coastal flood risks suggest that only larger schemes may be effective, while small salt marshes and MRs may even amplify coastal water levels (Kiesel et al., 2020; Stark et al., 2016). Such large schemes particularly require the endorsement and trust from coastal communities as coastal space is often scarce where increased coastal protection is needed. However, the implementation of MR is often perceived negatively by coastal communities and faces societal opposition, primarily because MR means giving previously used/cultivated land back to the sea. This opposition has cultural and socio-economic causes (Goeldner-Gianella, 2007; Rupp-Armstrong and Nicholls, 2007) but is also an indicator for a profound mistrust in the effectiveness of NBSs to mitigate coastal flood risks (Möller, 2019; Roca and Villares, 2012).
The effective design of any project requires a sound scientific understanding of the biophysical processes involved, while successful implementation usually depends in part on how well projects represent the needs of multiple stakeholders, including local communities. However, stakeholder engagement and participatory approaches in natural resource management, including flood management, have long been critiqued for offering only tokenistic opportunities for communities to contribute (Blunkell, 2017). Community involvement is often limited to data collection (e.g. citizen science), and the engagement process usually begins too late in the cycle of project design to allow for more than very basic consultation (Few et al., 2007). As a result, designing projects based on robust science and community engagement usually occur in isolation, with project design taking place much earlier than community consultation.
Further research is needed to investigate the use of MR as an NBS to address rising sea levels in the context of climate change. These NBS projects, and others that will undoubtedly emerge from efforts to adapt to climate change, are ideal opportunities for genuine knowledge co-production, embracing the best-practice principles of scientific practice and participation. In this perspectives piece, we discuss four current challenges around the implementation of MR to mitigate flood risks and suggest that developing a robust scientific basis for flood mitigation and effective participation can occur in parallel rather than separately. Specifically, we propose that involving stakeholders in the design of projects, as well as later phases of implementation, may facilitate more meaningful participation than traditional approaches to community engagement and produce more effective MR schemes.
The argument that MR schemes are efficient in mitigating coastal flood risks originates from a broad body of literature on natural coastal wetlands (Fairchild et al., 2021; Loder et al., 2009; Paquier et al., 2017; Smolders et al., 2015; Stark et al., 2015, 2016). Natural wetlands have been shown to be effective in reducing relative SLR (RSLR), particularly where RSLR rates have historically been compounded by anthropogenic subsidence and the disconnection of coastal lowlands from riverine and marine sediment sources, such as river deltas or estuaries (Temmerman and Kirwan, 2015; Temmerman et al., 2013). Kirwan et al. (2016) and Coleman et al. (2022), for example, show that tidal marshes globally are usually accreting sediment at the same, or a higher, pace than current local RSLR. They further show that low-elevation marshes are more efficient in accreting sediment vertically than high-elevated marshes because low-elevation sites are inundated more frequently, allowing for more sediment to be deposited. This negative feedback mechanism between marsh elevation and sediment accretion makes tidal marshes ideal landscapes to reduce RSLR rates and mitigate permanent inundation of coastal lowlands (Temmerman and Kirwan, 2015; Temmerman et al., 2013). The conservation and restoration of coastal wetlands is therefore thought to be essential for the protection against large-scale land losses under the projected climate change scenarios, e.g. as suggested for the Mississippi Delta (Fischbach et al., 2019).
The RSLR-reducing effect of coastal wetlands, however, does not merely reduce land losses but also increases the dissipation of storm surge and wave energies (Shepard et al., 2011; van Zelst et al., 2021), primarily caused by reduced water depths and increased vegetation-induced surface roughness (Möller, 2006; Wamsley et al., 2010). Particularly well established is the effect of salt marshes to attenuate coastal wave heights. Möller et al. (2014) measured a 12 %–20 % reduction in wave heights over a 40 m stretch of salt marsh and attributed 40 %–60 % of this attenuation to the presence of salt marsh vegetation. On most coastlines with salt marshes present, the overall wave attenuation may lead to a full attenuation of waves when they reach the coastline (Yang et al., 2012), hence reducing the risk of coastal flooding from wave overtopping and the pressure on coastal defences (van Zelst et al., 2021). Moreover, salt marshes are effective in reducing coastal erosion, a potential indirect cause of coastal flooding (Pollard et al., 2019), through increasing the sediment's shear strength and the potential protective function of flexible vegetation (Möller et al., 2014). Where salt marshes are eroded, coastal wave heights are expected to increase due to reduced surface roughness and a reduction in foreshore elevations associated with increased water depths and wave heights (Fagherazzi and Wiberg, 2009).
Besides their wave-height reducing effect, salt marshes are also reported to significantly reduce still water levels during storm surges. However, the range of reported attenuation rates varies greatly between 1.7 and 70 cm km−1 (Vafeidis et al., 2019), with most of the field and modelling evidence available for the Mississippi Delta, where vast areas of salt marsh exist. Meanwhile, the attenuation values reported for storm surge heights over smaller salt marshes are less conclusive. Some studies suggest the existence of a critical marsh width, below which salt marshes may lose their capacity to attenuate storm surge heights (Stark et al., 2016; Kiesel et al., 2022). Moreover, the ratio of subtidal to intertidal areas within a salt marsh (manifested mostly in the volume of the tidal creek network) and the storm duration and surge height appear to negatively impact the capacity of salt marshes to attenuate storm water levels (Stark et al., 2016; Loder, et al., 2009; Wamsley et al., 2010). For the highest water levels, even amplification of storm water levels has been reported (Stark et al., 2015). Water level amplification is thereby attributed to the reflection of the tidal wave against the sea defence, located at the landward edge of the salt marsh and truncating the natural marsh extent (Stark et al., 2016; Kiesel et al., 2022; Resio and Westerink, 2008; Wamsley et al., 2009). The latter highlights the importance to current and future salt marsh management to ensure the maintenance of salt marsh elevations and lateral extents, particularly under projected rates of future SLR (Reed et al., 2018).
A key process driving the SLR-induced loss and truncation of salt marshes globally is coastal squeeze (Schuerch et al., 2018), i.e. “intertidal habitat loss which arises due to the high water mark being fixed by a defence and the low water mark migrating landwards in response to sea level rise” (Pontee, 2013, p. 206). Managed realignment is widely considered to be a key management strategy to counteract coastal squeeze and restore the salt marshes' coastal protection function where this has been reduced as a consequence of SLR-induced habitat size reduction (Doody, 2013). However, we argue that the above-outlined uncertainties around the effectiveness of salt marshes in reducing coastal flood risks are even larger and less studied for such MR schemes than for natural salt marshes.
Managed realignments are considered an important management option to mitigate the loss of coastal salt marshes to coastal squeeze (Doody, 2013; Morris, 2013). The provisioning of additional accommodation space for salt marshes to establish is especially efficient in areas where historic land reclamation has led not only to the direct loss of salt marshes due to land conversion, but also to vast low-lying coastal areas (often below mean sea level) that are at risk of coastal flooding and provide suitable land to be converted into salt marsh habitat. Most notably, these areas include substantial areas around some of the world's largest estuaries (De Vriend et al., 2011) and deltas (Tessler et al., 2016), where historic coastal wetland losses and current coastal flood risks are highest. The implementation of MRs thereby provides space for truncated salt marshes to extend further inland and occupy a wider elevation range, and, providing sufficient sediment supply, these low-lying, newly inundated wetlands will quickly gain elevation (Liu et al., 2021; Spencer et al., 2012).
In estuaries and deltas, MR may provide flood risk mitigation not only through the deceleration of tidal surges or wind waves over the vegetated salt marsh surface, but also by storing flood water from either the river or the sea (Huguet et al., 2018; Cox et al., 2006). The latter is being referred to as “along-estuary” attenuation (Smolders et al., 2015). For example, in the Scheldt estuary (Belgium and the Netherlands) it has been modelled that a potential loss of its largest salt marsh area (ca. 3000 ha) may increase the maximum water level within the broader estuary by up to 19 cm during storm surges (Smolders et al., 2015). However, the location and size of MRs within estuaries is crucial for their capacity to reduce flood risks; in fact, schemes implemented in the wrong part of the estuary may lead to increases in estuarine water levels during storm surges and a potential loss of other wetland areas due to increased sediment demands (French, 2008; Leuven et al., 2019). Pre-implementation routines therefore usually involve the modelling of the hydrodynamic impacts of MRs on the wider estuarine environment, considering different possible scheme locations, sizes, and designs (Townend and Pethick, 2002; Pontee, 2015).
However, modelling is not routinely conducted for the hydro- and morphodynamic processes within the MR; hence little is known about the so-called “within-marsh” attenuation (Smolders et al., 2015), i.e. the direct reduction in current velocities and water levels during storm surges through the increased surface roughness of shallow vegetated salt marsh surfaces. In contrast to natural salt marshes, MRs are often characterized by one or multiple narrow inlets forming a semi-enclosed tidal basin where hydro- and morphodynamics may differ to those on natural marshes (Kiesel et al., 2020).
Increasingly, MRs are also implemented on open coastlines, where estuarine water level variations are negligible, and increased coastal protection is solely achieved by within-marsh attenuation (Kiesel et al., 2019). Presumably, the lack of meaningful pre-implementation within-marsh modelling is because modelling the geomorphic development of newly inundated salt marshes, e.g. the evolution of tidal creek networks, is challenging and associated with significant uncertainties (Dale et al., 2018). Meanwhile, field and modelling data indicate that the MR size, as well as the nature of the tidal creek networks, may play a deciding role in whether a salt marsh attenuates or amplifies storm surge water levels (Kiesel et al., 2020; Stark et al., 2016). Moreover, this efficiency of an MR salt marsh to attenuate storm surge water levels has been suggested to be reduced for more extreme events, associated with higher inundation depths (Fig. 2) (Hofstede, 2019; Kiesel et al., 2020, 2022).
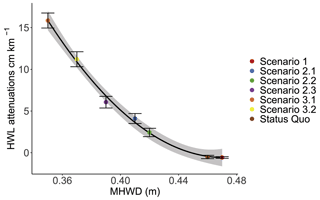
Figure 2Modelled attenuation rates (cm km−1) for the MR Freiston Shore (for equinox spring tides) as a function of the mean high water depth (MHWD) for a series of design scenarios (Kiesel et al., 2020). Status quo: three breaches (ca. 50 m each); scenario 1: complete removal of sea defence; scenario 2: one breach of 45 m (scenario 2.1), 99 m (scenario 2.2), and 30 m (scenario 2.3); scenario 3: extended site area of 1 416 350 m2 instead of 650 067 m2 (scenario 3.1) and 1 124 400 m2 instead of 650 067 m2 (scenario 3.2). Source: Kiesel et al. (2020), with permission from Elsevier.
Both the size of the MR and its biogeomorphic development (including the developing tidal creek network) are controlled by differing designs of MRs (Chirol et al., 2018; Kiesel et al., 2020; Gourgue et al., 2022). Kiesel et al. (2020) suggest that MR designs that reduce the mean high water depth (MHWD) within the site are most efficient in providing tide and storm surge attenuation (Fig. 2). This can be achieved by either increasing the size of the scheme or reducing the number and/or size of breaches of the original sea defence. However, the complete removal of the original defence is likely to create the most natural habitat as it ensures adequate drainage of the restored salt marsh through the formation of tidal channels and increased sediment supply (Hood, 2015; Oosterlee et al., 2020; Gourgue et al., 2022). Problematically, such scheme designs are least effective in reducing coastal flood risks (Fig. 2; Kiesel et al., 2020), suggesting that optimizing the MR's flood mitigation benefits may have trade-offs. Meanwhile, reducing tidal exchange through narrowing tidal breaches and increasing MR size may have undesired impacts on the social acceptability of MR schemes. For example, reduced tidal exchange and uniformly shallow inundation depths are likely to reduce the ecological value of the newly created salt marsh (Pétillon et al., 2010; Mossman et al., 2012), with implications for the aesthetic appearance and the touristic value of the site. To avoid such negative impacts from MR implementation, more recently, tidal exchange within MRs has been reduced by infilling MRs with externally sourced sediment (Dale et al., 2021) while introducing an increased habitat diversity. Meanwhile, larger sites, despite being suggested to be more effective in reducing coastal flood risk, equate to more land being abandoned.
Here, we argue that while more research is needed to optimize the coastal protection function of MRs, implementation of effective MR is also inherently linked to the cultural values and practical interests of local communities. Large MRs can only be implemented with community support (McKinley et al., 2020a), which in turn relies on the proposed scheme to be of cultural and practical value, as well as to be effective in delivering coastal protection (or any other pre-defined ecosystem service). Consequently, effective MR design needs to be adapted to pre-defined targets to ensure restoration success (Wolters et al., 2005; Gourgue et al., 2022).
Perceptions of coastal communities towards the implementation of MRs widely vary within and between schemes and communities (Yamashita, 2021; Goeldner-Gianella, 2007; Myatt-Bell et al., 2002). However, the available peer-reviewed literature on community perception of MRs, and salt marshes more widely, is very sparse. A review by Yamashita (2021) found just nine references relating to public attitudes to MR schemes. Meanwhile, public opposition is considered a key obstacle to MR implementation among practitioners (Esteves and Thomas, 2014), resulting in the delay or abandonment of schemes (Adnitt et al., 2021). For example, at Devereux Farm (Part 2), Essex, UK, the suggested diversion of the coastal footpath associated with the proposed MR implementation raised strong local opposition, which ultimately led to the abandonment of the project (Oliver, 2021). In Donna Nook, North Lincolnshire, UK, a public enquiry delayed the MR implementation by nearly 10 years (Burston, 2018), and in the MR Geltinger Birk, in Schleswig-Holstein, Germany, the public consultation process lasted more than 25 years (Schernewski et al., 2018). Whilst public acceptance is known to practitioners as a major barrier to implementing schemes, there is no comprehensive data in the public domain on where abandoned schemes are and the reasons for public opposition.
From the limited literature on this topic and our experience of working with practitioners, key reasons for public opposition to MR schemes include changes to public access and fear of landscape change (Yamashita et al., 2021), combined with a limited understanding of the benefits of the new intertidal habitats, e.g. for coastal protection (Myatt et al., 2003a, b; Goeldner-Gianella, 2007; McInnes et al., 2021) and other ecosystem services (McKinley et al., 2020b). This is illustrated by our observation that despite coastal managers and scientists arguing for reduced design levels and coastal defence costs behind vegetated wetlands (Burgess-Gamble et al., 2017; MacDonald et al., 2020; van Zelst et al., 2021) most newly established defences on the landward side of MR schemes are at least as high and strong as the original defences to reassure local communities. Nevertheless, MRs are often promoted to coastal communities as coastal protection projects, with co-benefits in habitat creation and carbon sequestration (Burgess-Gamble et al., 2017).
In reality, the primary driver for MR implementation is often to increase natural habitat and biodiversity in relation to upholding environmental policy such as the EU Habitat Directive, attracting significant amounts of private investment (Morris, 2013). Once implemented, MRs are often managed and run by wildlife charities, whose primary interest is the restoration of the marsh's ecological value; hence the post-implementation monitoring is usually focussed on elevation changes and the ecological site development (Mossman et al., 2012; Spencer et al., 2012). Very little research has been conducted on the effectiveness of MR schemes to mitigate coastal flood risks for communities. This knowledge gap has created a sense of uncertainty around the value of MR sites for communities; it is hence not surprising that the trust in MR schemes to mitigate coastal flood risks is low.
Here, we suggest that limited scientific understanding of the flood risk benefits of MRs (Challenge 2) contributes to the lack of community trust in these projects. The knowledge gap and uncertainty around the effectiveness of MR for flood protection offers two opportunities: firstly, to advance scientific understanding of how these projects function, and secondly, to develop methods of engaging communities to genuinely participate in decision-making for NBSs and their design and develop trusting relationships between scientist practitioners and communities.
The notion of involving stakeholders in decision-making to design and implement NBSs stems from wider shifts in the rhetoric, thinking, and practice of natural resource management over the past 3 decades. The participatory paradigm embodies the ideas, values, methods, and behaviours that have emerged to challenge the power dynamics deeply embedded in development throughout the 1950s, 1960s, and 1970s; traditionally, the role of experts and professionals has been to design solutions, while local communities have been framed as “the problem” (Chambers, 1998). Similarly, scientific knowledge is often framed as objective, while stakeholder preferences are viewed as subjective. In reality, scientific knowledge, and the focus of scientific enquiry, is equally subjective and makes assumptions about the preferences of people for how spaces are managed and valued (Owens, 2004). In coastal zones where space is sparse, and risks from natural hazards are high, the integration of scientific and local knowledge benefits the innovation of coastal adaptation solutions (Nursey-Bray et al., 2014). This post-positivist approach accounts for the limited depth of pure scientific knowledge (Foucault, 1970).
Participatory thinking recognizes the power imbalance inherent in these dynamics and reframes “the problem” as one of how professionals engage with communities. In the early 1990s, participatory approaches were envisaged as alternative ways of thinking and acting that shifted the goal of development from designing solutions for communities to designing solutions with communities to achieve empowerment as well as the more pragmatic outcomes of development projects (Park, 1992). In the years that followed, participation has become orthodoxy beyond the sphere of development, including more widely in academic research (Pain and Francis, 2003) and the practice of natural resource management in developed regions, such as engaging local communities for flood risk mitigation (Kelly and Kelly, 2017; Liski et al., 2019).
Early efforts to embrace participation have been heavily critiqued for a multitude of reasons. In brief, “shifts in language have not been accompanied by quite as significant changes in development thinking and practices as they imply” (Cornwall, 2006, p. 78). Two criticisms that are echoed strongly in natural resource management and research relate to the nature and degree of participation. Firstly, Pimbert (2004) distinguishes between engagement in rhetoric only compared to engagement that involves transformation, where transformation refers to multidirectional learning with a genuine capacity for change. Research projects that engage stakeholders in some form of participation often fail to move beyond rhetoric. Secondly, Bergold and Thomas (2012) highlight challenges associated with the degree of participation, including the point along the research continuum that stakeholders are engaged. Participation is usually relegated to a later stage of research once the serious decisions about defining problems and setting model parameters have been made by professionals and experts; often stakeholders are invited to select from pre-defined solutions rather than contributing to scenario building. Thus, mismanaged participation can risk reinforcing or recreating existing inequalities within new institutional frameworks that only partially fulfil the participatory orthodoxy.
An alternative to traditional top-down methods of community engagement is to begin the participatory process early in project design, allowing community values and beliefs to inform the building of management scenarios (i.e. scenario building). This approach, known as “knowledge co-production”, has been adopted for climate change adaptation (Singh et al., 2021), developing “sustainable future” scenarios (Iwaniec et al., 2020), and flood hazard mapping (Luke et al., 2018) among other applications. In the context of flood risk management, co-production is usually limited to agenda setting and evaluation (Mees et al., 2018). In part, the capacity for genuine knowledge co-production, such as developing scenarios for MR, depends on the accuracy of scientific knowledge as well as appropriately timed engagement. Ideally, a bottom-up approach would engage communities in every phase of designing and implementing a flood mitigation project.
In the case of MR, investigating the potential use of projects for coastal protection and flood mitigation will necessarily involve both improving scientific understanding of biophysical processes and developing effective and meaningful community engagement. Best-practice knowledge co-production offers a way forward.
Here, we propose a co-design process for developing MR schemes to optimize project success through scientific knowledge co-production, following the principles outlined by Norström et al. (2020), who propose efficient knowledge co-production to be (1) context-based, (2) pluralistic, (3) goal-oriented, and (4) interactive. For MR schemes, this involves engaging communities and other stakeholders early enough in the project development to contribute meaningfully to the negotiation of goals and objectives and the scenario building, as well as in later planning stages. This includes iterations of selecting preferred scenarios for implementation and assessing project effectiveness over different timescales (short, medium, and long).
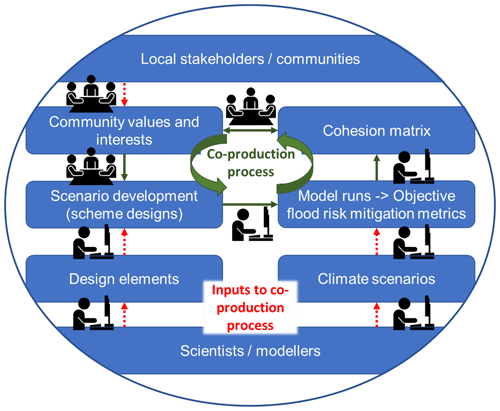
Figure 3Schematic representation of the proposed co-production process to plan and develop managed realignment schemes. Some workflows are completed through traditional academic knowledge production (researcher working on computer), whereas other workflows will be completed through collaboration with stakeholders, communities, and researchers (three people sitting around a table). The core workflow of the co-production process is indicated by full green linkages, while input into the co-production process is indicated by dashed red linkages.
Coastal management, and natural resource management more generally, is increasingly relying on user-pays approaches to establish and maintain infrastructure, practices, and projects that are perceived to serve the public good (Kauffman, 2015). The success of these projects can be undermined if local communities are unwilling to support schemes. Projects are more likely to succeed if stakeholder preferences are incorporated into project development in a way that promotes agency. Hence, facilitating a positive experience of engagement and allowing meaningful stakeholder–expert relationships to develop is as vital to the success of co-production as involving stakeholders in appropriate phases of decision-making. Tompkins et al. (2008) also highlight the need to incorporate stakeholder preferences into climate change planning, as well as to ensure stakeholders understand the necessary trade-offs involved in any coastal management decision.
Here, we propose using a scenario-building stakeholder model to engage communities in each phase of project design alongside experts and researchers. Scenario building through community engagement has previously been adopted for coastal management in the context of governance and selecting decision-making approaches for climate change mitigation (Tompkins et al., 2008). Our approach is designed to help address the apparent discrepancy between the discursive democratic stakeholder participation that is often pursued, and considered crucially important by most coastal management decision-makers, and the liberal constitutional approach that is usually implemented on the ground (Few et al., 2007; Blunkell, 2017). Local conflict around MR scheme design is widespread and may significantly delay project implementation (Oliver, 2021). This is not only because MR implementation could directly affect local communities, e.g. through reduced access to coastal land, but also because the multiple co-benefits of MR schemes are poorly quantified (McInnes et al., 2021). Co-designing coastal adaptation strategies between community stakeholders and researchers has previously proven useful to raise the value of numerical flood modelling and optimize adaptation solutions and to enhance the local community's awareness of local flood risks and the potential need for future action (Pasquier et al., 2020). Iwaniec et al. (2020) further argue that co-produced future scenarios may significantly enhance sustainability of the developed management solutions.
We envisage six steps for effective co-production to optimize the success of MR sites (Fig. 3):
-
Establish links to relevant stakeholder and community groups in proximity of a potential MR scheme. This first step involves identifying relevant stakeholders and community groups and getting to know them personally. Rather than having a pre-determined list of stakeholders and community groups, these should be identified through individual discussion with initial and further contacts, e.g. as outlined by Reed et al. (2009).
-
Define with community and stakeholder groups what a “successful” MR project would deliver. This gives communities a voice in determining the definition of success, which means that the subsequent production of scientific evidence might differ from that sought by a traditional positivist approach (Tompkins et al., 2008). The definition of success is expected to vary considerably between different communities due to geographic location, socio-economic and demographic structure, experience with past coastal flooding, and previous experiences with natural resource management projects (Rupp-Armstrong and Nicholls, 2007).
-
Develop possible scenarios to be considered. For the informed scenario development, researchers will provide the “building blocks”, or design elements, that may be used to develop MRs. Examples of such design elements include the nature of the breach in the sea defence (completely removed seaward dike, open breach, or tidal exchange via a sluice); the size and number of breaches (which could determine if it was possible to build bridges across them); the number, size, and structure of the drainage network (potentially determining the nature of any possible walking paths within the site and maintaining current access routes around the site); and the use of landscaping techniques, such as sediment infilling (allowing for the creation of a more bio-diverse area). Further “building blocks” may be added during the co-production process as the involved stakeholders should not be limited by any pre-defined set of available building blocks (Pasquier et al., 2020).
-
The co-produced MR designs are used to model coastal flood risk reductions for selected climate scenarios. Objective metrics on flood risk mitigation, such as ratio of water levels within and outside MRs, overtopping, tidal prism, and seiche formation (Christie et al., 2018), are used to evaluate the flood mitigation value of each co-designed MR scheme. Some of the MR designs may prove inefficient in reducing coastal flood risks or in extreme cases may even exacerbate them, whilst others may prove to be more efficient (Iwaniec et al., 2020). An MR design where the original sea defence is mostly removed to create natural habitat of high ecological value, for example, may be less efficient in mitigating coastal flood risks than an MR design with a strongly reduced water exchange and a potentially lower ecological value (Mossman et al., 2012; Kiesel et al., 2020).
-
By evaluating the objective flood risk mitigation metrics for different scheme designs (satisfying different community values and interests), so-called cohesion matrices are developed to map the compatibility of various community values and interests with the objective to mitigate coastal flood risks. Testing different co-produced scheme designs against the coastal protection function, now and in the long term, will inform stakeholders and communities about possible benefits and limitations of any one scheme design and provide novel scientific insights into the flood mitigation function of MRs.
-
Considering the outcome of the produced cohesion matrix, community values and interests may (or may not) shift in priorities, allowing for the potential development of alternative designs, which in turn are modelled and evaluated until a consensus for the scheme design is reached. The proposed co-production process thereby provides a tool for quantitatively evaluating the benefits of MRs with regards to flood mitigation and for contrasting those to the specific interests of the local community, which often significantly differ from those of coastal planners and researchers (McInnes et al., 2021).
Despite significant political ambitions to implement NBSs and MR for reducing coastal flood risks in the coastal zone, now and under future climate change and SLR scenarios, significant knowledge gaps with regards to the efficiency of MR schemes for coastal flood mitigation remain. This is surprising as the MR efficiency in mitigating coastal flood risks often constitutes (one of) the key argument(s) of scientists and coastal managers to convince local stakeholders and communities to give up their land. However, local support for MR implementation is often lacking as stakeholders and communities lose access to (valuable) land, and trust in the coastal protection function of MRs is low. This is becoming increasingly important, not least because the little available evidence there is suggests that only larger MR schemes may contribute to flood risk mitigation through attenuation of storm surge heights, whereas smaller schemes do not. We therefore argue that new approaches of stakeholder and community engagement are needed and that involving stakeholders and communities in the knowledge production process allows for the participating individuals to design a scheme that suits their purpose and is efficient in doing what it is supposed to do.
All data used for producing this paper, and most notably Fig. 1, are publicly and freely available on the OMReg database (ABPmer, 2021: https://www.omreg.net, last access: 17 November 2021).
MS, EKC and JK developed some of the early conceptual ideas around the flood mitigation potentials of managed realignments. MS brought together the author team and structured the initial draft. MS, HLM, HEM and EKC contributed to the writing and proofreading of the original draft, and MS, HLM and HEM contributed to the revision of the manuscript.
The contact author has declared that none of the authors has any competing interests.
Publisher's note: Copernicus Publications remains neutral with regard to jurisdictional claims in published maps and institutional affiliations.
The author team would like to thank the REST-COAST and ECAS-BALTIC project partners for providing inspiration for this perspectives piece and two anonymous reviewers for their constructive reviews and helpful comments.
This research has been supported by the European Union's Horizon 2020 research and innovation programme under grant agreement no. 101037097 (REST-COAST project) and by the German Bundesministerium für Bildung und Forschung (BMBF) under grant agreement no. 03F0860H (ECAS-BALTIC project).
This paper was edited by Sven Fuchs and reviewed by two anonymous referees.
ABPmer: OMReg – A database of completed coastal habitat creation schemes and other adaptation projects, https://www.omreg.net/, last access: 17 November 2021.
Adnitt, C., Vural, M., Yamashita, H., and Preston, J.: Legislation, in: Saltmarsh Restoration Handbook – UK & Ireland, edited by: Hudson, R., Kenworthy, J., and Best, M., Environment Agency, Bristol, UK, 37–49, https://catchmentbasedapproach.org/learn/saltmarsh-restoration-handbook (last access: 23 February 2022), 2021.
Bergold, J. and Thomas, S.: Participatory Research Methods: A Methodological Approach in Motion, Hist. Soc. Res., 37, 191–222, https://doi.org/10.17169/fqs-13.1.1801, 2012.
Blunkell, C. T.: Local participation in coastal adaptation decisions in the UK: between promise and reality, Local Environ., 22, 492–507, https://doi.org/10.1080/13549839.2016.1233525, 2017.
Burgess-Gamble, L., Ngai, R., Wilkinson, M., Nisbet, T., Pontee, N., Harvey, R., Kipling, K., Addy, S., Rose, S., Maslen, S., Jay, H., Nicholson, A., Page, T., Jonczyk, J., and Quinn, P.: Working with Natural Processes – Evidence Directory, Environment Agency, Bristol, UK, https://www.gov.uk/flood-and-coastal-erosion-risk-management-research-reports/working-with-natural-processes-to-reduce-flood-risk#reports (last access: 30 November 2021), 2017.
Burston, J.: Report to the Secretary of State for Transport, Report DPI/D2510/18/9, The Planning Inspectorate, https://www.kingschambers.com/assets/files/Donna Nook Inspector's Report.pdf (last access: 10 February 2022), 2018.
Chambers, R.: Forward, in: Who changes? Institutionalizing participation in development, edited by: Blackburn, J. and Holland, J., Intermediate Technology, London, UK, ISBN 978-1853394201, 1998.
Chirol, C., Haigh, I. D., Pontee, N., Thompson, C. E., and Gallop, S. L.: Parametrizing tidal creek morphology in mature saltmarshes using semi-automated extraction from lidar, Proc. SPIE, 209, 291–311, https://doi.org/10.1016/j.rse.2017.11.012, 2018.
Christie, E., Möller, I., Spencer, T., and Yates, M.: Modeling wave attenuation due to saltmarsh vegetation using a modified SWAN model, Coast. Eng. Proc., 1, 73, https://doi.org/10.9753/icce.v36.papers.73, 2018.
Coleman, D. J., Schuerch, M., Temmerman, S., Guntenspergen, G., Smith, C. G. and Kirwan, M. L.: Reconciling models and measurements of marsh vulnerability to sea level rise, Limnol. Oceanogr. Lett., 7, 140–149, https://doi.org/10.1002/lol2.10230, 2022.
Cornwall, A.: Historical perspectives on participation in development, Commonw. Comp. Polit., 44, 62–83, https://doi.org/10.1080/14662040600624460, 2006.
Cox, T., Maris, T., De Vleeschauwer, P., De Mulder, T., Soetaert, K., and Meire, P.: Flood control areas as an opportunity to restore estuarine habitat, Ecol. Eng., 28, 55–63, https://doi.org/10.1016/j.ecoleng.2006.04.001, 2006.
Dale J., Burgess, H. M., Burnside, N. G., Kilkie, P., Nash, D. J., and Cundy, A. B.: The evolution of embryonic creek systems in a recently inundated large open coast managed realignment site, Anthropocene Coasts, 1, 16–33, https://doi.org/10.1139/anc-2017-0005, 2018.
Dale, J., Burgess, H. M., Berg, M. J., Strong, C. J., and Burnside, N. G.: Morphological evolution of a non-engineered managed realignment site following tidal inundation, Estuar. Coast. Shelf. S., 260, 107510, https://doi.org/10.1016/j.ecss.2021.107510, 2021.
De Vriend, H. J., Wang, Z. B., Ysebaert, T., Herman, P. M. J., and Ding, P.: Eco-Morphological Problems in the Yangtze Estuary and the Western Scheldt, Wetlands, 31, 1033–1042, https://doi.org/10.1007/s13157-011-0239-7, 2011.
Doody, J. P.: Coastal squeeze and managed realignment in southeast England, does it tell us anything about the future?, Ocean Coast. Manage., 79, 34–41, https://doi.org/10.1016/j.ocecoaman.2012.05.008, 2013.
Esteves, L. S.: Managed Realignment: A Viable Long-Term Coastal Management Strategy?, Springer, Dordrecht, ISBN 978-9401790284, 2014.
Esteves, L. S. and Thomas, K.: Managed realignment in practice in the UK: results from two independent surveys, J. Coast. R., 70, 407–413, https://doi.org/10.2112/si70-069.1, 2014.
Fagherazzi, S. and Wiberg, P. L.: Importance of wind conditions, fetch, and water levels on wave-generated shear stresses in shallow intertidal basins, J. Geophys. Res.-Earth, 114, F03022, https://doi.org/10.1029/2008JF001139, 2009.
Fairchild, T. P., Bennett, W. G., Smith, G., Day, B., Skov, M. W., Möller, I., Beaumont, N., Karunarathna, H., and Griffin, J. N.: Coastal wetlands mitigate storm flooding and associated costs in estuaries, Environ. Res. Lett., 16, 074034, https://doi.org/10.1088/1748-9326/ac0c45, 2021.
Few, R., Brown, K., and Tompkins, E. L.: Public participation and climate change adaptation: avoiding the illusion of inclusion, Clim. Policy, 7, 46–59, https://doi.org/10.1080/14693062.2007.9685637, 2007.
Fischbach, J. R., Johnson, D. R., and Groves, D. G.: Flood damage reduction benefits and costs in Louisiana's 2017 Coastal Master Plan, Environ. Res. Commun., 1, 111001, https://doi.org/10.1088/2515-7620/ab4b25, 2019.
Foucault, M.: The Order of Things – An archaeology of the human sciences, Travistock Publications, London, UK, ISBN 978-0415267373, 1970.
Fox-Kemper, B., Hewitt, H. T., Xiao, C., Aðalgeirsdóttir, G., Drijfhout, S. S., Edwards, T. L., Golledge, N. R., Hemer, M., Kopp, R. E., Krinner, G., Mix, A., Notz, D., Nowicki, S., Nurhati, I. S., Ruiz, L., Sallée, J.-B., Slangen, A. B. A., and Yu, Y.: Ocean, Cryosphere and Sea Level Change, in: Climate Change 2021: The Physical Science Basis, Contribution of Working Group I to the Sixth Assessment Report of the Intergovernmental Panel on Climate Change, edited by: Masson-Delmotte, V., Zhai, P., Pirani, A., Connors, S. L., Péan, C., Berger, S., Caud, N., Chen, Y., Goldfarb, L., Gomis, M. I., Huang, M., Leitzell, K., E., L., Matthews, J. B. R., Maycock, T. K., Waterfield, T., Yelekçi, O., Yu, R., and Zhou, B., Cambridge University Press, Cambridge, UK and New York, NY, USA, 1211–1362, https://doi.org/10.1017/9781009157896.011, 2021.
French, J. R.: Hydrodynamic Modelling of Estuarine Flood Defence Realignment as an Adaptive Management Response to Sea-Level Rise, J. Coast. R., 24, 1–12, https://doi.org/10.2112/05-0534.1, 2008.
Gedan, K. B., Silliman, B. R., and Bertness, M. D.: Centuries of Human-Driven Change in Salt Marsh Ecosystems, Annu. Rev. Mar. Sci., 1, 117–141, https://doi.org/10.1146/annurev.marine.010908.163930, 2009.
Goeldner-Gianella, L.: Perceptions and Attitudes Toward De-polderisation in Europe: A Comparison of Five Opinion Surveys in France and the UK, J. Coast. R., 23, 1218–1230, https://doi.org/10.2112/04-0416R.1, 2007.
Gourgue, O., van Belzen, J., Schwarz, C., Vandenbruwaene, W., Vanlede, J., Belliard, J. P., Fagherazzi, S., Bouma, T. J., van de Koppel, J., and Temmerman, S.: Biogeomorphic modeling to assess the resilience of tidal-marsh restoration to sea level rise and sediment supply, Earth Surf. Dynam., 10, 531–553, https://doi.org/10.5194/esurf-10-531-2022, 2022.
Hinkel, J., Lincke, D., Vafeidis, A. T., Perrette, M., Nicholls, R. J., Tol, R. S. J., Marzeion, B., Fettweis, X., Ionescu, C., and Levermann, A.: Coastal flood damage and adaptation costs under 21st century sea-level rise, P. Natl. Acad. Sci. USA, 111, 3292–3297, https://doi.org/10.1073/pnas.1222469111, 2014.
Hofstede, J. L. A.: On the feasibility of managed retreat in the Wadden Sea of Schleswig-Holstein, J. Coast. Conserv., 23, 1069–1079, https://doi.org/10.1007/s11852-019-00714-x, 2019.
Hood, W. G.: Predicting the number, orientation and spacing of dike breaches for tidal marsh restoration, Ecol. Eng., 83, 319–327, https://doi.org/10.1016/j.ecoleng.2015.07.002, 2015.
Huguet, J.-R., Bertin, X., and Arnaud, G.: Managed realignment to mitigate storm-induced flooding: A case study in La Faute-sur-mer, France, Coast. Eng., 134, 168–176, https://doi.org/10.1016/j.coastaleng.2017.08.010, 2018.
Iwaniec, D. M., Cook, E. M., Davidson, M. J., Berbés-Blázquez, M., Georgescu, M., Krayenhoff, E. S., Middel, A., Sampson, D. A., and Grimm, N. B.: The co-production of sustainable future scenarios, Landsc. Urban Plan., 197, 103744, https://doi.org/10.1016/j.landurbplan.2020.103744, 2020.
Kauffman, G. J.: Governance, Policy, and Economics of Intergovernmental River Basin Management, Water Resour. Manage., 29, 5689–5712, https://doi.org/10.1007/s11269-015-1141-5, 2015.
Kelly, R. and Kelly, U.: Community engagement on climate adaptation – an evidence review, Environment Agency, Bristol, UK, ISBN 978-1849114295, 2017.
Kiesel, J., Schuerch, M., Möller, I., Spencer, T., and Vafeidis, A.: Attenuation of high water levels over restored saltmarshes can be limited. Insights from Freiston Shore, Lincolnshire, UK, Ecol. Eng., 136, 89–100, https://doi.org/10.1016/j.ecoleng.2019.06.009, 2019.
Kiesel, J., Schuerch, M., Christie, E. K., Möller, I., Spencer, T., and Vafeidis, A. T.: Effective design of managed realignment schemes can reduce coastal flood risks, Estuar. Coast. Shelf. Sci., 242, 106844, https://doi.org/10.1016/j.ecss.2020.106844, 2020.
Kiesel, J., MacPherson, L. R., Schuerch, M., and Vafeidis, A. T.: Can Managed Realignment Buffer Extreme Surges? The Relationship Between Marsh Width, Vegetation Cover and Surge Attenuation, Estuar. Coast., 45, 345–362, https://doi.org/10.1007/s12237-021-00984-5, 2022.
Kirwan, M. L., Temmerman, S., Skeehan, E. E., Guntenspergen, G. R., and Fagherazzi, S.: Overestimation of marsh vulnerability to sea level rise, Nat. Clim. Change, 6, 253–260, https://doi.org/10.1038/nclimate2909, 2016.
Leuven, J. R. F. W., Pierik, H. J., Vegt, M. v. d., Bouma, T. J., and Kleinhans, M. G.: Sea-level-rise-induced threats depend on the size of tide-influenced estuaries worldwide, Nat. Clim. Change, 9, 986–992, https://doi.org/10.1038/s41558-019-0608-4, 2019.
Liski, A. H., Ambros, P., Metzger, M. J., Nicholas, K. A., Wilson, A. M. W., and Krause, T.: Governance and stakeholder perspectives of managed re-alignment: adapting to sea level rise in the Inner Forth estuary, Scotland, Reg. Environ. Change, 19, 2231–2243, https://doi.org/10.1007/s10113-019-01505-8, 2019.
Liu, Z., Fagherazzi, S., and Cui, B.: Success of coastal wetlands restoration is driven by sediment availability, Commun. Earth Environ., 2, 44, https://doi.org/10.1038/s43247-021-00117-7, 2021.
Loder, N. M., Irish, J. L., Cialone, M. A., and Wamsley, T. V.: Sensitivity of hurricane surge to morphological parameters of coastal wetlands, Estuar. Coast. Shelf Sci., 84, 625–636, https://doi.org/10.1016/j.ecss.2009.07.036, 2009.
Lotze, H. K., Lenihan, H. S., Bourque, B. J., Bradbury, R. H., Cooke, R. G., Kay, M. C., Kidwell, S. M., Kirby, M. X., Peterson, C. H., and Jackson, J. B. C.: Depletion, Degradation, and Recovery Potential of Estuaries and Coastal Seas, Science, 312, 1806–1809, https://doi.org/10.1126/science.1128035, 2006.
Luke, A., Sanders, B. F., Goodrich, K. A., Feldman, D. L., Boudreau, D., Eguiarte, A., Serrano, K., Reyes, A., Schubert, J. E., AghaKouchak, A., Basolo, V., and Matthew, R. A.: Going beyond the flood insurance rate map: insights from flood hazard map co-production, Nat. Hazards Earth Syst. Sci., 18, 1097–1120, https://doi.org/10.5194/nhess-18-1097-2018, 2018.
MacDonald, M. A., de Ruyck, C., Field, R. H., Bedford, A., and Bradbury, R. B.: Benefits of coastal managed realignment for society: Evidence from ecosystem service assessments in two UK regions, Estuar. Coast. Shelf. Sci., 244, 105609, https://doi.org/10.1016/j.ecss.2017.09.007, 2020.
McInnes, R. J., Everard, M., and Yamashita, H.: People's perceptions towards the Steart Marshes Creation Project through stakeholder interviews and questionnaires, in: Coastal Wetlands Restoration, Routledge, New York, USA, 46–60, ISBN 978-036786309, 2021.
McKinley, E., Pagès, J. F., Alexander, M., Burdon, D., and Martino, S. Uses and management of saltmarshes: A global survey. Estuar. Coast. Shelf Sci., 243, 106840, https://doi.org/10.1016/j.ecss.2020.106840, 2020a.
McKinley, E., Pagès, J. F., Ballinger, R. C., and Beaumont, N. Forgotten landscapes: Public attitudes and perceptions of coastal saltmarshes, Ocean Coast. Manage., 187, 105117, https://doi.org/10.1016/j.ocecoaman.2020.105117, 2020b.
Mees, H., Alexander, M., Gralepois, M., Matczak, P., and Mees, H.: Typologies of citizen co-production in flood risk governance, Envion. Sci. Poliy, 89, 330–339, https://doi.org/10.1016/j.envsci.2018.08.011, 2018.
Möller, I.: Quantifying saltmarsh vegetation and its effect on wave height dissipation: Results from a UK East coast saltmarsh, Estuar. Coast. Shelf Sci., 69, 337–351, https://doi.org/10.1016/j.ecss.2006.05.003, 2006.
Möller, I.: Applying Uncertain Science to Nature-Based Coastal Protection: Lessons From Shallow Wetland-Dominated Shores, Front. Environ. Sci., 7, 49, https://doi.org/10.3389/fenvs.2019.00049, 2019.
Möller, I., Kudella, M., Rupprecht, F., Spencer, T., Paul, M., van Wesenbeeck, B. K., Wolters, G., Jensen, K., Bouma, T. J., Miranda-Lange, M., and Schimmels, S.: Wave attenuation over coastal salt marshes under storm surge conditions, Nat. Geosci., 7, 727–731, https://doi.org/10.1038/ngeo2251, 2014.
Morris, R. K. A.: Managed realignment as a tool for compensatory habitat creation – A re-appraisal, Ocean Coast. Manage., 73, 82–91, https://doi.org/10.1016/j.ocecoaman.2012.12.013, 2013.
Mossman, H. L., Davy, A. J., and Grant, A.: Does managed coastal realignment create saltmarshes with `equivalent biological characteristics' to natural reference sites?, J. Appl. Ecol., 49, 1446–1456, https://doi.org/10.1111/j.1365-2664.2012.02198.x, 2012.
Myatt, L. B., Scrimshaw, M. D., and Lester, J. N.: Public perceptions and attitudes towards a forthcoming managed realignment scheme: Freiston Shore, Lincolnshire, UK, Ocean Coast. Manage., 46, 565–582, https://doi.org/10.1016/S0964-5691(03)00035-8, 2003a.
Myatt, L. B., Scrimshaw, M. D., and Lester, J. N.: Public Perceptions and Attitudes Towards a Current Managed Realignment Scheme: Brancaster West Marsh, North Norfolk, U.K., J. Coast. Res., 19, 278–286, 2003b.
Myatt-Bell, L. B., Scrimshaw, M. D., Lester, J. N., and Potts, J. S.: Public perception of managed realignment: Brancaster West Marsh, North Norfolk, UK, Mar. Policy, 26, 45–57, https://doi.org/10.1016/S0308-597X(01)00033-1, 2002.
Norström, A. V., Cvitanovic, C., Löf, M. F., West, S., Wyborn, C., Balvanera, P., Bednarek, A. T., Bennett, E. M., Biggs, R., de Bremond, A., Campbell, B. M., Canadell, J. G., Carpenter, S. R., Folke, C., Fulton, E. A., Gaffney, O., Gelcich, S., Jouffray, J.-B., Leach, M., Le Tissier, M., Martín-López, B., Louder, E., Loutre, M.-F., Meadow, A. M., Nagendra, H., Payne, D., Peterson, G. D., Reyers, B., Scholes, R., Speranza, C. I., Spierenburg, M., Stafford-Smith, M., Tengö, M., van der Hel, S., van Putten, I., and Österblom, H.: Principles for knowledge co-production in sustainability research, Nat. Sustain., 3, 182–190, https://doi.org/10.1038/s41893-019-0448-2, 2020.
Nursey-Bray, M. J., Vince, J., Scott, M., Haward, M., O'Toole, K., Smith, T., Harvey, N., and Clarke, B.: Science into policy? Discourse, coastal management and knowledge, Environ. Sci. Policy, 38, 107–119, https://doi.org/10.1016/j.envsci.2013.10.010, 2014.
Oliver, S.: Land Abandoned to the Sea: The Managed Realignment of Coastal Area, Bloomsbury Publishing, London, UK, ISBN 978-1788310857, 2021.
Oosterlee, L., Cox, T. J. S., Temmerman, S., and Meire, P.: Effects of tidal re-introduction design on sedimentation rates in previously embanked tidal marshes, Estuar. Coast. Shelf Sci., 244, 106428, https://doi.org/10.1016/j.ecss.2019.106428, 2020.
Owens, S.: Siting, sustainable development and social priorities, J. Risk Res., 7, 101–114, https://doi.org/10.1080/1366987042000158686, 2004.
Pain, R. and Francis, P.: Reflections on Participatory Research, Area, 35, 46–54, https://doi.org/10.1111/1475-4762.00109, 2003.
Park, P.: The discovery of participatory research as a new scientific paradigm: Personal and intellectual accounts, Am. Sociol., 23, 29–42, https://doi.org/10.1007/BF02691929, 1992.
Paquier, A.-E., Haddad, J., Lawler, S., and Ferreira, C. M.: Quantification of the Attenuation of Storm Surge Components by a Coastal Wetland of the US Mid Atlantic, Estuar. Coasts, 40, 930–946, https://doi.org/10.1007/s12237-016-0190-1, 2017.
Pasquier, U., Few, R., Goulden, M. C., Hooton, S., He, Y., and Hiscock, K. M.: “We can't do it on our own!” – Integrating stakeholder and scientific knowledge of future flood risk to inform climate change adaptation planning in a coastal region, Environ. Sci. Policy, 103, 50–57, https://doi.org/10.1016/j.envsci.2019.10.016, 2020.
Pétillon, J., Erfanzadeh, R., Garbutt, A., Maelfait, J.-P., and Hoffmann, M.: Inundation Frequency Determines the Post-Pioneer Successional Pathway in a Newly Created Salt Marsh, Wetlands, 30, 1097–1105, https://doi.org/10.1007/s13157-010-0115-x, 2010.
Pimbert, M.: Natural resources, people and participation, in: Participatory Learning and Action 50: Critical reflections, future directions, edited by: Chambers, R., Kenton, N., and Ashley, H., Institute for Environment and Development, 131–139, https://pubs.iied.org/sites/default/files/pdfs/migrate/9440IIED.pdf (last access: 9 July 2022), 2004.
Pollard, J., Spencer, T., and Brooks, S.: The interactive relationship between coastal erosion and flood risk, Prog. Phys. Geogr., 43, 574–585, https://doi.org/10.1177/0309133318794498, 2019.
Pontee, N.: Defining coastal squeeze: A discussion, Ocean Coast. Manage., 84, 204–207, https://doi.org/10.1016/j.ocecoaman.2013.07.010, 2013.
Pontee, N. I.: Impact of managed realignment design on estuarine water levels, P. I. Civ. Eng.-Mar. En., 168, 48–61, https://doi.org/10.1680/jmaen.13.00016, 2015.
Reed, D., van Wesenbeeck, B., Herman, P. M. J., and Meselhe, E.: Tidal flat-wetland systems as flood defenses: Understanding biogeomorphic controls, Estuar. Coast. Shelf Sci., 213, 269–282, https://doi.org/10.1016/j.ecss.2018.08.017, 2018.
Reed, M. S., Graves, A., Dandy, N., Posthumus, H., Hubacek, K., Morris, J., Prell, C., Quinn, C. H., and Stringer, L. C.: Who's in and why? A typology of stakeholder analysis methods for natural resource management, J. Environ. Manage., 90, 1933–1949, https://doi.org/10.1016/j.jenvman.2009.01.001, 2009.
Resio, D. T. and Westerink, J. J.: Modeling the physics of storm surges, Phys. Today, 61, 33–38, https://doi.org/10.1063/1.2982120, 2008.
Roca, E. and Villares, M.: Public perceptions of managed realignment strategies: The case study of the Ebro Delta in the Mediterranean basin, Ocean Coast. Manage., 60, 38–47, https://doi.org/10.1016/j.ocecoaman.2012.01.002, 2012.
Rupp-Armstrong, S. and Nicholls, R. J.: Coastal and Estuarine Retreat: A Comparison of the Application of Managed Realignment in England and Germany, J. Coast. R., 23, 1418–1430, https://doi.org/10.2112/04-0426.1, 2007.
Schernewski, G., Bartel, C., Kobarg, N., and Karnauskaite, D.: Retrospective assessment of a managed coastal realignment and lagoon restoration measure: the Geltinger Birk, Germany, J. Coast. Conserv., 22, 157–167, https://doi.org/10.1007/s11852-017-0496-6, 2018.
Schuerch, M., Spencer, T., Temmerman, S., Kirwan, M. L., Wolff, C., Lincke, D., McOwen, C. J., Pickering, M. D., Reef, R., Vafeidis, A. T., Hinkel, J., Nicholls, R. J., and Brown, S.: Future response of global coastal wetlands to sea-level rise, Nature, 561, 231–234, https://doi.org/10.1038/s41586-018-0476-5, 2018.
Shepard, C. C., Crain, C. M., and Beck, M. W.: The Protective Role of Coastal Marshes: A Systematic Review and Meta-analysis, PLOS ONE, 6, e27374, https://doi.org/10.1371/journal.pone.0027374, 2011.
Singh, C., Iyer, S., New, M. G., Few, R., Kuchimanchi, B., Segnon, A. C., and Morchain, D.: Interrogating `effectiveness' in climate change adaptation: 11 guiding principles for adaptation research and practice, Clim. Dev., https://doi.org/10.1080/17565529.2021.1964937, in press, 2021.
Smolders, S., Plancke, Y., Ides, S., Meire, P., and Temmerman, S.: Role of intertidal wetlands for tidal and storm tide attenuation along a confined estuary: a model study, Nat. Hazards Earth Syst. Sci., 15, 1659–1675, https://doi.org/10.5194/nhess-15-1659-2015, 2015.
Spencer, T., Friess, D. A., Möller, I., Brown, S. L., Garbutt, R. A., and French, J. R.: Surface elevation change in natural and re-created intertidal habitats, eastern England, UK, with particular reference to Freiston Shore, Wetl. Ecol. Manage., 20, 9–33, https://doi.org/10.1007/s11273-011-9238-y, 2012.
Stark, J., Van Oyen, T., Meire, P., and Temmerman, S.: Observations of tidal and storm surge attenuation in a large tidal marsh, Limnol. Oceanogr., 60, 1371–1381, https://doi.org/10.1002/lno.10104, 2015.
Stark, J., Plancke, Y., Ides, S., Meire, P., and Temmerman, S.: Coastal flood protection by a combined nature-based and engineering approach: Modeling the effects of marsh geometry and surrounding dikes, Estuar. Coast. Shelf Sci., 175, 34–45, https://doi.org/10.1016/j.ecss.2016.03.027, 2016.
Temmerman, S. and Kirwan, M. L.: Building land with a rising sea, Science, 349, 588–589, https://doi.org/10.1126/science.aac8312, 2015.
Temmerman, S., Meire, P., Bouma, T. J., Herman, P. M. J., Ysebaert, T., and De Vriend, H. J.: Ecosystem-based coastal defence in the face of global change, Nature, 504, 79–83, https://doi.org/10.1038/nature12859, 2013.
Tessler, Z. D., Vörösmarty, C. J., Grossberg, M., Gladkova, I., and Aizenman, H.: A global empirical typology of anthropogenic drivers of environmental change in deltas, Sustain. Sci., 11, 525–537, https://doi.org/10.1007/s11625-016-0357-5, 2016.
Tompkins, E. L., Few, R., and Brown, K.: Scenario-based stakeholder engagement: Incorporating stakeholders preferences into coastal planning for climate change, J. Environ. Manage., 88, 1580–1592, https://doi.org/10.1016/j.jenvman.2007.07.025, 2008.
Townend, I. and Pethick, J.: Estuarine flooding and managed retreat, Philos. T. Roy. Soc. A, 360, 1477–1495, https://doi.org/10.1098/rsta.2002.1011, 2002.
Vafeidis, A. T., Schuerch, M., Wolff, C., Spencer, T., Merkens, J. L., Hinkel, J., Lincke, D., Brown, S., and Nicholls, R. J.: Water-level attenuation in global-scale assessments of exposure to coastal flooding: a sensitivity analysis, Nat. Hazards Earth Syst. Sci., 19, 973–984, https://doi.org/10.5194/nhess-19-973-2019, 2019.
van Zelst, V. T. M., Dijkstra, J. T., van Wesenbeeck, B. K., Eilander, D., Morris, E. P., Winsemius, H. C., Ward, P. J., and de Vries, M. B.: Cutting the costs of coastal protection by integrating vegetation in flood defences, Nat. Commun., 12, 6533, https://doi.org/10.1038/s41467-021-26887-4, 2021.
Vousdoukas, M. I., Ranasinghe, R., Mentaschi, L., Plomaritis, T. A., Athanasiou, P., Luijendijk, A., and Feyen, L.: Sandy coastlines under threat of erosion, Nat. Clim. Change, 10, 260–263, https://doi.org/10.1038/s41558-020-0697-0, 2020.
Wamsley, T. V., Cialone, M. A., Smith, J. M., Ebersole, B. A., and Grzegorzewski, A. S.: Influence of landscape restoration and degradation on storm surge and waves in southern Louisiana, Nat. Hazards, 51, 207–224, https://doi.org/10.1007/s11069-009-9378-z, 2009.
Wamsley, T. V., Cialone, M. A., Smith, J. M., Atkinson, J. H., and Rosati, J. D.: The potential of wetlands in reducing storm surge, Ocean Eng., 37, 59–68, https://doi.org/10.1016/j.oceaneng.2009.07.018, 2010.
Wolters, M., Garbutt, A., and Bakker, J. P.: Salt-marsh restoration: evaluating the success of de-embankments in north-west Europe, Biol. Conserv., 123, 249–268, https://doi.org/10.1016/j.biocon.2004.11.013, 2005.
Yamashita, H.: Studying social perceptions of risks and benefits of coastal wetland restorations: Its importance and complexities, in: Coastal Wetlands Restoration, Routledge, 1–16, ISBN 978-0367863081, 2021.
Yamashita, H., Mossman, H., McGrath, T., Taylor, E., Hayashi, A., Austin, W., and Maynard., C.: Communication and Engagement, in: Saltmarsh Restoration Handbook – UK & Ireland, edited by: Hudson, R., Kenworthy, J., and Best, M., Environment Agency, Bristol, UK, https://catchmentbasedapproach.org/learn/saltmarsh-restoration-handbook (last access: 23 February 2022), 2021.
Yang, S. L., Shi, B. W., Bouma, T. J., Ysebaert, T., and Luo, X. X.: Wave Attenuation at a Salt Marsh Margin: A Case Study of an Exposed Coast on the Yangtze Estuary, Estuar. Coast., 35, 169–182, https://doi.org/10.1007/s12237-011-9424-4, 2012.
- Abstract
- Introduction
- Challenge 1: understanding how salt marshes mitigate coastal flood risks
- Challenge 2: designing managed realignment to mitigate coastal flood risks
- Challenge 3: implementing managed realignments for coastal communities
- Challenge 4: developing participatory approaches to stakeholder involvement
- Towards co-producing MR schemes with coastal communities
- Conclusions
- Data availability
- Author contributions
- Competing interests
- Disclaimer
- Acknowledgements
- Financial support
- Review statement
- References
Please read the editorial note first before accessing the article.
- Article
(2190 KB) - Full-text XML
- Abstract
- Introduction
- Challenge 1: understanding how salt marshes mitigate coastal flood risks
- Challenge 2: designing managed realignment to mitigate coastal flood risks
- Challenge 3: implementing managed realignments for coastal communities
- Challenge 4: developing participatory approaches to stakeholder involvement
- Towards co-producing MR schemes with coastal communities
- Conclusions
- Data availability
- Author contributions
- Competing interests
- Disclaimer
- Acknowledgements
- Financial support
- Review statement
- References