the Creative Commons Attribution 4.0 License.
the Creative Commons Attribution 4.0 License.
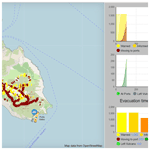
Assessing the effectiveness and the economic impact of evacuation: the case of the island of Vulcano, Italy
Costanza Bonadonna
Ali Asgary
Franco Romerio
Tais Zulemyan
Corine Frischknecht
Chiara Cristiani
Mauro Rosi
Chris E. Gregg
Sebastien Biass
Marco Pistolesi
Scira Menoni
Antonio Ricciardi
Evacuation planning and management represent a key aspect of volcanic crises because they can increase people's protection as well as minimize potential impacts on the economy, properties and infrastructure of the affected area. We present an agent-based simulation tool that assesses the effectiveness of different evacuation scenarios using the small island of Vulcano (southern Italy) as a case study. Simulation results show that the overall time needed to evacuate people should be analysed together with the percentage of people evacuated as a function of time and that a simultaneous evacuation on Vulcano is more efficient than a staged evacuation. For example, during the touristic (high) season between July and August, even though the overall duration is similar for both evacuation strategies, after ∼ 6 h about 96 % of people would be evacuated with a simultaneous evacuation, while only 86 % would be evacuated with a staged evacuation. We also present a model to assess the economic impact of evacuation as a function of evacuation duration and of the starting period with respect to the touristic season. It reveals that if an evacuation lasting 3 to 6 months was initiated at the beginning or at the end of the touristic season (i.e. June or November), it would cause a very different economic impact on the tourism industry (about 78 %–88 % and 2 %–7 % of the total annual turnover, respectively). Our results show how the assessment of evacuation scenarios that consider human and economic impact carried out in a pre-disaster context helps authorities develop evacuation plans and make informed decisions outside the highly stressful time period that characterizes crises.
Evacuation is a key measure used in emergencies that can save lives and reduce human impact (e.g. Moriarty et al., 2007; Tomsen et al., 2014; Thompson et al., 2017). The call for evacuation is often taken under periods of pressure and uncertainty (Bebbington and Zitikis, 2016) and is often a costly decision that, depending on how it is managed, can lead to both positive and negative outcomes (Doyle et al., 2014). Miscalculation or delays in the key phases of the evacuation process, such as, for example, the timing as to when the evacuation order is issued, the channels and sources through which the order is communicated to the public, the time required by the population to process and implement the evacuation order, and evacuation logistics and routes, can significantly reduce the evacuation effectiveness (Sparks, 2003; Sorensen and Sorensen, 2007; Lindell et al., 2019). Evacuation planning carried out in a pre-disaster context provides a better understanding of when to issue evacuation orders, who should be evacuated at what time, which routes and alternate routes should be considered, where evacuees should go, what resources are needed and how long the evacuation might last (MCDEM, 2008; Marzocchi and Woo, 2009). In this regard, volcanic crises differ from many other natural hazards, as they are often associated with an unrest phase, during which most volcanic systems exhibit precursors from hours to days, weeks and even months before the onset of an eruption (Gregg et al., 2015). The unrest phase is typically associated with long-lasting, time-dependent uncertainties regarding forecasts on where, when and even if a future eruption will take place. However, it also represents an important opportunity during which preparedness activities can, and should, be initiated with the aim of increasing resilience of the system as well as facilitating the potential evacuation.
Evacuation orders can be issued before the actual onset of an eruption when increasing volcanic unrest is observed. This type of evacuation is an important preventive measure and an efficient response to minimize human impacts (Baxter et al., 1998; Leone et al., 2019; Wilson et al., 2012). If planned and implemented well, preventive evacuation can save lives, as was the case in many past volcanic emergencies, including the 1991 eruption of Pinatubo in the Philippines, the 2006 and 2010 Merapi eruptions in Indonesia, the 2017–2019 eruption of Mt Agung in Bali, and the 2017–2018 eruption of Manaro Voui in Vanuatu (e.g. Leone et al., 2019). Evacuation can also be initiated after the beginning of an eruption, especially in the case of short-fuse events with little to no warning. In these situations, people may have to be evacuated due to the approaching of potentially impactful hazards such as lava flows, pyroclastic density currents (PDCs), lahars and tephra fall (e.g. 2021 eruption of Cumbre Vieja, La Palma, Spain, https://volcano.si.edu/volcano.cfm?vn=383010, last access: 26 March 2022). However, failure to evacuate in anticipation of an eruption or of the associated primary and secondary hazards can lead to catastrophic outcomes as seen during the 1985 Nevado del Ruiz eruption in Colombia and during the 2018 Fuego eruption in Guatemala (Voight et al., 2013; IFRC, 2019; Leone et al., 2019).
Unlike other emergencies, the duration of volcano-related evacuations can last for days, months or even years depending on the style of eruption and its impacts on the landscape and can result in long-lasting or even the permanent relocation of communities (e.g. Soufrière Hills, Montserrat, and Tungurahua, Ecuador; Barclay et al., 2019). Evacuations involving long durations mostly occur because periods of elevated unrest or eruptive activity can be protracted, post-eruption activity such as the remobilization of pyroclastic deposits by water (i.e. lahars) and wind (i.e. ash storms) can continue threatening communities, and/or the damage can be so overwhelming that people and their government lack the resources to rebuild in a timely period.
While evacuations can save lives, they are often costly and may trigger adverse economic and social impacts (e.g. Woo, 2008; Bouwer et al., 2011). Additionally, the consequences of a certain hazardous phenomenon can be lower than predicted. As an example, since the 1950s, 75 % of evacuations issued due to tsunami warnings turned out to be either non-events, or the tsunami generated was not as impactful as anticipated (The Economist, 2003; Selva et al., 2021). In this context, the potential economic impacts of an eruption should be accounted for in the process of decision-making for evacuations. According to Meyer et al. (2013), the management of natural risks can result in five different types of costs (Table 1): first, direct costs that result from the physical destruction of assets due to the interaction with hazards; second, business interruption costs that refer to losses that occur in areas directly affected by the hazard when people are not able to carry out their work because their workplace is destroyed, damaged or inaccessible; third, indirect costs that are induced by either direct damages or business interruption costs (e.g. production losses for suppliers and customers of enterprises; they can occur inside or outside of the hazard zone); fourth, intangible costs that concern damages to goods and services for which market prices do not exist, such as impacts on the environment, health or cultural heritage; and fifth, risk mitigation costs, which include risk management planning and adaptation plans; hazard modification, monitoring and early warning; emergency response; and evacuation. This category itself can also be divided in subgroups of costs such as direct (any action taken for mitigation infrastructures), indirect (secondary costs such as economic disruption due to mitigation measures) and intangible (e.g. environmental damage due to a change in agriculture practices) (Meyer et al., 2013). In this study, we are mostly concerned with the third type of cost related to the interruption of economic activities (i.e. tourism) as a result of a prolonged evacuation.
We present here a novel methodology to couple an evacuation model with an assessment of its potential economic impact. We use the island of Vulcano in the Aeolian Islands of southern Italy to illustrate strategies for the assessment of the effectiveness of an evacuation as well as the associated economic impact on the island's main source of revenue during periods of varying population on the island. On Vulcano, the low touristic season (i.e. when fewer visitors are on the island) occurs during the months of November to March, while the high season is in July–August. We developed an agent-based model (ABM) in GIS (geographic information system) space using the AnyLogic® software platform to assist emergency managers and assess the effectiveness of specific evacuation parameters, i.e. the number of people present on the island (during the low and high touristic seasons), type of evacuation (simultaneous whole community evacuation or sequentially staged evacuation of different areas), eruption probability, exposure and timing (before, during or after the eruptive event). Modelling using an ABM has been used in evacuation simulation extensively (e.g. Bae et al., 2014; Hilljegerdes and Augustijn-Beckers, 2019), and it has many advantages compared to aggregate and static approaches, as it allows us to incorporate individual level behaviours, event scheduling, dynamics of agent interactions, flexibility and a natural description of the evacuation process (Mas et al., 2015). In addition, the platform AnyLogic® allows for visually observing and assessing the evacuation scenarios. A strategy to assess the economic impact of an evacuation based on the analysis of the consequences on the main economic activity (i.e. tourism) is also presented.
The next section provides a conceptual background related to effective evacuation, types of evacuation methods and evacuation modelling, while Sect. 3 describes the study area. Section 4 illustrates the methodology adopted in our analysis, while Sects. 5 and 6 present and discuss the results of the assessment of evacuation efficiency as well as the assessment of the economic impact of an evacuation considering different durations and the starting time of both a total and partial evacuation (i.e. evacuation of individual areas); the recent unrest on Vulcano is also discussed in relation to the work presented here. Section 7 provides conclusions.
Han et al. (2007) developed and described a four-tier evacuation effectiveness framework, by looking at evacuation time, individual evacuation time, exposure over time and spatiotemporal exposure measures. The effectiveness of evacuation planning and operations for volcano emergencies can be assessed using this four-tier framework. One of the most common goals of evacuation analysis and planning is to improve the effectiveness of evacuation by reducing evacuation time to minimize the adverse impacts associated with people leaving their place of employment or study or their homes. Several methods have been proposed to improve the effectiveness of emergency evacuation such as enhancing the outcome of an evacuation order and dissemination of warning messages, controlling flows and movements in and out of designated areas, implementing a staged evacuation, directing people to the best evacuation routes, and focusing on flexibility to plan a possible evacuation (Abdelgawad and Abdulhai, 2009; Gaudard and Romerio, 2015).
In this paper we distinguish between “evacuation time”, defined as the time required for the last person to evacuate an emergency zone (Urbanik, 2000), and “evacuation duration”, which represents the period during which a community has been removed from a risky area. In addition, we define “evacuation effectiveness” as the time required to evacuate a certain fraction of the population (e.g. 95 %) (Han et al., 2007). The evacuation time of individuals or families depends on behavioural, logistical, perceptual and communicative factors (Tomsen et al., 2014). In order to minimize evacuation time, it is, therefore, important to reduce the evacuation warning time (time it takes for the evacuation warning to reach each individual), evacuation preparedness time (time it takes for individuals to prepare for evacuation after receiving an evacuation warning) and evacuation travel time (time it takes for individuals to travel from their residence to designated evacuation assembly areas). Each of these time segments varies from person to person and family to family depending on their demographic attributes, preparedness levels, and access to information and resources (Jumadi et al., 2019; Lechner and Rouleau, 2019).
2.1 Simultaneous and staged evacuations
Evacuation can be implemented using simultaneous or staged methods. In the simultaneous evacuation, people in an exposed area are informed and expected to evacuate simultaneously. In the staged evacuation, the exposed area is divided into several zones, and people in each zone are evacuated according to a specific order (Sbayti and Mahmassani, 2006). Both simultaneous and staged evacuations have been used in past emergencies. Staged evacuations have been frequently used during hurricanes and for the 2000 Los Alamos wildfire in New Mexico (Malone et al., 2001; Farrell, 2005; Wolshon et al., 2006). Simultaneous evacuations are often used during sudden emergencies when rapid evacuation is necessary (e.g. earthquake, landslide and industrial accidents), whereas staged evacuation is considered more effective when sufficient lead time exists to prepare for evacuation or when resources are limited for the simultaneous evacuation of the whole population. Chen and Zhan (2008) also found that simultaneous evacuations are more suited in areas of low traffic congestion, whereas staged evacuation may be the most effective in high-population-density areas and complex street networks. In the case of staged evacuation, the number of stages can influence the evacuation effectiveness, and thus optimizing the number of stages is essential in reducing delays during the evacuation process (Chien and Korikanthimath, 2007). Jumadi et al. (2019) developed a staged evacuation using a spatial multi-criteria analysis for the prioritization of evacuees and found that while the staged evacuation was more effective in reducing potential traffic congestion, the simultaneous evacuation still showed better results in reducing the population at risk.
2.2 Evacuation modelling and simulation
Several simulation and modelling approaches have been proposed and used for evacuation including cellular automata, game theory, discrete events, multi-criteria decision support systems (Cole et al., 2005; Marrero et al., 2013), and agent-based (Voight et al., 2000; Jumadi et al., 2016a, b) and experimental methods (Yang et al., 2015). Evacuation modelling has been performed for small- and medium-scale emergencies such as building fires and structural blasts (Pluchino et al., 2015) and those occurring in metro stations (Wang et al., 2013), oil and gas platforms and refineries (Cheng et al., 2018), and university campuses (Asgary and Yang, 2016). Larger-scale emergency evacuations have also been modelled, such as volcanic eruptions, flooding and hurricanes (Jumadi et al., 2016; Bernardini et al., 2017; Fahad et al., 2019). Modelling with an ABM has emerged as a suitable and promising framework for evacuation analysis and planning in recent years (Chen and Zhan, 2008; Liang et al., 2015; Jumadi et al., 2019). An ABM is appropriate for modelling complex and interactive systems (Gilbert and Bankes, 2002) such as emergency evacuation because it combines behavioural attributes with spatial and environmental data (Brown and Xie, 2006). Moreover, an ABM can provide a more realistic evacuation simulation with respect to the aforementioned approaches by incorporating human agents to the geographical environment (Mas et al., 2012; Joo et al., 2013).
The Aeolian Islands, which earned UNESCO (United Nations Educational, Scientific and Cultural Organization) World Heritage status in 2000, are a volcanic arc located in the Tyrrhenian Sea (25 km north of Sicily) associated with the subduction of the African plate under the Eurasian plate. The volcanoes of Stromboli on the island of Stromboli and La Fossa on the island of Vulcano are the youngest and most active volcanic edifices (Selva et al., 2020). Vulcano is the southernmost of the seven Aeolian Islands, having a surface area of ∼ 20 km2 and containing five main urban settlements: Vulcanello, Porto, Lentia, Piano and Gelso (Fig. 1). The areas of Vulcanello and Porto both have mixed land use zones with commercial, residential and touristic activities. Piano is mostly a relatively rural residential area with one dual primary and middle school (for children up to 14 years in age). Lentia and Gelso are small residential areas (associated with <4 % of total residents on Vulcano). Vulcano has a few local critical facilities and infrastructure that include three helipads, one main port (Porto Levante) and two smaller ports (Porto Ponente and Porto Gelso), one main power plant in Porto and one secondary solar plant in Piano as well as one telecommunication station, one desalination plant, and one wastewater plant in Porto (Fig. 1). The road network is limited with only one road connecting Porto and Piano (Galderisi et al., 2013; Bonadonna et al., 2021). Vulcano's predominant economic activity is tourism (Galderisi et al., 2013; Aretano et al., 2013; Bonadonna et al., 2021). The island's economy and urbanization have been growing fast since the 1980s by attracting tourists from Italy and other countries, particularly during the summer season. Vulcano has a fluctuating population ranging from about 800 residents in the winter to monthly peaks of about 22 000–28 000 visitors in July–August (Bonadonna et al., 2021). With an increasing number of visitors and seasonal workers, volcanic risk also increases. Emergency management, particularly evacuation planning and preparedness, has also become an important issue for the island.
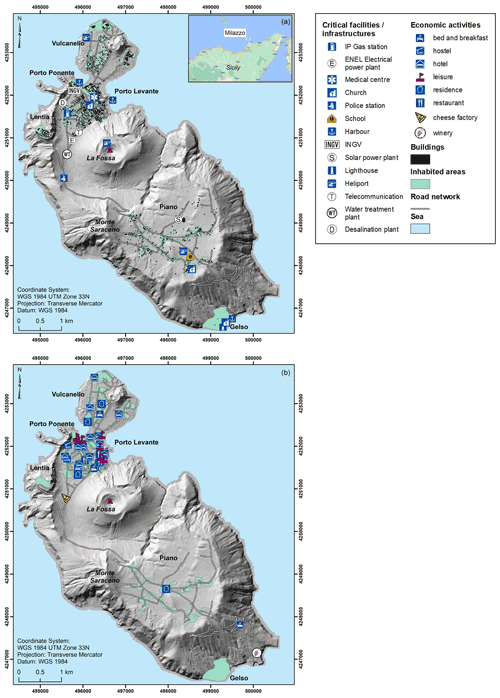
Figure 1(a) Built-up areas, critical infrastructure and (b) economic activities on the island of Vulcano. Inset shows the island's location in relation to mainland Sicily and the closest large port, Milazzo. The roads and the map of the inset are extracted from © OpenStreetMap (https://www.openstreetmap.org/copyright, last access: 26 March 2022). INGV: Istituto Nazionale di Geofisica e Vulcanologia.
3.1 Geological settings
In terms of geological settings, Vulcano consists of several overlapping volcanic structures including two caldera systems (Il Piano caldera to the south and La Fossa caldera in the central portion of the island) and a smaller structure (Vulcanello) in the north. A stratovolcano (i.e. La Fossa cone) rests within the La Fossa caldera, while three smaller and coalescing pyroclastic cones sit atop the Vulcanello islet. Subaerial volcanic activity on the island dates to 135 and 120 ka (Zanella et al., 2001), with the La Fossa cone (hereafter referred to simply as La Fossa) starting at ∼ 6 ka and being the current most active system (Dellino et al., 2011; De Astis et al., 2013). The last eruption of La Fossa was a long-lasting vulcanian cycle that occurred between 1888–1890 (Mercalli and Silvestri, 1891). The eruption produced the emission of ballistics and tephra fallout, while the intense remobilization of the tephra-fallout deposits by rainwater produced lahars (Di Traglia et al., 2013). The most likely hazards associated with eruptive activity of La Fossa are tephra fallout (including ballistic projectiles) and pyroclastic density currents as well as gas emissions, volcanic debris flows, lahars, ground deformation and seismicity that can also occur during the quiescent and unrest states (Selva et al., 2020). However, it is also important to consider that the activity of La Fossa has been characterized by a large variety of eruption styles, including effusive activity and explosive events. Among this variety, hydrothermal events of various intensity have occurred and are associated with impactful hazards such as blast, diluted PDCs and ballistic fallout, with the most violent being the Breccia di Commenda eruption dated around 1300 (Rosi et al., 2018; Pistolesi et al., 2021). It is thus important to distinguish between magmatic events, for which the main driver is the magma rising to the surface, and hydrothermal (or phreatic) events, for which the main driver is the interaction amongst water, rocks, and magmatic heat and gas (e.g. Barberi et al., 1992; Rouwet et al., 2014; Stix and de Moor, 2018). By their nature, hydrothermal events may be more difficult to predict than magmatic unrest, and they can also happen outside the main active vent (as often been the case at La Fossa). In fact, the La Fossa system is a permanent and powerful emitter of fluids whose flow is maintained by an elevated gas overpressure in the subsoil (Selva et al., 2020). Even modest disequilibrium in the supply of fluids can trigger explosive eruptions as the numerous cases that have occurred on Vulcano after the Breccia di Commenda in the last 8 centuries demonstrate (e.g. 1444 CE, 1550 CE, 1727 CE, 1873–1876 CE; Selva et al., 2020). This is also the case of other volcanoes that have been associated with recent and sudden explosions such as White Island and Tongariro in New Zealand (Breard et al., 2015; You Lim and Flaherty, 2020), Ontake in Japan (Oikawa et al., 2016), and Turrialba and Poás in Costa Rica (Alvarado et al., 2016; de Moor et al., 2016). Most of these volcanoes are located in remote areas, so hydrothermal events represent a threat mostly for tourists in close proximity to them; however, in Vulcano they represent a serious threat also for residents that live very close to the volcano (e.g. Porto area on the north of La Fossa). The main infrastructure (including the two ports of Porto Levante and Porto Ponente, the telecommunication station, and the main power plant) and the majority of economic and touristic activities are concentrated in the Porto area also located just north of the La Fossa cone (Fig. 1). Therefore, in the case of Vulcano, the potential for evacuation becomes an important issue even in the case of weak unrest. Both a hydrothermal explosion and a magmatic eruption would be especially challenging events during which to manage an evacuation if they happened during the high tourist season (July–August) and with little or no warning, as has been the case for the recent small but deadly eruptions at touristic places mentioned above (e.g. While Island and Ontake).
3.2 Civil-protection system in Italy
In order to reduce the potential volcanic impact, scientists, including those of the Istituto Nazionale di Geofisica e Vulcanologia (INGV), of selected research institutions (Centri di Competenza) and of the Italian Consiglio Nazionale delle Ricerche Institute for Electromagnetic Sensing of the Environment (CNR-IREA), continuously monitor all active volcanoes in Italy, including La Fossa, periodically transferring information on the state of volcanic activity to the national and regional decision makers each with defined authorities, roles and responsibilities; all these institutions are part of the overall civil-protection system in Italy (legislative decree “Codice della protezione civile”, 2018). In Italy, in fact, civil-protection activities are not assigned to a single body but represent complementary tasks attributed to an integrated system composed of both public and private and both national and territorial (regional and local) structures. The Italian Civil Protection Department (at the national level) is a structure of the Presidency of the Council of Ministers and coordinates the entire civil-protection system.
Another important aspect to consider is that volcanic risk management in Italy is based on an alert level system, and four volcanic alert levels have been identified based on monitoring parameters that describe the state of activity of each Italian volcano. These levels correspond to four colours (green, yellow, orange and red), which are indicative of the level of activity and of its possible evolution, including the shift from local to national impact scenarios. In fact, volcanic activity can also generate local impact events, which are managed at the local level by the appropriate institutions (i.e. regions and municipalities). Recently, the Italian Civil Protection Department financed detailed studies to improve the current understanding of the volcanic system and the whole range of potential volcanic hazards on the island of Vulcano (Selva et al., 2020). Based on these results, an updated version of the alert levels for Vulcano was issued at the end of 2021. These alert levels have been included in the national civil-protection plan for volcanic risk on the island. The plan also describes the “national-level operational phases”, which include the mitigation actions that all the stakeholders involved in the emergency management must take, and foresees the evacuation of the population before the eruption onset in the case of increasing volcanic unrest.
3.3 Potential consequences of a volcanic crisis on Vulcano
Although it would appear to be a quick and small operation, the evacuation of the island under different weather and marine conditions, occurrence of different hazards (gas, tephra fall, PDCs, lava flows, lahars, landslides and tsunami) and various tourist seasons (low, middle and high) could result in different decisions and actions. Unforeseen factors might also limit the availability and efficiency of evacuation (e.g. damaged ports). In addition, forecasting volcanic eruptions and managing volcanic crises represent an important challenge for both scientists working in observatories (e.g. geochemists, geophysicist, geologists and volcanologists) and civil authorities such as those associated with emergency management (i.e. civil-protection system in Italy). Many impediments may be encountered in interpreting key aspects such as (i) whether or not unrest will lead to an eruption; (ii) the nature of explosive activity (magmatic or hydrothermal); (iii) the eruptive style (i.e. effusive, explosive or both); (iv) the potential activation of lateral vents; (v) the eruption magnitude (i.e. erupted mass) and intensity (i.e. the rate of discharge of magma that is correlated to plume height); and (vi) the type, extension and timing of hazards with the potential to impact human life and the infrastructure supporting evacuation whether occurring in either the unrest phase or eruptive phase or both. The interpretation of scientific data complicates the decision-making process for the officials (Fearnley, 2013).
High levels of scientific uncertainty may thus translate to increased difficulty for emergency managers to understand the value of evacuation (measured in terms of human lives saved) and the costs associated with any evacuation associated with unrest that does not result in eruption. Although successful forecasts have been made (e.g. Mt St Helen's, USA, in 1980; Mt Redoubt, USA, in 1989–1990; Pinatubo, Philippines, in 1991), alarms raised that are not followed by hazards impacting exposed areas sometimes cause both scientists and other officials to lose credibility among the public (e.g. Sparks, 2003; Tilling, 2008). In addition, a volcanic crisis can easily result in an economic crisis, with or without an evacuation and an eruption occurring. Interesting examples are those of the 1983–1985 volcanic crisis at Rabaul caldera (Papua New Guinea) and of the 1999 volcanic crisis of Tungurahua (Ecuador). In the first case, a dramatic short-term increase in seismicity and ground deformation led to an intensification of disaster-preparedness activities and voluntary evacuations by villagers, which resulted in substantial losses of revenue due to business interruptions and a large cost of emergency preparations; in the end, many people thought that 2 years of preparation was a waste of money even though public awareness of potential volcanic hazards increased and the community became more resilient (Hastings, 2013; Tilling, 2008). In the second case, an economic crisis at the both local and national level resulted from an evacuation that followed a successful forecast, as most of the community's economic activity depended on tourism (Lane et al., 2003). This is also valid in the case of the island of Vulcano. Even without an evacuation order, the increasing level of unrest may cause the local people to leave the island if they believe that tourism on the island may be affected negatively by the increasing volcanic activity. This is especially true, since most business owners are not from Vulcano, and they may decide to relocate their activities. In both cases, the economy of the island would be negatively impacted. Additionally, there could be significant negative economic impacts on Vulcano associated with changes in the volcano alert level even when an eruption or evacuation does not occur, as Peers et al. (2021) described for the protracted unrest at Long Valley caldera, California, in the USA.
Volcanic unrest and eruptions can also have positive impact on the economy. As an example, volcano geotourism has become increasingly popular around the world (e.g. Dóniz-Páez et al., 2020; Quesada-Román and Pérez-Umaña, 2020). It is estimated that between 150 and 200 million people visit volcanic and geothermal environments on an annual basis (Heggie, 2009; Bird et al., 2010; Erfurt-Cooper and Cooper, 2010; Erfurt-Cooper, 2011). The island of Vulcano appeals to a wide range of tourists: some visit to relax and/or for health reasons, whereas others are attracted to the volcanic landform and geothermal features. Thus, an increase in unrest may attract more adventure-driven tourists, unless such visits are curtailed by civil authorities as a result of an increased likelihood of eruption and resulting limitation of the number of people on the island. If the increasing activity on the island results in an evacuation and finally in an eruption, many tourists interested in natural areas and adventure may still want to visit the island once the activity is back to a pre-eruption phrase and the risk is decreased.
4.1 Agent-based modelling of pedestrian evacuation
The Vulcano Evacuation Simulation Tool has been developed using the AnyLogic platform (version 8.7.5), which provides ABM capabilities as well as GIS spatial data incorporation. Our simulation tool includes four main agents, each of which is described below (i.e. hazard, evacuees, ferries and ports, and agents' environment). In order to correctly characterize such agents and tailor the analysis to the specifics of the island, risk factors including hazard, vulnerability and exposure of both the community and critical assets must be known as well as their dynamics over time. Such key elements have been extensively addressed and analysed in a paper presenting a novel risk assessment model for volcanic risk, named ADVISE (integrAteD VolcanIc risk asSEssment), based on long-term research efforts of the authors and applied to the island of Vulcano (Bonadonna et al., 2021). All the needed aspects and elements required to assess the various indicators are provided there and rely on extensive surveys and data collection carried out in the last 10 years. The following ABM uses the outcomes of such data collection and risk assessment, especially as far as hazard and exposure are considered. Some aspects of systemic vulnerability are also considered related to the accessibility of the three ports of the island and their intrinsic characteristics.
4.1.1 Hazard (volcano) agent
We define La Fossa volcano as a physical agent with specific behaviour and states defined based on scientific evaluation. It is important here to differentiate between the state of the volcano (quiescence, unrest, and impending/ongoing eruption) with alert levels (green, yellow, orange and red) identified by the Italian Civil Protection Department. In this ABM, La Fossa volcano has three main states including quiescence (i.e. normal conditions), unrest (divided into shallow hydrothermal crisis and deep hydrothermal crisis), and impending or ongoing eruptive activity (Fig. 2). It is important to consider that, for the sake of illustration, these states have been simplified with respect to the alert level system specific to Vulcano (https://rischi.protezionecivile.gov.it/it/vulcanico/vulcani-italia/vulcano, last access: 26 March 2022). In any case, modifications can be made to reflect the specificities of individual volcanoes. Both the quiescence and unrest states are different for different volcanoes. In Vulcano, the quiescence state mostly consists of fumarolic emissions (mostly concentrated in two main fumarolic fields located on the northern rim of the active crater of the La Fossa cone and at the beach of Baia di Levante, in the Porto Levante area), ground deformation, earthquakes and accompanying landslides (Barberi et al., 1992; Selva et al., 2020), while the unrest state is mostly related to a shallow or deep activity of the hydrothermal system associated with various levels of increase in the flux and temperature of gas emissions combined with seismicity and the deformation of the volcanic edifice. As already mentioned, phreatic eruptions can occur in all these volcano states with little or no warning. However, in our model, we assume that the simulation of evacuation starts before an eruption when the volcano is in the impending-eruption state (Fig. 2). Forecasting of a volcanic eruption can be based on various functions, e.g. exponential hazard function (Ho, 1992; Cornelius and Voight, 1994; Chastin and Main, 2003; Connor et al., 2003; De la Cruz-Reyna and Reyes-Dávila, 2001). The spatial exposure of evacuees can also be determined based on the probability of being impacted by an eruption using the models of Aucker et al. (2013) and Brown et al. (2017) as well as hazard analyses of Vulcano (Dellino et al., 2011; Biass et al., 2016a, b; Bonadonna et al., 2021; Gattuso et al., 2021). The probability of being impacted by various volcanic hazards depends on eruption dynamics (i.e. gas, occurrence of ballistics, tephra fallout, lava flows, blast surge-like PDCs and lahars) as well as topography and atmospheric conditions (e.g. wind speed and direction). Hazard maps for Vulcano exist that describe the potential extent and intensity of tephra fallout and ballistic projectiles (Biass et al., 2016a, b), PDCs (Dellino et al., 2011) and lahars (Gattuso et al., 2021). However, given that before the actual eruption (phreatic or magmatic) takes place, the extent and intensity of the associated hazards are not known, we consider here the evacuation of certain areas to be based on the worst-case scenario, e.g. occurrence of PDCs and ballistic ejection in the case of Vulcano which could impact the whole La Fossa caldera (including the Porto area) and part of the Piano caldera (e.g. Dellino et al., 2011; Biass et al., 2016b).
4.2 Evacuee agent
We combined and expanded the Sorensen and Mileti (2014) and Stepanov and Smith (2009) multi-step evacuation process models to include four main time segments: (1) warning issuance, i.e. the step from when unrest or evidence of a hazard appears to when decision makers decide to issue the warning; (2) warning diffusion, i.e. the time from when the warning is issued to when the warning reaches the intended audiences; (3) preparation for evacuation, i.e. the time from when the warning reaches the intended audience to when they are ready to evacuate (this includes the time required to organize their departure and secure their belongings that are left behind, e.g. house, car(s), other vehicles and boats); and (4) evacuation movement (Fig. 3). The agent is created, and its initial state is set to “before warning” (or normal). As soon as a warning is issued, the agent's states change from before warning to “warning issued”, corresponding to an evacuation order. Transition from this state to the “warning received” is controlled by a timeout-triggered transition. We use a truncated normal distribution for this transition with minimum and maximum time values that can be set by evacuation planners before running the simulation. Use of this distribution allows us to limit the lower bound to 0 and the upper bound to a finite value. Transition from the warning-received state to the prepared state is also handled by a truncated normal distribution that can be set by the evacuation planner. However, this transition is triggered only if the evacuation is either a simultaneous evacuation or the evacuee is located in the assigned evacuation stage.
The order of evacuation during a staged evacuation is based on the proximity to the hazard, with the most exposed people being evacuated first. In Vulcano, the northern part of the island will be evacuated from Porto Levante and Porto Ponente, and the south of the island will be evacuated from Porto Gelso. In our simulations, people in Porto and Piano will be simultaneously evacuated first, and people in Vulcanello will be evacuated last. However, to provide the emergency planner with more flexibility, the simulation allows the users to set the evacuation order as needed. Evacuation time depends on the evacuees' walking speed and their distance to the closest active port. We consider the walking speed as a uniform distribution, but the model allows the lower and upper bounds of this distribution to be set depending on the environmental situations and population scenarios being analysed. For the sake of illustration, we assume here only pedestrian evacuation, but the simulation can be adapted to also include evacuation by vehicles or a combination of the two. We recognize that while walking may be a more feasible option for those in the northern part of the island (Vulcanello and Porto), it may be more difficult for the people in the southern part of the island (Piano). Upon arriving at the closest active port, evacuees wait for ferries. Given that our simulations are based on the assumption that the evacuation takes place before the eruption, evacuees are considered to be evacuated once the ferries arrive and are boarded. However, in the case that the evacuation was carried out during the eruption, people should be considered evacuated once the ferries actually leave the ports, as both ports and ferries could be impacted by the eruption.
4.2.1 Ferry and port agents
Ferries transport evacuees from ports on Vulcano southward some 44 km to the large port of Milazzo on the northern shore of Sicily (Fig. 1a inset). As an evacuation order is issued, ferries are mobilized in the Milazzo port. In our simulations, ferries have a capacity of 200, 400, 600 and 800 passengers and an average speed of 50 km h−1, but these variables can be changed. In fact, ferry speed depends on the weather and marine conditions that can be set by the users before running the simulation. As ferries arrive at the port, evacuees start boarding until full capacity is reached, at which point they travel back to Milazzo. If there are more requests, ferries continue going back to the assigned Vulcano ports; otherwise they stay in Milazzo. Port agents have two main states in our ABM including the normal and evacuation states (Fig. 4). As soon as an evacuation order is issued, the state of the ports changes from normal to evacuation through a message transition. Inside the evacuation state, two substates demonstrate whether a port has ferries to board evacuees or not. The transition between these two substates is controlled by the interactions between the ferries' agents and ports' agents.
4.2.2 Agents' environment
Two main GIS networks were created for this study. The first connects the three ports in Vulcano (Porto Ponente, Porto Levante and Porto Gelso) to the port in Milazzo (Fig. 1a). The second connects buildings in Vulcano (e.g. residential, commercial, hotels and facilities) with the road network created based on the existing road network on OpenStreetMap (Fig. 1).
4.2.3 Model setup
We illustrate our evacuation simulation tool by setting up two pre-eruption evacuation scenarios taking place during the low and the high touristic seasons. The scenario's initial conditions are summarized in Table 2. Figure 5 and Appendices A and B show the parameters and scenario settings and the main interface of the Vulcano Evacuation Simulation Tool. The low-season scenario involves 1000 people consisting of 300 local residents living in Piano, 100 residents living in Vulcanello and 600 people including residents, seasonal workers and tourists in Porto. The high-season evacuation involves a total population of 4600 consisting of 400 people each in Piano and in Vulcanello and 3800 people in Porto, where residents, tourists and seasonal workers are mixed across the different areas. For the sake of simplicity here we only consider the Porto, Piano and Vulcanello areas, as Lentia and Gelso are associated with <4 % of the residents. Both scenarios assume only pedestrian evacuation, where each evacuee is assigned a walking speed uniformly sampled between 0.8 to 1.6 m s−1 (Wood et al., 2018). The evacuation warning time follows a uniform distribution between 15 and 90 min, and the evacuation preparedness time also has a uniform distribution ranging between 30 and 120 min (Table 2). In addition, our simulations do not account for variable weather and marine conditions. Note that these parameters were chosen based on our knowledge of the area and are used only with the purpose of illustrating the functionality of the tool. All parameters can and should be identified by emergency managers based on the availability of information and on the range of conditions to be tested (e.g. people with reduced mobility or with health issues and evacuation using a variety of vehicles).
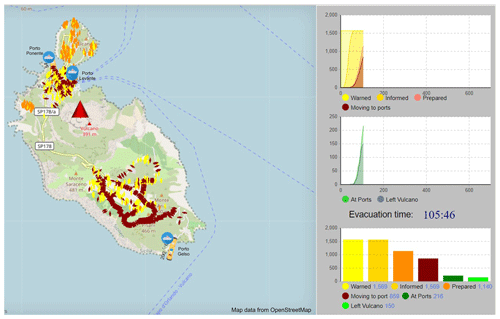
Figure 5Example of a pedestrian evacuation simulation run. The map on the left shows the movement of evacuees from different parts of the island to their designated or nearest port from where they will be evacuated by ferry (Porto Levante and Porto Ponente in the north and Porto Gelso in the south). Yellow colour represents people who have received the evacuation order; orange colour shows people who are prepared for evacuation; and brown colour shows people who are moving towards the ports. First graph on the top right shows the evolution in time of the number of people warned, informed, prepared and moving to ports; graph in the middle shows the number of evacuees at ports and number of people evacuated from the island with time; and graph on the bottom right shows the same information as above in a bar chart format. Evacuation time is indicated in minutes (in blue). See also Appendices A and B. SP178 indicates the main road that connects Porto and Piano.
4.3 Assessment of the economic impact of an evacuation
When the hazard level is high and human life is at stake, economic losses usually play little to no role in the decision of whether to evacuate. In less extreme situations, however, authorities weigh different factors, and different evacuation plans can be considered. In fact, the management of the crisis will take different courses depending on the evolution of the unrest and the time-dependent evolution of the hazard. Accurate data necessary for a reliable cost–benefit analysis are rarely available, especially in the context of small islands where they are aggregated at the level of the municipality. Furthermore, in the case of relatively simple economic systems such as that of a small island, complex and sophisticated models can be replaced by a set of reasonable hypotheses. Consequently, we present an approach to estimate the loss of revenue caused by a total or partial evacuation of the population on the island at any one time (i.e. residents, seasonal workers and tourists) due to an imminent eruption. Such an analysis is especially important in case of scenarios of long-lasting vulcanian cycles, such as that of the 1888–1890 eruption of the La Fossa volcano that would disrupt the island's economy for a long time (many months to years).
The data collection required to estimate the impact of an evacuation on the island's main source of revenue was carried out between 2014 and 2016. This investigation focused on tourism-related business activities. We spoke with owners and workers of shops, restaurants, hotels and a tourist office in May 2014 to constrain working seasons, business hours and consumer prices. We also spoke with the tourist office in Lipari to determine the number of tourists visiting Vulcano. This was supported with online research (2014–2016) to assess hotel prices that could not be obtained through discussions with personnel on site. Several booking websites were used in the case that the hotel did not have its own website.
While there are two main beaches between Porto and Vulcanello that serve as the main attraction for visitors overall, one of the most popular touristic activities on Vulcano is the mud pool in the Porto Levante area. The mud pool was initially developed around an exploration drilling site for geothermal exploitation in the 1950s (Faraone et al., 1986; Gioncada et al., 1995). Many people visit the island only for this reason. Tourists mostly come to the island during summer, and in addition to visiting the mud pool they also like to taste the local cuisine and take boat tours around Vulcano and/or around other Aeolian Islands. Hiking to and around the summit of La Fossa and daily visits to other islands are also popular activities. A variety of lodging and accommodation solutions are available on the island (Fig. 1b).
At the time of our survey in 2014 and web search in 2016, there were 17 hotels on Vulcano, of which only 4 were open the whole year (Fig. 1b). Those open during the middle season are not fully occupied. From June until September, they all operate almost at full capacity. In addition to the hotels, there were 21 B&Bs (bed and breakfasts), hostels and residences with 2 camping areas, as well as 40 apartments. All of them are open in the high season; few are open in the middle season. Most of the restaurants are closed during the low and middle seasons. From June to September, all 24 restaurants were open until after midnight and were always full of tourists. Both day trippers from Sicily and elsewhere in the Aeolian Islands and visitors spending the night on the island dine in these restaurants. Vulcano has dozens of stores located in the Porto area, mainly consisting of clothing and souvenir shops, but very few of them are open the whole year. The rest are open mostly around Easter until the end of October. One main supermarket and two smaller grocery stores are located in the Porto Levante area, which is the area within the broader Porto area defined by the presence of the main port on the island (Porto Levante) and relatively dense development. Like most of the Aeolian Islands, Vulcano has many notable activities for outdoor enthusiasts. Most all leisure activities (e.g. mud pool, motor car and motor bike rental, bicycle rental, and scuba diving) on the island are in the Porto and Vulcanello areas, although a few hiking trails exist in the Piano area and more remote area of Gelso in the far south of the island. Most activities are closed during the low season, but a few are in service during the middle season.
Three seasons have been identified based on the number of tourists, which include the low season (November to March) with no touristic activity on the island (most of the hotels, hostels, B&Bs and residences usually undergo maintenance activities), the middle season (April–May–June and September–October) with a gradual increase/decrease in the number of tourists (some of the restaurants, hotels, hostels and B&Bs open; repair of residences continues from April to June) and the high season (July–August) with monthly peaks that approach 22 000–28 000 visitors (18–23 times the number of residents; Bonadonna et al., 2021). Being the closest island to Sicily, Vulcano is an easy getaway for mainland day trippers, many of them coming to the island on their private boats and dining at the restaurants. In fact, buoys are available in Levante Bay, and mooring is possible at the Marina di Vulcanello jetty to the north of the bay or the Baia di Levante jetty to the bay's south inside the commercial port. All types of leisure activities and shops are functional during the high season.
Cheese and wine are also produced on Vulcano. The cheese factory La Vecchia Fattoria is situated on the western side of Porto and just off the road to Lentia. In 2016, the owner indicated that the farm included 280 goats, 40 cows and 30 sheep and that the main production takes place between March and October, i.e. the middle and high seasons. From November until February they have less goat milk because the goats are pregnant and/or feeding their lambs. While they have only a few clients in mainland Italy, exports are limited mostly to the Aeolian Islands, especially with supermarkets in Lipari, where the main income is derived. The main wine factory (Punta dell'Ufala) is located in Gelso, and the vineyards are dispersed on 5 ha of slightly steep hills between Piano and Gelso. According to personal communication with the owner (Ms Paola Lantieri) in 2016, the most delicate season for the grapes is between March and July because this is when the vineyard flourishes and becomes more susceptible to pests. They sell the wine mainly in Vulcano to hotels, restaurants and the grocery stores/supermarket and export some products to mainland Italy and the USA and Japan rather than elsewhere in the Aeolian Islands.
4.4 Methodology to calculate the revenues from touristic-business activities in Vulcano
Our analysis focused on the turnover created by tourism-related businesses, which provides the main income to the island's economy. The turnover represents the gross revenue that a business generates without considering associated expenses (e.g. food, water, energy and maintenance). The economic impact associated with an evacuation of the island is represented by the loss of this revenue. This revenue must not be confused with the added value provided by the national accounts, which includes profits, wages, interest and amortization but not intermediate goods and services. We consider neither the revenues from the grocery stores, supermarket and shops due to lack of sufficient reliable data nor the revenues from the cheese and wine factories because they are not tourism-related businesses. The income from maritime transport is also not included because it does not have a major impact on the local economy.
As mentioned in Table 1, different categories of costs are concerned when dealing with impacts from natural events such as those involving volcanic unrest and eruption that might necessitate an evacuation of people from the island. Our focus on Vulcano was identifying tangible business interruption cost related to the interruption of touristic activities. The revenues, expressed by the turnover, for all the touristic activities on the island, which will become the loss in case of an evacuation, are calculated for different seasons as part of the cost assessment. The main touristic business on the island can be defined as B&Bs, hostels and residences; restaurants and bars; hotels; and leisure activities. Each of these is described below.
4.4.1 B&Bs, hostels and residences
Data were collected from the internet and discussion with personnel on site for 7 B&Bs, 1 hostel and 6 residences (out of 21; we were unable to obtain data for the remaining 7 structures due to a lack of online information). The revenue for each season is calculated by multiplying the capacity, the price, the total days and the occupancy rate. In the equations below, H and M indicate the high and medium seasons, respectively.
where RH and RM represent the total revenues for the high and middle seasons, respectively; C represents the total capacity, i.e. maximum number of people that can be accommodated at a given place; PH and PM are prices per night per person; TH and TM are the number of total days estimated in calculations; and OH and OM are occupancy rates, i.e. the proportion of available accommodation occupied. As there are no official statistics available, simple assumptions are made for occupancy rates that are based on observations done over more than 10 years of research on the island, which are expected to be reasonable within a margin of 5 %–10 %. A rate of 50 % and 100 % is estimated for the middle and high seasons, respectively.
4.4.2 Restaurants and bars
Dine-in data (i.e. meal prices and total days open) were collected from discussions with owners/workers at 11 of the 24 restaurants and bars, but “take-away” (dine-out) revenues are not included. For this category the meal prices do not change with different seasons. The revenue is calculated by multiplying the capacity, the average meal price, the table turn, the total days and the occupancy rate. In the equations below, H, M and L indicate the high, middle and low seasons, respectively.
where RH, RM and RL are the total revenues for the high, middle and low seasons, respectively and capacity C, i.e. the total number of people the restaurant can host, is multiplied by the approximate meal price and table turn TT, i.e. number of times a table is occupied with different groups, to calculate the revenue per day RPD.
It is important to note that, during the high season H, the number of times a table can be occupied during working hours varies for each restaurant. For example, at Faraglione, which is small but popular restaurant and bar adjacent to Porto Levante, one table may turn as many as 20 times during a day because it is open from very early in the morning until very late at night. However, for the middle and low seasons, the time a table turns is fixed to once, considering a restaurant never works on full capacity during these seasons. TH, TM and TL represent the number of total days, and OH, OM and OL represent the occupancy rate for the high, middle and low seasons which is 100 %, 50 % and 15 %, respectively.
4.4.3 Hotels
We were able to collect the required data (capacity, prices and opening season) for 12 out of 17 hotels. As for the other missing facilities, there was no official website, or they were not open for us to speak with them when data were collected. In the equations below, H, M and L indicate the high, middle and low seasons, respectively.
where RH, RM and RL are the revenues for the high season, middle season and low season, respectively and PH, PM and PL indicate the price of a room for the high, middle and low seasons. Even though the middle season could be subdivided into two groups, as the months of June and September are busier than those of May and October, the same value was assumed, considering the limited data. TH, TM and TL represent the number of total days, and OH, OM and OL represent the occupancy rate for the high, middle and low seasons which is 100 %, 50 % and 15 %, respectively.
4.4.4 Leisure activities
While touristic attractions contribute an important amount of revenue to the economy of the island, they close for the low season, as do B&Bs and hostels, because there are insufficient numbers of tourists to keep the businesses open. The type and price of leisure activities are all determined based on discussions with the owners. The revenue per day RPD for seven groups of different activities (i.e. vehicle rentals, scuba diving, snorkelling, kayaking, guided boat tours, boat rental and mud pool) is calculated for both the high H and middle M seasons.
where TH is the total days of the high season (July and August, 62 d); OH is the occupancy rate during the high season, i.e. the proportions of activities occupied; and RH represents the revenue for the high season.
TM is the total days for the middle season (April–May–June and September–October, 152 d), and the occupancy rate is set to 40 %.
5.1 Evacuation effectiveness
Figures 6a and b and 7a and b show simulation results for simultaneous and staged evacuations during the low and high seasons, respectively. When considering evacuation time as a proxy for effectiveness, both simultaneous and staged evacuation scenarios are slightly faster during the low season (∼ 6.7 and ∼ 7.1 h, respectively) with respect to the evacuation during the high season (∼ 8.9 h for both). For the latter one, although both scenarios have equal evacuation times, their evacuation effectiveness differs (Fig. 7c). During the low season, a 95 % evacuation effectiveness is reached within ∼ 5.8 and ∼ 6.5 h for the simultaneous and staged evacuations, respectively (Fig. 6c). For the high season, a similar effectiveness is reached within ∼ 6.1 h (simultaneous) and ∼ 7.5 h (staged) (Fig. 7c). These results have two implications. Firstly, the simultaneous evacuation results in fewer people left exposed to an increasing hazard over time, which confirms findings from previous studies (e.g. Chen and Zhan, 2008; Jumadi et al., 2019). Secondly, a population increase of 360 % between the low and high seasons results only in an increase in evacuation time of ∼ 12 %. In fact, assuming that warning time and preparedness time distributions are independent of population size, the main aspects that could impact the evacuation time are the walking speed and the number and capacity of the ferries used for evacuation. For the case of Vulcano, the pedestrian density in the roads considered under both scenarios does not impact walking speed (the population density in the space, in our case roads, increases beyond a single person per square metre, which is not reached in Vulcano). In addition, the number and capacity of the ferries can equally accommodate the population increase during the high season. If a larger number of people had to be evacuated (e.g. 10 000 people as supposed to 4600), the time needed to evacuate 95 % of the population would nearly double because of the number of ferries (10) and associated capacity (200 to 800 passengers) set in the simulation. However, 1000 people (considered in the low-season scenario) and 4600 people (considered in the high-season scenario) can be almost equally managed by the capacity and number of ferries used.
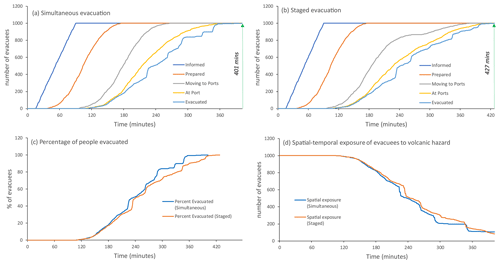
Figure 6Plots of evacuation simulations for the low-season scenario showing (a) a simultaneous evacuation, (b) a staged evacuation, (c) the percentage of people evacuated with time and (d) variation of exposure with time.
5.2 Determination of revenue on Vulcano
With the methodology explained in Sect. 4.3, the revenues from four different categories (hotels; hostels, B&Bs and residences; restaurants; and leisure activities) are calculated. Hotels and restaurants are the only two categories providing revenues during the low season (Table 3). With a monthly amount of about EUR 153 233, the revenue from hotels is 4.4 times greater than the revenue from the restaurants during the low season. From the beginning of April (beginning of the middle season), the tourist population starts to increase on the island. Equations (2), (3) and (5) are used to calculate the monthly revenues from hostels, B&Bs and residences and restaurants. The occupancy rate (OM) used for this season is 50 %. On the other hand, while calculating monthly revenue for the middle season for hotels and leisure activities, an average is taken due to different occupancy rates (OM) throughout the season. For leisure activities 40 % occupancy is considered, whereas 50 % of the occupancy rate is used for hotels. As seen in Table 4, hotels provide more than half (58 %) of the monthly revenue for the middle season with EUR 939 610, whereas the restaurants; hostels, B&Bs and residences; and leisure activities provide 29 %, 12 % and 1 % of the monthly revenue, respectively.
Table 4Revenues for each business activity on Vulcano during the middle season (April, May, June, September and October).
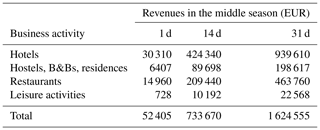
The touristic population reaches its peak during July and August. Thus, the occupation rate (OH) is considered to be 100 % for all the categories. The revenues are calculated by using Eqs. (1), (3), (4), (7) and (10). Hotels and restaurants provide the highest revenue for this period with 44 % and 46 % of total revenue, respectively, whereas leisure activities and hostels, B&Bs and residences contribute 4 % and 6 %, respectively (Table 5). While calculating revenue for the low and middle seasons, table turn (TT), i.e. the number of times a table is occupied by different groups, is considered 1. However, during July and August, restaurants are full of tourists, and a table in a restaurant is served more than once. Thus, TT varies for each restaurant while calculating the revenues for the high season.
It should be noted that the prices for hotels and hostels, B&B and residences are not constant during different seasons, and, in fact, they slightly differ for each month. The highest prices throughout the year are applied for the second and third week of August, which is considered summer vacation in Italy. An average price for each season is calculated based on website data. Additionally, different occupancy rates are considered to obtain a range of revenue for each season. About half of the yearly revenue (EUR 33 692 640) comes from hotels (Table 6). The other half is divided between the remaining three groups, with restaurants providing the second-highest revenue after hotels with 41 % of total revenue. Even though in Tables 3, 4 and 5 the monthly calculation is based on 31 d, the number of days considered in Table 6 is related to each individual month.
5.3 Analysis of potential economic impact of an evacuation
The evacuation results in a very different impact on the island's revenue, whether it starts at the beginning or at the end of the tourist season. The total loss of revenue (expressed by the turnover) is significant if the evacuation begins in June and lasts for more than 1 month (i.e. > EUR 1.5 million; Table 7, Fig. 8a). If it starts in November, the impact becomes significant if it lasts more than 6 months (i.e. > EUR 2 million; Table 7, Fig. 8a). The high season represents the critical period. The impact of an evacuation starting in November and June in the two Vulcano main touristic areas (Porto and Vulcanello) is also considered (Table 7, Fig. 8). A partial evacuation of Piano was not considered because most of the tourist infrastructure is located in Porto and Vulcanello, and, therefore, most of the turnover is related to activities in Porto and Vulcanello. Clearly the evacuation of only Vulcanello would result in a smaller loss of revenue with respect to a partial evacuation of Porto for any of the durations considered (i.e. < EUR 10 million). However, in the case of escalating unrest activity, the safety of people is typically prioritized with respect to economic factors. As a result, the areas that are the most exposed to the hazard (i.e. Porto) would be evacuated first.
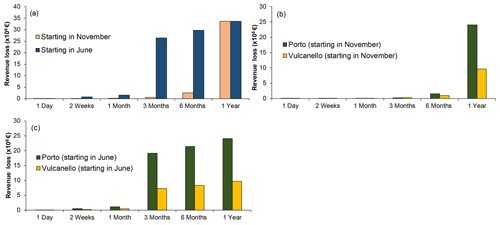
Figure 8(a) Total loss of revenue (EUR) for different evacuation periods starting in November and in June (whole of the island of Vulcano) and a partial evacuation of Porto and Vulcanello starting (b) in November and (c) in June (see Table 7 for original data).
6.1 Effectiveness of evacuation
The main objective of our study is to provide decision makers with an operational tool to investigate various evacuation scenarios. This evacuation simulation tool allows emergency managers to identify and optimize individual and organizational parameters (related to actions, behaviours, policies and resources) that minimize the evacuation time as crises evolve. The tool allows for estimating key indicators such as the minimum time necessary to fully accomplish the evacuation, which, in the context of volcanic crises, can be compared to eruption forecasts provided by monitoring networks. Together, these two aspects provide a comprehensive picture of the various components to pursue successful emergency management.
Although the overall evacuation time and the individual evacuation time are vital measures for enhancing the effectiveness of the evacuation process, they do not fully consider the dynamics of hazard and exposure during a volcanic eruption. In volcanic eruptions, hazard and exposure vary in time and space. In other words, the risk can increase because the probability of eruption might increase with time and because the actual exposure could be significantly higher a few hours after the evacuation order is issued compared to the first hour due to the movement of people towards the evacuation areas (e.g. ports), which are sometimes closer to the source of the eruption (La Fossa) than where they initiated the evacuation (e.g. Vulcanello). Therefore, to reduce exposure, the goal should be to evacuate more people more quickly (Han et al., 2007). The spatial exposure on Vulcano is complicated by the proximity of the main port to the La Fossa crater (i.e Porto Levante is located at the foot of the northwest flank of La Fossa). Particularly, for people in Vulcanello moving towards Porto Levante, evacuation requires that they get closer to the hazard source at La Fossa. While optimizing evacuation requires that evacuees move away from the hazard source, the evacuation of people on the northern side of Vulcano to either Porto Levante or Porto Ponente cannot be done without moving people closer to the hazard source, especially when moving people to Porto Levante because it is closer to La Fossa than Porto Ponente. Exposure could be reduced by moving people from the Porto Levante area to Porto Ponente, but the latter port can neither accept large ferries nor handle large volumes of people. It is, in fact, significantly smaller and characterized by shallower water than the facility at Porto Levante. Therefore, the planning of an effective evacuation should assess the evacuation time as well as the temporal variation of exposure. For the case of the two evacuation scenarios described above, exposure was assessed based on the distance from the La Fossa volcano and was found to be higher for the staged evacuation (Fig. 6d) during both seasons, with an increasing difference over time during the high season (Fig. 7d).
Some assumptions have been made to carry out our evacuation simulations that should be mentioned: (i) the evacuation starts before the eruption (so evacuation operations are not disrupted by volcanic hazards); (ii) people are not allowed to return to the island after the alarm has been issued; (iii) people are only allowed to evacuate by foot for the sake of these simulations (however, a combination of evacuation strategies can also be considered in the future, both by foot and motorized vehicles); (iv) people with disabilities are considered in the simulations by using a low walking speed (however, other considerations could be made in order to improve the analysis, e.g. integrating evacuation with dedicated motorized vehicles); (v) people might be able to take small pets with them; (vi) animals for farming activities (e.g. goats and cows) are not considered here but represent a critical aspect for an island such as Vulcano; (vii) evacuation is carried out from the three ports available on the island (i.e. Porto Ponente, Porto Levante and Porto Gelso), even though the only port that can be accessible by large ferries is Porto Levante (more studies should be carried out based on the actual evacuation capacities of Porto Ponente and Porto Gelso and in various weather and marine conditions); and (viii) people follow the instructions provided in the evacuation orders (this is particularly important for staged evacuation, as people in each community are asked to evacuate according to their turn; the possibility of having a fraction of the population not following the order of staged evacuation can be included in the simulations in order to add a level of uncertainty). In addition, while we did not directly include social-vulnerability aspects due to small community size and a lack of up-to-date data, the current evacuation simulation tool can be enhanced to include social vulnerabilities, especially if used in larger and more complex social systems. The simulation can be parameterized based on more granular detail on socio-demographic characteristics of the agent population. This will allow for the inclusion of social-vulnerability factors related to age, health conditions, gender, language, education, and access to resources and information in the evacuation simulation tool. Finally, it is important to consider and discuss some stochasticity and uncertainty aspects of the proposed evacuation simulation tool. Given that most of the distributions we have used to describe the various evacuation parameters are uniform, the stochasticity and uncertainty are relatively low, and the different simulations do not produce significantly different results. The main source of uncertainty in our model is related to the random distribution of the population and capacity of the ferries. However, more parameters can be varied in order to explore a wider range of conditions.
6.2 Assessment of the economic impact of an evacuation of the island of Vulcano
The loss of revenue due to touristic-business interruption associated with an evacuation of the island of Vulcano is studied as a function of time, in order to investigate the influence of different touristic seasons, and as a function of space, in order to investigate how a partial evacuation affects economic loss on the island. According to our results, both the time when the evacuation process is carried out and the duration of the evacuation period have significant impact on tourism. For instance, a short-term evacuation (i.e. up to 3 months) during the low season (e.g. November to January) causes about EUR 0.5 million of revenue loss. Should the island be evacuated for 6 months, the loss could increase up to about EUR 2.5 million due to an overlap with the beginning of the middle season when touristic activities start to resume; 1 year of total evacuation on the island causes about EUR 34 million of revenue loss. Only 2 % of this loss results from evacuation during the low season (about EUR 0.5 million). This is due to the fact that there are no tourists on the island during these months, and most touristic activities stop. The situation is, therefore, critical if the evacuation needs to be carried out towards the end of the middle season (e.g. June) and/or during the high season when the population on the island reaches its peak. In such a case, a month-long evacuation in June is about EUR 1 million higher than 3 months of evacuation during the low season (i.e. November to January). After that, a rapid increase in revenue loss is observed on the island: an evacuation period of 3 months starting in June causes up to EUR 26 million of revenue loss, which corresponds to 78 % of the 1-year loss because it includes the high season. It should also be considered that an evacuation during the low season could affect or also compromise the high season, due to the typical maintenance works of the touristic infrastructures performed during the low season and the impact on preparation activities (e.g. hotel booking). However, eruptions also attract tourists, as recently shown by the 2021 crises of Cumbre Vieja (La Palma, Spain) and Fagradalsfjall (Iceland). As a result, the overall impact on the high-season revenue of an evacuation during the low season due to an eruption of La Fossa would be difficult to forecast. Finally, in addition to the high revenue loss that could occur during the high season, it is important to note that the evacuation process becomes more complicated due to the high number of tourists between June and September (in addition to the diversity of languages represented by international tourists and workers), whereas an evacuation between November and April would mostly concern residents.
The loss of revenue on the island is also considered a function of space. To do this, partial evacuations including only Porto or only Vulcanello are evaluated. The main reason for assessing the partial evacuation is to be able to maintain at least some activities on the island, without interrupting all tourism-dependent businesses, and also to see which part of the island has the highest impact on the economy. According to our results, during the low season the loss of evacuating Vulcanello is slightly higher than the loss of evacuating Porto (< EUR 1 million). Although most of the touristic facilities and all the restaurants are located in Porto, the largest hotels on the island are all situated in Vulcanello, and they are open for the whole year (Therasia Resort Sea & Spa and Jera' Aloe Resort B&B). However, with the beginning of the middle season the revenue loss in Porto exceeds that in Vulcanello. If the evacuation includes July and August, the loss resulting from evacuating Porto is more than double the loss of evacuating Vulcanello.
Piano is not considered in the partial evacuation scenarios; in fact, on this southern side of the island, there are no shops, hotels or any other leisure activities to attract tourists with the exception of a famous lookout (Capo Grillo) and small beaches. Only two B&Bs and two restaurants are located in Piano with negligible revenue compared to those located in Porto and Vulcanello. However, this does not mean that Piano has no effect on Vulcano's economy. As mentioned earlier, the wine factory is situated between Piano and Gelso. According to the owner, the vineyard flourishes between March and July. Thus, if an eruption occurs during this period and the area is evacuated, there would be at least EUR 60 000 of loss generating from Piano. In addition, the critical infrastructure that is not considered in the cost assessment of this study, such as the solar plant located in Piano, may cause problems for other businesses. For example, if the electricity is cut on the island, the restaurants and hotels cannot function, and this directly affects the tourism and thus the revenues, even though the evacuation is partial and that part of the island is not affected.
Cost assessments are also required to conduct cost–benefit analyses of different mitigation measures. Although evacuating the island will cause an economic loss (i.e. the loss of revenue as the cost), it is a key measure to reduce the impact on public health. It helps ensure the prevention of eruption-related injuries and deaths, hence the main components of benefits. Quantifying the value of life is an ethical issue. Although there are studies that try to assign a value to a human life (e.g. Cropper and Sahin, 2009), here we do not consider it, as this is beyond the scope of our analysis. In any case, if an eruption on the island is imminent, total and/or partial evacuations will be conducted regardless of the cost in order to avoid casualties. However, it is important to evaluate the socio-economic impact on affected communities for authorities, in order to help them to implement informed decisions.
Although this study has provided some significant findings on the tourist sector of the economic system of the island of Vulcano, such as the main income activities and the possible loss in the case of an evacuation, it does not provide a complete picture for the cost assessments, and some important caveats need to be discussed. The first and the most important limitation concerns the lack of data. Although the municipality of Lipari was visited in May 2014, no access was granted for any official data concerning the economic situation of the Aeolian Islands, let alone Vulcano itself. All the data used to calculate the revenue on the island were based on our field visit in May 2014; official websites and various booking websites were used to complete the dataset. Another important point to mention is that the main focus of our study is on the revenue originating only from tourism-related businesses, and, therefore, the total cost of the evacuation process is not investigated (i.e. cost of evacuation operations and of relocating people). This loss presents only one part of the total cost associated with an evacuation. An extensive cost assessment requires the consideration of all different types of costs involved with the evacuation process.
6.3 Recent unrest on Vulcano and implications for crisis management
Starting from August–September 2021, analysis of the monitoring data at the island of Vulcano showed an increase in the concentration, flux and temperature of volcanic fumaroles at the crater area; an expansion of the emission areas; and an increase in low-energy seismicity. Satellite and ground deformation analysis also showed an uplift in the summit area of the volcano of about 1 cm. These phenomena caused the Italian Civil Protection Department to raise the alert level on 1 October 2021 from green (quiescence) to yellow (minor shallow hydrothermal crisis). Resulting operational actions carried out on the island as a result of this change in alert level included (i) the strengthening of the monitoring activities, (ii) constant communication between the scientific community and the operational structures of the Italian Civil Protection Department, and (iii) an update of the civil-protection plans at different territorial levels (municipal, regional and national).
At local level, the Mayor of Lipari formalized a first draft of the new municipal civil-protection plan for volcanic risk on the island of Vulcano in November 2021. Moreover, due to the increase in gas emissions from the ground, which represents a hazardous event with a local impact, overnight stays in Vulcano Porto (Porto) and adjacent areas were prohibited, through the issuing of a special order, as of 22 November 2021. Non-residents were also banned from entering the island, and the use of self-protection measures was recommended (e.g. ventilating buildings, limiting use of basements, avoiding areas of gas emissions, monitoring of health conditions and paying attention to communication from the municipality). Since mid-December 2021, the monitoring parameters have shown a gradual stabilization at high values so that some mitigation measures at the local level have been lessened through the issuing of a specific order by the mayor of Lipari. On 24 December 2021 residents were allowed back to the Porto area also at night, with the exclusion of vulnerable people (citizens with reduced mobility and/or respiratory diseases), and gas concentration values are constantly monitored in order to inform the population. On 1 February 2022 also non-residents were allowed back to the island, even though some critical restrictions remain (e.g. no access to potentially hazardous areas such as the La Fossa volcano and the beach at Porto Levante and excluding vulnerable people from sleeping in the whole of the Porto area).
At the national level, the decree of the head of the Italian Civil Protection Department of 6 December 2021 formalized a technical group for environmental monitoring of volcanic gas and air quality to complement routine volcanic monitoring, which includes representatives of public-health, environment, volcanology and civil-protection organizations. On 7 December 2021, the national civil-protection plan for volcanic risk on the island of Vulcano was formalized. The plan, compiled by the Italian Civil Protection Department in agreement with the regional Civil Protection Department of Sicily, the prefecture territorial office of the government of Messina and the municipality of Lipari, defines the mitigation actions that all the stakeholders involved in the emergency management must take and foresees the evacuation of the population before the eruption onset in the case that increasing volcanic unrest is observed. The results of the work presented in this paper as well as the insights into risk assessment presented in Bonadonna et al. (2021) were used to finalize the emergency plan at the national level. However, given that the recent unrest was mostly related to gas hazard and was, therefore, managed at the local level (i.e. by the municipality) and that the overnight restrictions issued by the municipality did not involve an evacuation of the island, the results of these two analyses could be neither tested nor validated.
Evacuation is often the only strategy to save lives in the case of extreme volcanic activity and rapidly escalating unrest. This is especially critical for the La Fossa volcano, whose activity has been characterized by hydrothermal events, which are typically very sudden and unpredictable, and magmatic events with little warning signals (e.g. 1888–1890 vulcanian cycle). In such a case and considering the high level of population exposure to dangerous hazards, evacuation might be considered even in the case of weak unrest. Nonetheless, the timing and routing of evacuation is critical to remove people from hazard zones before they are impacted.
The Vulcano Evacuation Simulation Tool described here has been developed to test the effectiveness of ABM simulation in evacuation planning for areas subject to volcanic hazards on a small island. Based on a pre-eruption simulation at Vulcano, both the simultaneous and the staged evacuation are slightly faster during the low touristic season (∼ 6.7 and ∼ 7.1 h to evacuate 1000 people, respectively; Fig. 6a, b) with respect to the high touristic season (∼ 8.9 h to evacuate 4600 people; Fig. 7a, b). Nonetheless, we have also shown that the type of evacuation (i.e. staged or simultaneous) can optimize the number of people evacuated in time, with the simultaneous evacuation being more efficient at removing people from the island than the staged evacuation. In fact, after 5 h (300 min), during the low season, about 84 % and 72 % of people would be evacuated with a simultaneous and a staged evacuation, respectively, while after ∼ 6 h (370 min), during the high season, about 96 % and 86 % would be evacuated with a simultaneous and a staged evacuation, respectively (Figs. 6c and 7c). Additional analyses should be carried out to explore more evacuation conditions (e.g. evacuation by car, evacuation from fewer ports and evacuation after the onset of the eruption) and the role of social vulnerability. In fact, the proposed evacuation simulation tool can be used to model varying impacts for different scenarios to enable the proper allocation of resources required for evacuations and economic support of the affected areas.
We have also shown how, in an island like Vulcano whose economy is based on tourism, the timing and duration of evacuation can have a very different impact. In fact, if the evacuation of the whole island starts at the beginning of the low touristic season (e.g. November), the impact becomes significant only if it lasts more than 6 months (> 7 % of annual total turnover), whereas if it starts in June (i.e. at the end of the middle season and approaching the high touristic season in July–August), the impact becomes significant after 1 month (> 5 % of annual total turnover) and reaches 78 % of the annual total turnover after 3 months. Our results also show that a total evacuation starting in June of various durations results in 88 %–98 % more revenue loss than an evacuation starting in November. This is directly related to the large number of tourists on the island during the high season (July–August). In addition, an evacuation of up to 3 months in the low season would not produce a large difference in revenue loss between the partial evacuations of Porto and of Vulcanello in terms of revenue loss. On the contrary, an evacuation of Porto of 1 year would cause 60 % higher revenue loss than evacuating Vulcanello. This is due to the fact that all leisure activities and restaurants with the majority of hotels, hostels and B&Bs are located in Porto. Regardless of the beginning of the evacuation and associated duration, the total evacuation of the island would generate 28 %–53 % more revenue loss than the partial evacuation of Porto and 47 %–73 % more revenue loss than the partial evacuation of Vulcanello. It is important to stress that, during crises, human life is prioritized over economic losses, whatever the situation. However, our analysis provides insights into the potential economic impact, which could be mitigated if foreseen and integrated into an emergency plan.
An example of a pedestrian evacuation simulation run for a simultaneous evacuation scenario during the high season on Vulcano (4600 people consisting of 400 people in Piano, 400 people in Vulcanello and 3800 people in Porto; see main text for details) is provided (https://doi.org/10.5446/56499) (Bonadonna, 2022). The simulation shows movement of evacuees from different parts of the island to their designated or nearest active port from where they will be evacuated by ferry (Porto Levante and Porto Ponente in the north and Porto Gelso in the south). Yellow colour represents people who have received the evacuation order; orange colour shows people who are preparing for evacuation; and brown colour shows people who are moving towards the ports. Bar at the bottom shows the number of ports and ferries used (in this case three ports on Vulcano and one port in Milazzo where ferries bring the evacuated people), the number of people at a given time step and the evacuation time indicated in minutes.
The datasets supporting the conclusions of this article are included within the article (see Tables 2–7).
CB, AA, FR, TZ and CF conceived and wrote the first draft of the paper. AA developed the ABM tool and performed the simulations for different evacuation scenarios based on information provided by CB and CF. TZ carried out the data collection and analysis necessary for the assessment of economic impact of an evacuation of Vulcano under the supervision of FR, CB and CF. CC, MR, CEG, SB, SM, MP and AR provided key insights into the interpretation of the results. All authors contributed to the finalization of the paper.
The contact author has declared that neither they nor their co-authors have any competing interests.
The information set out in this publication reflects the authors' views.
Publisher’s note: Copernicus Publications remains neutral with regard to jurisdictional claims in published maps and institutional affiliations.
The authors are grateful to the colleagues at the Dipartimento della Protezione Civile of Italy and to the participants of the CERG-C programme for fruitful discussions that helped shape the evacuation analysis presented in this paper. They also thank the population of the island of Vulcano for their support of the CERG-C programme over the years. The editor and two anonymous reviewers are thanked for their constructive comments that helped improve the paper.
This research has been supported by the Swiss National Science Foundation (project nos. 200021-129997 and IZSEZ0_181030), the CERG-C programme of the University of Geneva and the Ontario Research Fund (ADERSIM).
This paper was edited by Giovanni Macedonio and reviewed by two anonymous referees.
Abdelgawad, H. and Abdulhai, B.: Emergency evacuation planning as a network design problem: a critical review, Transp. Lett., 1, 41–58, 2009.
Alvarado, G. E., Mele, D., Dellino, P., de Moor, J. M., and Avard, G.: Are the ashes from the latest eruptions (2010–2016) at Turrialba volcano related to phreatic or phreatomagmatic events?, J. Volcanol. Geoth. Res., 327, 407–415, 2016.
Aretano, R., Petrosillo, I., Zaccarelli, N., Semeraro, T., and Zurlini, G.: People perception of landscape change effects on ecosystem services in small Mediterranean islands: A combination of subjective and objective assessments, Landscape Urban Plan., 112, 63–73, 2013.
Asgary, A. and Yang, P.: Simulating campus evacuation: case of york university, Society for Computer Simulation International, Proceedings of the Summer Computer Simulation Conference, 24–27 July 2016, Montréal, Québec, CA, 1–6pp., 2016.
Auker, M. R., Sparks, R. S. J., Siebert, L., Crosweller, H. S., and Ewert, J.: A statistical analysis of the global historical volcanic fatalities record, Journal of Applied Volcanology, 2, 2, https://doi.org/10.1186/2191-5040-2-2, 2013.
Bae, J. W., Lee, S., Hong, J. H., and Moon, I. C.: Simulation-based analyses of an evacuation from a metropolis during a bombardment, Simulation, 90, 1244–1267, 2014.
Barberi, F., Bertagnini, A., Landi, P., and Principe, C.: A review on phreatic eruptions and their precursors, J. Volcanol. Geoth. Res., 52, 231–246, 1992.
Barclay, J., Few, R., Armijos, M. T., Phillips, J. C., Pyle, D. M., Hicks, A., Brown, S. K., and Robertson, R. E. A.: Livelihoods, Wellbeing and the Risk to Life During Volcanic Eruptions, Front. Earth Sci., 7, 205, https://doi.org/10.3389/feart.2019.00205, 2019.
Baxter, P., Neri, A., and Todesco, M.: Physical modelling and human survival in pyroclastic flows, Nat. Hazards, 17, 163–176, 1998.
Bebbington, M. and Zitikis, R.: Dynamic Uncertainty in Cost-Benefit Analysis of Evacuation Prior to a Volcanic Eruption, Math. Geosci., 48, 123–148, 2016.
Bernardini, G., Camilli, S., Quagliarini, E., and D'Orazio, M.: Flooding risk in existing urban environment: From human behavioral patterns to a microscopic simulation model, in: Sustainability in Energy and Buildings 2017: Proceedings of the Ninth KES International Conference, 5–7 July 2017, Chania, Greece, 134, 131–140, 2017.
Biass, S., Bonadonna, C., Di Traglia, F., Pistolesi, M., Rosi, M., and Lestuzzi, P.: Probabilistic evaluation of the physical impact of future tephra fallout events for the Island of Vulcano, Italy, B. Volcanol., 78, 37, https://doi.org/10.1007/s00445-016-1028-1, 2016a.
Biass, S., Falcone, J.L., Bonadonna, C., Di Traglia, F., Pistolesi, M., Rosi, M., and Lestuzzi, P.: Great Balls of Fire: A probabilistic approach to quantify the hazard related to ballistics – A case study at La Fossa volcano, Vulcano Island, Italy, J. Volcanol. Geoth. Res., 325, 1–14, 2016b.
Bird, D. K., Gisladottir, G., and Dominey-Howes, D.: Volcanic risk and tourism in southern Iceland: Implications for hazard, risk and emergency response education and training, J. Volcanol. Geoth. Res., 189, 33-48, 2010.
Bonadonna, C.: Example of a pedestrian evacuation simulation run (Vulcano island, Italy), TIB AV-Portal [video], https://doi.org/10.5446/56499, 2022.
Bonadonna, C., Frischknecht, C., Menoni, S., Romerio, F., Gregg, C. E., Rosi, M., Biass, S., Asgary, A., Pistolesi, M., Guobadia, D., Gattuso, A., Ricciardi, A., and Cristiani, C.: Integrating hazard, exposure, vulnerability and resilience for risk and emergency management in a volcanic context: the ADVISE model, Journal of Applied Volcanology, 10, 7, https://doi.org/10.1186/s13617-021-00108-5, 2021.
Bouwer, L. M., Poussin, J., Papyrakis, E., Daniel, V., Pfurtscheller, C., Thieken, A. H., and Aerts, J. C. J. H.: Methodology report on costs of mitigation, CONHAZ Report, https://www.ufz.de/index.php?en=35939 (last access: 12 July 2021), 2011.
Breard, E. C. P., Lube, G., Cronin, S. J., and Valentine, G. A.: Transport and deposition processes of the hydrothermal blast of the 6 August 2012 Te Maari eruption, Mt. Tongariro, B. Volcanol., 77, 100, https://doi.org/10.1007/s00445-015-0980-5, 2015.
Brown, D. and Xie, Y.: Spatial agent-based modelling, Int. J. Geogr. Inf. Sci., 20, 941-943, 2006.
Brown, S. K., Jenkins, S. F., Sparks, R. S. J., Odbert, H., and Auker, M. R.: Volcanic fatalities database: Analysis of volcanic threat with distance and victim classification, Journal of Applied Volcanology, 6, 15, https://doi.org/10.1186/s13617-017-0067-4, 2017.
Chastin, S. F. M. and Main, I. G.: Statistical analysis of daily seismic event rate as a precursor to volcanic eruptions, Geophys. Res. Lett., 30, 1671, https://doi.org/10.1029/2003GL016900, 2003.
Chen, X. and Zhan, F. B.: Agent-based modeling and simulation of urban evacuation: relative effectiveness of simultaneous and staged evacuation strategies, J. Oper. Res. Soc., 59, 25–33, https://doi.org/10.1057/palgrave.jors.2602321, 2008.
Cheng, J. C., Tan, Y., Song, Y., Mei, Z., Gan, V. J., and Wang, X.: Developing an evacuation evaluation model for offshore oil and gas platforms using BIM and agent-based model, Automat. Constr., 89, 214–224, 2018.
Chien, S. I. and Korikanthimath, V. V.: Analysis and Modeling of Simultaneous and Staged Emergency Evacuations, J. Transp. Eng., 133, 190–197, 2007.
Codice della protezione Civile: Decreto Legislativo n.1 del 2 gennaio 2018, Gazzetta Ufficiale n. 17 del 22 gennaio 2018, [Legislative decree: civil protection code], https://www.protezionecivile.gov.it/en/normativa/decreto-legislativo-n-1-del-2-gennaio-2018–codice-della-protezione-civile (last access: 26 March 2022), 2018.
Cole, J. W., Sabel, C. E., Blumenthal, E., Finnis, K., Dantas, A., Barnard, S., and Johnston, D.: GIS-based emergency and evacuation planning for volcanic hazards in New Zealand, Bulletin of the New Zealand Society for Earthquake Engineering, 38, 149–164, 2005.
Connor, C. B., Sparks, R. S. J., Mason, R. M., Bonadonna, C., and Young, S. R.: Exploring links between physical and probabilistic models of volcanic eruptions: The Soufrière Hills Volcano, Montserrat, Geophys. Res. Lett., 30, 1701, https://doi.org/10.1029/2003GL017384, 2003.
Cornelius, R. R. and Voight, B.: Seismological aspects of the 1989–1990 eruption at Redoubt Volcano, Alaska: The Materials Failure Forecast Method (FFM) with RSAM and SSAM seismic data, J. Volcanol. Geoth. Res., 62, 469–498, 1994.
Cropper, M. L. and Sahin, S.: Valuing mortality and morbidity in the context of disaster risks, Policy research working paper, The World Bank, WPS 4832, https://openknowledge.worldbank.org/handle/10986/4041 (last access: 26 March 2022), 2009.
De la Cruz-Reyna, S. and Reyes-Davila, G. A.: A model to describe precursory material-failure phenomena: applications to short-term forecasting at Colima volcano, Mexico, Bull. Volcanol., 63, 297–308, 2001.
de Moor, J. M., Aiuppa, A., Pacheco, J., Avard, G., Kern, C., Liuzzo, M., Martínez, M., Giudice, G., and Fischer, T. P.: Short-period volcanic gas precursors to phreatic eruptions: insights from Poás volcano, Costa Rica, Earth Planet Sci. Lett., 442, 218–227, https://doi.org/10.1016/j.epsl.2016.02.056, 2016.
De Astis, G., Lucchi, F., Dellino, P., La Volpe, L., Tranne, C. A., Frezzotti, M. L., and Peccerillo, A.: Geology, volcanic history and petrology of Vulcano (central Aeolian archipelago), Geol. Soc. Lond. Memoirs, 37, 281–349, https://doi.org/10.1144/M37.11, 2013.
Dellino, P., De Astis, G., La Volpe, L., Mele, D., and Sulpizio, R.: Quantitative hazard assessment of phreatomagmatic eruptions at Vulcano (Aeolian Islands, Southern Italy) as obtained by combining stratigraphy, event statistics and physical modelling, J. Volcanol. Geoth. Res., 201, 364–384, 2011.
Di Traglia, F., Pistolesi, M., Rosi, M., and Bonadonna, C.: Growth and erosion: the volcanic geology and the morphological evolution during the last 1000 years of the eruptive activity at the La Fossa cone volcano (Island of Vulcano, Southern Italy), Geomorphology, 94–107, https://doi.org/10.1016/j.geomorph.2013.04.018, 2013.
Dóniz-Páez, J., Beltrán-Yanes, E., Becerra-Ramírez, R., Pérez, N. M., Hernández, P. A., and Hernández, W.: Diversity of Volcanic Geoheritage in the Canary Islands, Spain, Geosciences, 10, 390, https://doi.org/10.3390/geosciences10100390, 2020.
Doyle, E. H., McClure, J., Paton, D., and Johnston, D. M.: Uncertainty and decision making: Volcanic crisis scenarios, Int. J. Disast. Risk Re., 10, 75–101, 2014.
Erfurt-Cooper, P.: Geotourism in volcanic and geothermal environments: playing with fire?, Geoheritage, 3, 187–193, 2011.
Erfurt-Cooper, P. and Cooper, M. (Eds.): Volcano and geothermal tourism: sustainable geo-resources for leisure and recreation, Earthscan, 3–32, 2010.
Fahad, M. G. R., Nazari, R., Bhavsar, P., Jalayer, M., and Karimi, M.: A decision-support framework for emergency evacuation planning during extreme storm events, Transport. Res. D-Tr. E., 77, 589–605, 2019.
Faraone, D., Silvano, A., and Verdiani, G.: The monzogabbroic intrusion in the island of Vulcano, Aeolian Archipelago, Italy, B. Volcanol., 48, 299–307, https://doi.org/10.1007/BF01081758, 1986.
Farrell, J.: Alternatives to road building to improve hurricane evacuation in coastal South Carolina, Spring 2005 environmental advocacy seminar, School of Law, University of South Carolina, Columbia, SC, 2005.
Fearnley, C. J.: Assigning a volcano alert level: negotiating uncertainty, risk, and complexity in decision-making processes, Environ. Plann. A, 45, 1891–1911, 2013.
Galderisi, A., Bonadonna, C., Delmonaco, G., Ferrara, F. F., Menoni, S., Ceudech, A., Biass, S., Frischknecht, C., Manzella, I., Minucci, G., and Gregg, C.: Vulnerability Assessment and Risk Mitigation: The Case of Vulcano Island, Italy, in: Landslide Science and Practice, edited by: Margottini, C., Canuti, P., and Sassa, K., Springer, Berlin, Heidelberg, https://doi.org/10.1007/978-3-642-31313-4_8, 2013.
Gattuso, A., Bonadonna, C., Frischknecht, C., Cuomo, S., Baumann, V., Pistolesi, M., Biass, S., Arrowsmith, R., Moscariello, M., and Rosi, M.: Lahar risk assessment from source identification to potential impact analysis: the case of Vulcano Island, Italy, Journal of Applied Volcanology, 10, 9, https://doi.org/10.1186/s13617-021-00107-6, 2021.
Gaudard, L. and Romerio, F.: Natural hazard risk in the case of an emergency: The real options approach, Nat. Hazards, 75, 473–488, 2015.
Gilbert, N. and Bankes, S.: Platforms and methods for agent-based modeling, Proc. Natl. Acad. Sci. USA, 99, 7197–7198, 2002.
Gioncada, A., Sbrana, A., and Teklemariam, M.: Hydrothermal alteration and fluid inclusion studies in the Vulcano geothermal wells (Italy), Proceeding of the World Geothermal Congress, Florence, Italy, 18–31 May 1995, 1099–1104, 1995.
Gregg, C. E., Houghton, B. F., and Ewert, J.: Volcano warning systems, in: Encyclodpedia of Volcanoes, second edn., edited by: Sigurdson H., Houghton, B., McNutt, S., Rymer, H., and Stix, J., Elsevier, 1173–1185, 2015.
Han, L. D, Yuan, F., and Urbanik II, T.: What Is an Effective Evacuation Operation?, J. Urban Plan. D., 133, 1, https://doi.org/10.1061/(ASCE)0733-9488(2007)133:1(3), 2007.
Hastings, P.: Eruption Alert at Rabaul Caldera, in: Fire mountains of the island: A history of volcanic eruptions and disaster management in Papua New Guinea and the Solomon Islands, edited by: Johnson, R. W., Australian National University, 12, 257–275, 2013.
Heggie, T. W.: Geotourism and volcanoes: health hazards facing tourists at volcanic and geothermal destinations, Travel Med. Infect. Di., 7, 257–261, 2009.
Hilljegerdes, M. and Augustijn-Beckers, E. W.: Evaluating the effects of consecutive hurricane hits on evacuation patterns in Dominica, 16th International Conference on Information Systems for Crisis Response and Management 2019, Valencia, Spain, 19–22 May 2019, 462–472, http://idl.iscram.org/files/martinhilljegerdes1/2019/1734_MartinHilljegerdes1+Ellen-WienAugustijn-Beckers22019.pdf (last access: 26 March 2022), 2019.
Ho, C. H.: Predictions of volcanic eruptions at Mt Vesuvius, Italy, J. Geodyn., 15, 13–18, 1992.
International Federation of Red Cross and Red Crescent Societies (IFRC): 12-month update Guatemala: volcanic eruption (Fuego volcano), Report MDRGT013, https://reliefweb.int/sites/reliefweb.int/files/resources/MDRGT01312m.pdf (last access: 26 March 2022), 2019.
Joo, J., Kim, N., Wysk, R. A., Rothrock, L., Son, Y.-J., Oh, Y.-g., and Lee, S.: Agent-based simulation of affordance-based human behaviors in emergency evacuation, Simul. Model. Pract. Th., 32, 99–115, 2013.
Jumadi, J., Carver, S., and Quincey, D.: ABM and GIS-based multi-scenarios volcanic evacuation modelling of Merapi, AIP Conf. Proc., 1730, 050005, https://doi.org/10.1063/1.4947401, 2016a.
Jumadi, J., Carver, S., and Quincey, D.: A conceptual framework of volcanic evacuation simulation of Merapi using agent-based model and GIS, Procd. Soc. Behv., 227, 402–409, 2016b.
Jumadi, J., Carver, S. J., and Quincey, D. J.: An Agent-Based Evaluation of Varying Evacuation Scenarios in Merapi: Simultaneous and Staged, Geosciences, 9, 317, https://doi.org/10.3390/geosciences9070317, 2019.
Lane, L. R., Tobin, G. A., and Whiteford, L. M.: Volcanic hazard or economic destitution: hard choices in Baños, Ecuador, Global Environmental Change Part B: Environmental Hazards, 5, 23–34, 2003.
Lechner, H. N. and Rouleau, M. D.: Should we stay or should we go now? Factors affecting evacuation decisions at Pacaya volcano, Guatemala, Int. J. Disast. Risk Re., 40, 101160, https://doi.org/10.1016/j.ijdrr.2019.101160, 2019.
Leone, F., Komorowski, J., Gherardi-Leone, M., and Lalubie G.: Integrating spatial accessibility in the design of volcano evacuation plans in the French West Indies (Guadeloupe and Martinique), Journal of Applied Volcanology, 8, 8, https://doi.org/10.1186/s13617-019-0089-1, 2019.
Liang, W., Lam, N. S.-N., Qin, X., and Ju, W.: A Two-level Agent-Based Model for Hurricane Evacuation in New Orleans, J. Homel. Sec. Emerg., 12, 407–435, 2015.
Lindell, M., Murray-Tuite, P., Wolshon, B., and Baker, E.: Large-Scale Evacuation, Boca Raton: CRC Press, 346 pp., ISBN-10 1482259850, 2019.
Malone, S. W., Miller, C. A., and Neill, D. B.: Traffic flow models and the evacuation problem, UMAP Journal, 22, 1–47, 2001.
Marrero, J., Garcia, A., Llinares, A., De la Cruz-Reyna, S., Ramos, S., and Ortiz, R.: Virtual tools for volcanic crisis management, and evacuation decision support: Applications to El Chichón volcano (Chiapas, México), Nat. Hazards, 68, 955–980, 2013.
Marzocchi, W. and Woo, G.: Principles of volcanic risk metrics: Theory and the case study of Mount Vesuvius and Campi Flegrei, Italy, J. Geophys. Res., 114, 1–12, 2009.
Mas, E., Suppasri, A., Imamura, F., and Koshimura, S.: Agent-based Simulation of the 2011 Great Japan Earthquake/Tsunami Evacuation: An integrated model of Tsunami inundation and evacuation, Natural Disaster Science, 34, 41–57, 2012.
Mas, E., Koshimura, S., Imamura, F., Suppasri, A., Muhari, A., and Adriano, B.: Recent advances in agent-based tsunami evacuation simulations: Case studies in Indonesia, Thailand, Japan and Peru, Pure Appl. Geophys., 172, 3409–3424, 2015.
MCDEM: Mass evacuation planning: director's guidelines for civil defence emergency management (CDEM) groups, DGL 07/08, Ministry of Civil Defence and Emergency Management, http://www.civildefence.govt.nz/memwebsite.nsf/Files/Director_Guidelines/{\$}file/Mass-evacu-planningDGL07-08.pdf (last access: 12 February 2019), 2008.
Mercalli, G. and Silvestri, O.: Le eruzioni dell'isola di Vulcano, incominciate il 3 Augusto 1888 e terminate il 22 Marzo 1890, Ann. Uffic. Cent. Meteorol. Geodin., 10, 213 pp., 1891.
Meyer, V., Becker, N., Markantonis, V., Schwarze, R., van den Bergh, J. C. J. M., Bouwer, L. M., Bubeck, P., Ciavola, P., Genovese, E., Green, C., Hallegatte, S., Kreibich, H., Lequeux, Q., Logar, I., Papyrakis, E., Pfurtscheller, C., Poussin, J., Przyluski, V., Thieken, A. H., and Viavattene, C.: Review article: Assessing the costs of natural hazards – state of the art and knowledge gaps, Nat. Hazards Earth Syst. Sci., 13, 1351–1373, https://doi.org/10.5194/nhess-13-1351-2013, 2013.
Moriarty, K., Ni, D., and Collura, J.: Modelling traffic flow under emergency evacuation situations: current practices and future directions, 86th Transportation Research Board Annual Meeting, Transportation Research Board, Washington, D.C., USA, 21–25 January 2007.
Oikawa, T., Yoshimoto, M., Nakada, S., Maeno, F., Komori, J., Shimano, T., Takeshita, Y., Ishizuka, Y., and Ishimine, Y.: Reconstruction of the 2014 eruption sequence of Ontake Volcano from recorded images and interviews, Earth Planets Space, 68, 79, https://doi.org/10.1186/s40623-016-0458-5, 2016.
Peers, J. B., Gregg, C. E., Lindell, M. K., Pelletier, D., Romerio, F., and Joyner, A. T.: The Economic Effects of Volcanic Alerts–A Case Study of High-Threat U.S. Volcanoes, Risk Anal., 41, 1759–1781, https://doi.org/10.1111/risa.13702, 2021.
Pistolesi, M., Rosi, M., Malaguti, A. B., Lucchi, F., Tranne, C. A., Speranza, F., Albert, P. G., Smith, V., Di Roberto, A., and Billotta, E.: Chrono- stratigraphy of the youngest (last 1500 years) rhyolitic eruptions of Lipari (Aeolian Islands, Southern Italy) and implications for distal tephra correlations, J. Volcanol. Geoth. Res., 420, 107397, https://doi.org/10.1016/j.jvolgeores.2021.107397, 2021.
Pluchino, S., Tribulato, C., Caverzan, A., Mc Quillan, A., Cimellaro, G., and Mahin, S.: Agent-Based Model for Pedestrians' Evacuation after a Blast Integrated with a Human Behavior Model, Structures Congress, Resten: ASCE, 1506–1518, 2015.
Quesada-Román, A. and Pérez-Umaña, D.: State of the Art of Geodiversity, Geoconservation, and Geotourism in Costa Rica, Geosciences, 10, 211, https://doi.org/10.3390/geosciences10060211, 2020.
Rosi, M., Di Traglia, F., Pistolesi, M., Esposti Ongaro T., de' Michieli Vitturi, M., and Bonadonna, C.: Dynamics of shallow hydrothermal eruptions: new insights from Vulcano's Breccia di Commenda eruption, B. Volcanol., 80, 83, https://doi.org/10.1007/s00445-018-1252-y, 2018.
Rouwet, D., Sandri, L., Marzocchi, W., Gottsmann, J., Selva, J., Tonini, R., and Papale, P.: Recognizing and tracking volcanic hazards related to non-magmatic unrest: a review, Journal of Applied Volcanology, 3, 17, https://doi.org/10.1186/s13617-014-0017-3, 2014.
Sbayti, H. and Mahmassani, H. S.: Optimal Scheduling of Evacuation Operations, in: Transportation Research Record: Journal of the Transportation Research Board, No. 1964, Transportation Research Board of the National Academies, Washington, D.C., USA, 238–246, 2006.
Selva, J., Bonadonna, C., Branca, S., De Astis, G., Gambino, S., Paonita, A., Pistolesi, M., Ricci, T., Sulpizio, R., Tibaldi, A., and Ricciardi, A.: Multiple hazards and paths to eruptions: A review of the volcanic system of Vulcano (Aeolian Islands, Italy), Earth-Sci. Rev., 207, 103186, https://doi.org/10.1016/j.earscirev.2020.103186, 2020.
Selva, J., Lorito, S., Volpe, M., Romano, F., Tonini, R., Perfetti, P., Bernardi, F., Taroni, M., Scala, A., Babeyko, A., Løvholt, F., Gibbons, S. J., Macías, J., Castro, M. J., González-Vida, J. M., Sánchez-Linares, C., Bayraktar, H. B., Basili, R., Maesano, F. E., Tiberti, M. M., Mele, F., Piatanesi, A., and Amato, A.: Probabilistic tsunami forecasting for early warning, Nat. Commun., 12, 5677, https://doi.org/10.1038/s41467-021-25815-w, 2021.
Sorensen, J. H. and Mileti, D. S.: Protective action initiation time estimation for dam breaches, controlled dam releases, and levee breaches or overtopping, US Army Corps of Engineers, Institute for Water Resources, Risk Management Center (CEIWR-RMC), 2014.
Sorensen, J. H. and Sorensen, B. V.: Community Processes: Warning and Evacuation, in: Handbook of Disaster Research, Handbooks of Sociology and Social Research, Springer, New York, NY, 183–199, https://doi.org/10.1007/978-0-387-32353-4_11, 2007.
Sparks, R. S. J.: Forecasting volcanic eruptions, Earth Planet. Sc. Lett. 210, 1–15, 2003.
Stepanov, A. and Smith, J. M.: Multi-objective evacuation routing in transportation networks, Eur. J. Oper. Res., 198, 435–446, 2009.
Stix, J. and de Moor, J. M.: Understanding and forecasting phreatic eruptions driven by magmatic degassing, Earth Planets Space, 70, 83, https://doi.org/10.1186/s40623-018-0855-z, 2018.
The Economist: The next big wave, 16 August 2003 edn., https://www.economist.com/science-and-technology/2003/08/14/the-next-big-wave (last access: 2 October 2021), 2003.
Thompson, R. R., Garfin, D. R., and Silver, R. C.: Evacuation from natural disasters: a systematic review of the literature, Risk Anal., 37, 812–839, 2017.
Tilling, R. I.: The critical role of volcano monitoring in risk reduction, Advances in Geosciences, 14, 3–11, 2008.
Tomsen, E. L., Gahegan, J. M., Wilson, M., Blake, T. M., and Daniel, M.: Evacuation planning in the Auckland Volcanic Field, New Zealand: a spatio-temporal approach for emergency, J. Appl. Volcanol., 3, 6, https://doi.org/10.1186/2191-5040-3-6, 2014.
Urbanik, T.: Evacuation time estimates for nuclear power plants, J. Hazard. Mater., 75, 165–180, 2000.
Voight, B., Constantine, E. K., Siswowidjoyo, S., and Torley, R.: Historical eruptions of Merapi Volcano, Central Java, Indonesia, 1768–1998, J. Volcanol. Geoth. Res., 100, 69–138, 2000.
Voight, B., Calvache, M. L., Hall, M. L., and Monsalve, M. L.: Nevado del Ruiz Volcano, Colombia 1985, in: Encyclopedia of Natural Hazards, edited by: Bobrowsky, P. T., Encyclopedia of Earth Sciences Series, Springer, Dordrecht, The Netherlands, https://doi.org/10.1007/978-1-4020-4399-4_253, 2013.
Wang, X., Chen, S., Zhou, Y., Peng, H., and Cui, Y.: Simulation on passenger evacuation under fire emergency in Metro station, 2013 IEEE International Conference on Intelligent Rail Transportation Proceedings, Beijing, China, 30 August–1 September 2013, 259–262, https://doi.org/10.1109/ICIRT.2013.6696304, 2013.
Wilson, T., Cole, J., Johnston, D., Cronin, S., Stewart, C., and Dantas, A.: Short-and long-term evacuation of people and livestock during a volcanic crisis: lessons from the 1991 eruption of Volcán Hudson, Chile, Journal of Applied Volcanology, 1, 1–11, 2012.
Wolshon, B., Catarella-Michel, A., and Lambert, L.: Louisiana highway evacuation plan for Hurricane Katrina: Proactive management of a regional evacuation, J. Transp. Eng. 132, 1–10, 2006.
Woo, G.: Probabilistic criteria for volcano evacuation decisions, Nat. Hazards, 45, 87–97, 2008.
Wood, N., Jones, J., Peters, J., and Richards, K.: Pedestrian evacuation modeling to reduce vehicle use for distant tsunami evacuations in Hawai'i, Int. J. Disast. Risk Re., 28, 271–283, https://doi.org/10.1016/j.ijdrr.2018.03.009, 2018.
Yang, L., Liu, Q., Yang, S., and Yu, D.: Evacuation Planning with Flood Inundation as Inputs, in: Proceedings of the ISCRAM 2015 Conference, Kristiansand, Norway, 24–27 May 2015, 24–27 pp., 2015.
You Lim, Z. and Flaherty, G.: Fiery eruptions: Travel health risks of volcano tourism, J. Travel Med., 27, 32080725, https://doi.org/10.1093/jtm/taaa019, 2020.
Zanella, E., Astis, G. D., and Lanza, R.: Paleomagnetism of weded, pyroclastic-fall scoriae at vulcano, aeolian Archipelago, J. Volcanol. Geoth. Res., 107, 71–86, 2001.
- Abstract
- Introduction
- Background on effective evacuation
- Case study: the island of Vulcano, Italy
- Methods
- Results
- Discussion
- Conclusions
- Appendix A: Vulcano Evacuation Simulation Tool settings page and interface
- Appendix B: Example of a pedestrian evacuation simulation run (video)
- Data availability
- Author contributions
- Competing interests
- Disclaimer
- Acknowledgements
- Financial support
- Review statement
- References
- Abstract
- Introduction
- Background on effective evacuation
- Case study: the island of Vulcano, Italy
- Methods
- Results
- Discussion
- Conclusions
- Appendix A: Vulcano Evacuation Simulation Tool settings page and interface
- Appendix B: Example of a pedestrian evacuation simulation run (video)
- Data availability
- Author contributions
- Competing interests
- Disclaimer
- Acknowledgements
- Financial support
- Review statement
- References