the Creative Commons Attribution 3.0 License.
the Creative Commons Attribution 3.0 License.
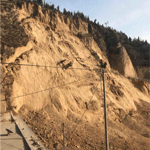
Factors influencing development of cracking–sliding failures of loess across the eastern Huangtu Plateau of China
Yanrong Li
Jiarui Mao
Xiqiong Xiang
Ping Mo
Loess is a porous, weakly cemented, and unsaturated Quaternary sediment deposited by the wind in arid and semiarid regions. Loess is widely and thickly distributed in China, making the Huangtu Plateau the largest bulk accumulation of loess on Earth. However, the fragile geoenvironment in the loess areas of China causes frequent and various geohazards, such as cracking–sliding failure (“beng-hua” in Chinese), which is a typical geohazard that causes the largest number of casualties each year. This study investigates the main influencing factors and development patterns of cracking–sliding failure of loess to help prevent its occurrence and reduce losses effectively. The following conclusions are derived from this study: (1) cracking–sliding failures mostly take place in rectilinear slopes, convex slopes, slopes with gradients greater than 60∘, slopes with heights of 5 to 40 m, and slopes mostly exposed to sunlight with aspects of 180 to 270∘; (2) cracking–sliding failures occur mostly from 10 pm to 4 am and mainly in the rainy season (July to September) and in the freeze-thaw season (March to April); and (3) highly intense human activities in the region correspond to a high possibility of cracking–sliding failures.
- Article
(2517 KB) - Full-text XML
- BibTeX
- EndNote
Any yellowish, carbonate-bearing, quartz-rich, silt-dominated strata formed by aeolian deposition and aggregated by loessification during glacial times are widely defined as loess (Richthofen, 1882; Pye, 1987). Loess (“huang-tu” in Chinese) and its related deposits are one of the most widespread Quaternary sedimentary formations, and they are most abundant in arid or semiarid regions in inner Eurasia and North America; they are characterized by high porosity, weak cementation, and unsaturation (Smalley et al., 2011).
The Huangtu (Loess) Plateau in China (LPC) is a key region for the comprehensive development of agriculture, forestry, animal husbandry, and industrial resources with an arable land area of 173 000 km2, which accounts for more than one-fifth of the entire arable land of the country and feeds more than 200 million people (Zhang, 2014). However, geohazards, such as cracking–sliding, toppling, falling, sliding, peeling, and failures of cavities built by humans, occur frequently because of fragile geological and natural environments, excessive reclamation, and unreasonable engineering activities. Among these geohazards, cracking–sliding failure, normally with a volume of several hundred cubic meters, causes the largest number of casualties in the eastern LPC (Lei, 2001). More than 1000 cracking–sliding failures were recorded in the past two decades, and they caused an average of more than 100 fatalities per year, despite the small volume of each individual failure. Unlike “flows” or “slides” as defined by Cruden and Varnes (1996), cracking–sliding failures have composite failure planes composed of two parts. The upper part normally develops vertically from the crown of the slope down for one to several meters deep. The upper part forms by tensile cracking, but the slope can stably stand for a long time despite such cracks. The lower part is generally inclined at an angle ranging from 15 to 60∘. Sliding along the lower part, which is triggered by rainfall, freezing-thawing, daily temperature fluctuations, slope undercutting, and earth tremors likely mobilizes cracking–sliding failures.
According to historical records, 62 cracking–sliding failures occurred in Shenmu, Mizhi, Zizhou, and other sites in northern Shaanxi Province from 1985 to 1993 and caused 258 deaths and more than 40 injuries (Qu et al., 2001). In 2005, the cracking–sliding failure in Jixian County in Shanxi Province resulted in 24 deaths and economic losses of approximately RMB 10 million. Failure with a volume of 2.5×104 m3 took place in Zhongyang County in Shanxi on 16 November 2009, causing 23 deaths and destroying 6 houses. In 2013, 36 loess failures were documented in Tianshui City, Gansu Province (Xin et al., 2013). In 2015, a cracking–sliding failure in Linxian County, Shanxi buried four families comprised of nine people. All of these failures developed within the loess-paleosol sequence, with relatively uniform mineralogical and chemical compositions. More recently, a cracking–sliding failure occurred in Shilou County of Shanxi Province on 10 March 2018 and destroyed 36 houses (Fig. 1). The original loess slope was characterized by slope gradient of 60∘, height of 50 m, and aspect of 280∘. The scarp of this failure was dominated by near-vertical tensile cracks with an average gradient of 85∘. The displaced mass reached 7600 m3.
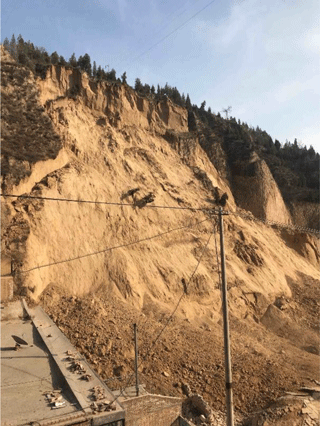
Figure 1A cracking–sliding failure that occurred in Shilou County of Shanxi Province on 10 March 2018 (36∘59′54.76′′ N), 110∘50′48.54′′ E,.
Frequent and disastrous events demand an in-depth understanding of causative factors and development patterns of loess failures to reduce the occurrence of such geohazards. This study collects a large set of data on loess cracking–sliding failures, climate, and soil temperature to facilitate a detailed analysis of the internal and external causes of such failures. This study also emphasizes the influences of slope features (i.e., slope type, gradient, height, and aspect), rainfall, freezing-thawing cycles, daily temperature fluctuations, and human engineering activities.
The study area is limited to the eastern LPC and covers northern Shaanxi and western Shanxi provinces, considering the homogeneous background of climatic, morphologic, geologic, and anthropic conditions in these regions (Fig. 2). Elevation of the study area ranges from 800 to 1300 m above sea level from southeast to northwest. The study area has a typical semiarid continental monsoon climate with four distinct seasons. The average annual rainfall in this area varies from 400 to 700 mm. Rainfall in summer (from July to September) accounts for approximately 70 % of the year (Hui, 2010; Qian, 2011; Zhu 2014). For instance, the maximum precipitation over an hour in Yan'an City can accumulate to more than 60 mm in summer (Zhu, 2014). The total rainfall in Shilou County reached 412 mm over a month from early July to early August in 2013 (Lv, 2011) and corresponded to 81% of rainfall in the same year. According to records for the past 10 years, the average annual temperature is relatively constant, ranging from 8 to 12 ∘C. However, variations in temperature in a day can occasionally be higher than 25 ∘C, the highest temperature being recorded at noon and the lowest temperature at midnight.
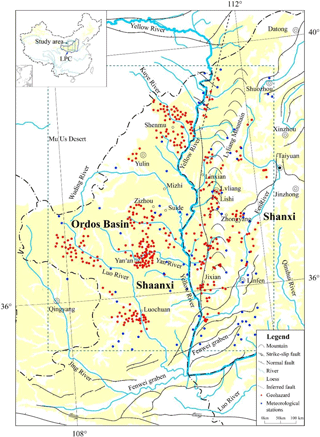
Figure 2Geological map of the study area. The red dots denote the cracking–sliding failure cases and the blue dots indicate the meteorological stations in the study area.
The study area is located in the east of the Ordos basin. The Fenwei Graben, spanning north-east to south-west, is a tectonic depression encountering a number of normal and strike-slip faults and covering more than 20 000 km2 (Huang et al., 2008; Liu et al., 2013). The thickly bedded Pleistocene loess-paleosol sequence constitutes more than 70 % of the study area and reaches a maximum thickness of 300 m. From top to bottom, the loess–paleosol sequence includes Late Pleistocene Malan Loess (Q3), Middle Pleistocene Lishi Loess (Q2), and Early Pleistocene Wucheng Loess (Q1). The Malan Loess, with thickness ranging from 10 to 30 m, is the most widespread. The Lishi Loess, with up to dozens of inter-layers of loess and paleosol, underlies the Malan Loess and forms a 60–150 m thick layer. The Wucheng Loess is sporadically exposed along some of the loess gullies. Remarkable landforms, such as loess platforms, ridges, and hillocks, have been formed in the study area because of intensive surficial erosion (Zhang, 1983, 1986). Loess platforms are mainly distributed in the Luochuan area in northern Shaanxi Province; loess ridges are mainly found in the peripheries of the Luochuan platform and the eastern regions of the Yellow River; and loess hillocks are mainly located in Yan'an, Suide and on both sides of the Yellow River between Shaanxi and Shanxi provinces.
A large set of data of loess cracking–sliding failure events were collected from published literature and unpublished reports by local governments. Slope profile, gradient, height, and aspect were derived polygonally from the initiation areas. The initiation areas, rather than the whole landslides, were compared in the following statistical analysis. The polygons were obtained by means of (1) interpretation of remote sensing images which were taken prior to the event; (2) engineering documentation if the host slope was engineered; or (3) post-event field survey and consultation with the local population. The field survey was normally conducted within 1–2 days immediately after the event. A total of 1176 cracking–sliding events were recorded in the past 20 years across the study area. Of these events, 321 were published in the literature, 670 were presented in government reports, and 185 were unpublished. All of the 1176 failures were individually reviewed by verifying the reliability, accuracy, and completeness of the original records. Finally, 458 cases (red dots in Fig. 2) were selected to set up the dataset for this study by considering the completeness of the records of any type.
Data pertaining to rainfall were obtained from the records of 75 meteorological stations (blue dots in Fig. 2), which are almost uniformly distributed across the study area. Statistical analysis shows that the variation in average annual rainfall in the past 15 years among these stations is less than 80 mm, indicating a relatively homogeneous climatic condition over the study area.
4.1 Internal factors
Loess slopes are divided into four types in terms of slope profile: rectilinear, convex, concave, and stepped slopes (Table 1). Concave and stepped slopes are more stable than the rectilinear and convex slopes. We surveyed 212 loess slopes in Lishi City in Shanxi Province and found that stepped slopes, convex slopes, rectilinear slopes, and concave slopes account for 38, 31, 18, and 13 % of all slopes, respectively (Fig. 3a). This finding is consistent with the conclusion of Qin et al. (2015), who performed a field survey on loess slopes in Yan'an City, Shaanxi Province. However, approximately one-half of cracking–sliding failures occur in rectilinear slopes. In Fig. 3b, the statistical analysis of the 458 failure cases indicates that rectilinear slopes are the most susceptible to cracking–sliding failure (48 %), followed by convex slopes (28 %). Stepped (13 %) and concave (11 %) slopes are the least susceptible to such failures.
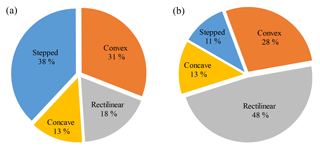
Figure 3Statistical analysis results: (a) classification of loess slopes in Lishi City, Shanxi Province, China, on the basis of a field survey of 212 loess slopes, indicating that stepped slopes are dominant in the study area. (b) Percentage of cracking–sliding failures that occurred in different types of loess slopes across the study area, showing that rectilinear slopes are highly susceptible to loess failures.

Figure 5Development of tension zones in slopes of different gradients (Stacey, 1970; Zhang et al., 2009).
In general, the overall gradients of rectilinear and convex slopes are steep, resulting in large internal stresses and stress concentrations, particularly at the shoulder and toe sections (Table 1). The bottom part of the concave slope has a gentle gradient and has a supporting function to the steep upper part, thereby relieving the stress concentration; the maximum shear stress at the foot of concave slopes is typically only one-half of the shear stress at the foot of rectilinear slopes (Zhang et al., 2009). The stress distribution pattern in each section of a stepped slope is similar to that of a rectilinear slope. However, the magnitude of internal stress of stepped slopes is lower than that of rectilinear slopes because of the small height of each step and the gentle overall gradient. These findings explain that most cracking–sliding failures occur in rectilinear slopes, although these slopes are not the dominant slope type in the loess area.
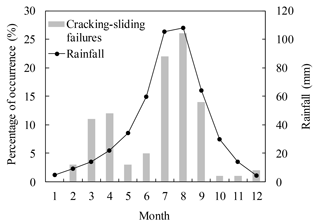
Figure 6Occurrence of cracking–sliding failures mainly in July to September and consistent with average monthly rainfall.
In addition to slope profile, the gradient, height, and aspect of loess slopes are closely related to the occurrence of cracking–sliding failures. Figure 4a shows that failure occurs mostly on slopes with gradients greater than 60∘ and that the number of failures increases with gradients. Of the cracking–sliding failures, 16, 25, and 47 % occur on slopes with gradients ranging from 61 to 70∘, from 71 to 80∘, and from 81 to 90∘, respectively. Figure 5 shows the tension zones that developed at slope shoulders, where radial and tangential stresses transform into tensile stresses. The steeper the slope is, the wider the tension band is (Stacey, 1970; Zhang et al., 2009).
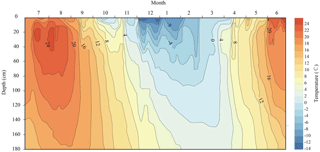
Figure 7Annual variation of temperature (∘C) within a shallow zone of a typical loess slope (Yang and Shao, 1995).
Figure 4b illustrates that slope height is another main factor controlling the occurrence of cracking–sliding failures. In the study area, most cracking–sliding failures occur on slopes with heights of 5 to 40 m and thus account for 87 % of the total number of occurrences. The remaining 13 % take place on slopes with heights of more than 40 m. A high slope normally develops a gentle gradient because of long-term weathering and erosion. By contrast, a low slope generally forms a steep gradient (Zhu et al., 2011), thereby becoming prone to collapses.
Slopes that have a surface that is exposed to sunlight are more prone to the development of cracking–sliding failures than shady slopes (Fig. 4c). Statistical analysis shows that 69 % of the cracking–sliding failures occur on slopes with aspects in the range of 90 to 270∘, particularly within 180 to 270∘ because sun-exposed slopes receive long sunshine hours and soil temperature is relatively high during the day. Therefore, high differences in temperature exist between day and night. Sunward slopes are generally subjected to more weathering than shady slopes, resulting in fractured structures, which are unfavorable to slope stability. Furthermore, people usually reside on sun-exposed slopes, and dense human engineering activities exert a large degree of disturbance on the slope body, thereby increasing the occurrence of failures.
4.2 External factors
4.2.1 Rainfall
Rainfall has a remarkable influence on the stability of loess slopes. In Fig. 6, the number of loess failures is closely and positively correlated with the average monthly rainfall of the past 15 years. Summer rainfall (July to September) in the study area accounts for approximately 60 % of the annual precipitation, and the number of cracking–sliding failures in the same period corresponds to 62 % of the total failures. This finding is consistent with that of Gao et al. (2012), who indicated that more than 60 % of loess failures happen in Gansu Province in the rainy season. Wei (1995) and Liu et al. (2012) presented a similar conclusion in Shanxi and Shaanxi provinces, respectively.
Rainfall induces loess cracking–sliding failures in three ways, namely, splash erosion, shovel runoff, and seepage. At the beginning of rainfall, soil particles with poor adhesion are separated and broken under the impact of raindrops. When potholes formed by splash erosion are filled with water, a layer of water flow forms and triggers small soil particles to move. Along with the continued rain, this water flow converges into the slope runoff to erode and destroy the slope surface further (Tang et al., 2015). In cases of persistent rainfall, preferential seepage pipes usually develop inside a slope, thereby saturating the soils, reducing the shear strength, and eventually causing cracking–sliding failures.
4.2.2 Freezing and thawing
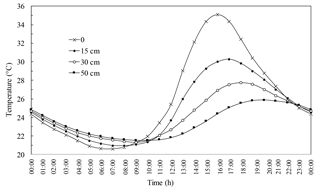
Figure 9Daily soil temperature variation in loess areas of China in summer. Data are from field monitoring from April 2014 to September 2017 in Linxian County, Shanxi, China.
Figure 6 shows that cracking–sliding failures occur frequently, not only in the rainy season from July to September, but also in the winter–spring transition from March to April. Soil temperature increases rapidly from values below 0 to values above 0 ∘C. As shown in Fig. 7, soil temperature remains negative and the frozen depth can reach approximately 1.0 m underground from late November to February in the loess areas in China. At the end of March, the ground temperature begins to increase, and the frozen layer gradually enters the thawing stage. By mid-April, the soil is rapidly heated, up to approximately 8 ∘C.
Freezing and thawing mainly promote the occurrence of cracking–sliding failures via two mechanisms: (1) frost heaving damages the soil structure and reduces soil shear strength. The loess itself contains a considerable number of large pores. Frost heaving further increases the distance between soil particles, reduces the dry density of soil, and loosens the structure, thereby reducing its cohesion and internal friction angle. (2) Thawing causes the loess structure to collapse and reduce its shear strength. Thawed water can dissolve cement, especially calcareous cement, between loess particles, consequently damaging the loess structure and increasing pore water pressure; as a result, the shear strength of the soil decreases (Pang, 1986).
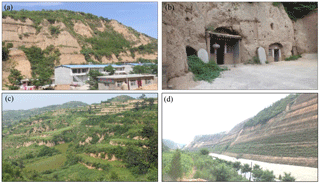
Figure 10Typical engineering activities in loess areas in China: (a) cut slopes for buildings; (b) excavations for cave dwellings; (c) terraced fields for farming; and (d) cut slopes for highways.
4.2.3 Daily temperature fluctuation
Consistent with previous findings (Wei, 1995), our results indicate a relatively high frequency of occurrence of cracking–sliding failures between 10 pm and 4 am (Fig. 8). The difference in temperature between day and night in the loess area is more obvious than that in other regions at the same latitude in China (Sun and Zhang, 2011), and variations in air temperature in a day can occasionally reach 30 ∘C. The soil at a 50 cm depth shows an average daily temperature difference of approximately 5 ∘C in summer (Fig. 9). Thermal expansion and shrinkage occur during the rapid change in day and night temperatures. Under the cyclic functioning of shrinkage and expansion stresses, a soil structure loosens.
4.2.4 Human activity
Loess areas in China have a population of more than 200 million. Human engineering activities frequently occur and mainly involve cutting slopes for buildings, excavation for cave dwellings, and construction of terraced fields and roads (Del Prete and Parise, 2013). Cutting slopes for buildings causes the side slope to become steep. Unloading induced tensile fractures are usually produced on the trailing edge of slopes during the rapid adjustment of a stress field within a slope (Fig. 10a). When a cave is excavated, roof damage (normally caving) happens because of a local tensile stress concentration if the design of a geometric section of a cave is inappropriate (Fig. 10b). Terraced fields change the original path of surface runoff and enhance rainfall infiltration. Together with irrigation, terraced fields increase the water content of loess slopes and increase their phreatic level (Fig. 10c). The majority of traffic lines in the loess area stretch along valleys and bank slopes. Slope cutting and excavation during road construction result in a large number of high and steep side slopes, which provide a suitable environment for failures (Fig. 10d).
More than half of the failures are attributed to human engineering activities (Fig. 11). In 2014, 9 of 16 failure cases that occurred in Yan'an City were caused by extremely steep slopes for cave dwelling construction, and the 7 other cases were consequences of improper treatment of side slopes for road construction (Lei, 2001). These findings demonstrate that intense human activities likely result in a high probability of loess failures.
This study investigates the influencing factors and development patterns of loess cracking–sliding failures in the eastern LPC according to a large collection of field investigation data. The following conclusions are obtained:
-
the influencing factors of cracking–sliding failures are divided into internal and external causes. Internal causes include various features, such as slope geometry, height, gradient, and aspect of loess slopes, whereas external causes comprise rainfall, freezing-thawing cycles, temperature fluctuation, and human engineering activities;
-
cracking–sliding failures more likely occur in rectilinear and convex slopes than in concave and stepped slopes. Rectilinear and convex slope gradients are generally steep, stress concentrations are obvious, and slope stability is poor. The stress concentration in concave and stepped slopes is minimized, and stability is fair. Cracking–sliding failure more likely takes place on slopes with gradients greater than 60∘, and the greater the gradient is, the higher the likelihood of failures. cracking–sliding failure also tends to occur on slopes with heights of 5 to 40 m. Slopes below 5 m have low internal stress and high stability. Slopes above 40 m are generally gentle with low stress concentration. The dominant aspect of the development of cracking–sliding failure is within 180 to 270∘ (sun-exposed slopes) because of the evident temperature difference between day and night and the strong weathering;
-
the occurrence of cracking–sliding failure displays a particular time pattern. Within a year, its occurrence coincides with seasonal rainfall. Failures mainly occur in the rainy season, or from July to September. In addition, failures frequently take place from March to April because of freezing and thawing. Within a day, failures happen mostly from 10 pm to 4 am because of the large temperature variation between day and night; and
-
the more intense the engineering activities, the greater the possibility of loess failures. Human engineering activities in loess areas include cutting slopes for buildings, excavation of cave dwellings, and construction of terraced fields and roads. These engineering activities usually lead to a rapid change in the features and stress field of slopes. Such high and steep side slopes tend to develop unloading-induced tensile fractures, thereby increasing the possibility of loess failures.
As the data for this research were collected from published literature and unpublished reports by local governments, the data are not all publicly accessible. Some unpublished reports are kept confidential from public by the government. However, the data set can be provided by the authors and the government upon request from readers for personal research usage.
The authors declare that they have no conflict of interest.
This study was supported by the Key Program of National Natural Science
Foundation of China (No. 41630640), the Major Program of the National Natural
Science Foundation of China (No. 41790445), the 2014 Fund Program for the
Scientific Activities of Selected Returned Overseas Professionals in Shanxi
Province, Shanxi Scholarship Council of China, Outstanding Innovative Teams
of Higher Learning Institutions of Shanxi, Soft-science Fund Project of
Science and Technology in Shanxi, Research Project for Young Sanjin
Scholarship of Shanxi, Collaborative Innovation Center for Geohazard Process
and Prevention at Taiyuan Univ. of Tech., Recruitment Program for Young
Professionals of China.
Edited by: Mario
Parise
Reviewed by: four anonymous referees
Cruden, D. M. and Varnes, D. J.: Landslide types and processes, in: Landslides: investigation and mitigation, Transportation Research Board Special Report, 247, 1996.
Del, P. S. and Parise, M.: An overview of the geological and morphological constraints in the excavation of artificial cavities, in: Proceedings 16th International Congress of Speleology, edited by: Filippi, M. and Bosak, P., Brno, 21–28 July 2013.
Gao, H., Zhang, Y. J., and Zhang, X. G.: Factors on geological hazards of losee slope in Lanzhou city, Gansu Geol., 3, 30–36, 2012.
Huang, Z., Xu, M., Wang, L., Mi, N., Yu, D., and Li, H.: Shear wave splitting in the southern margin of the Ordos Block north China, Geophys. Res. Lett., 35, 402–411, 2008.
Hui, X.: Research on relationship between geo-hazard and rainfall in Loess Plateau of Northern Shanxi Province, PhD thesis, Chang'an University, Xi'an, China, 2010.
Lei, X. Y.: Loess landslide and loess collapse, in: Geohazards of Loess Plateau and their relation with human activities, Geol. Press., Beijing, 2001.
Liu, J., Zhang, P., Lease, R. O., Zheng, D., Wan, J., Wang, W., and Zhang, H.: Eocene onset and late Miocene acceleration of Cenozoic intracontinental extension in the North Qinling range–Weihe graben: Insights from apatite fission track thermochronology, Tectonophysics, 584, 281–296, 2012.
Liu, J. N., Gu, Y., Jin, J., Ni, S. H., and Shen, Y.: Analysis of rainfall, floods and droughts in middle Shanxi in recent years, China, J. China. Hydrol., 2, 51–54, 2013.
Lv, M.: The present situation of the loess collapse of geological sisasters in Shanxi Province and the water sensitivity analysis, PhD thesis, Taiyuan University of Technology, Taiyuan, China, 2011.
Pang, G. L.: A discussion on maximum seasonal frost depth of ground, China, J. Glaciol. Geocryol., 3, 253–254, 1986.
Pye, K.: Aeolian dust and dust deposits, Academic Press, London, 1987.
Qian, P.: Study of types for highway drainage system in loess areas in northern Shaanxi Province, PhD thesis, Chang'an University, Xi'an, China, 2011.
Qin, L. L., Qi, Q., and Ju, Y. W.: Study on the feature governance research of loess geological disasters in Shanxi Province, China, Shanxi Archit., 6, 58–59, 2015.
Qu, Y. X., Zhang, Y. S., and Chen, Q. L.: Preliminary study on loess slumping in the area between northern Shaanxi and western Shanxi – taking the pipeline for transporting gas from west to esat in China, China, J. Eng. Geol., 9, 233–240, 2001.
Richthofen, F.: On the mode of origin of the Loess, Geol. Mag., 9, 293–305, 1882.
Smalley, I., Marković, S. B., and Svirčev, Z.: Loess is [almost totally formed by] the accumulation of dust, Quatern. Int., 240, 4–11, 2011.
Stacey, T. R.: Stress surrounding open-pit mine slopes, Int. J. Rock. Mech. Min., 10, 523–533, 1973.
Sun, Z. X. and Zhang, Q.: Analysis of climate characteristics of land surface temperature and energy in the semi-arid region in the Loess Plateau, China, J. Desert Res., 5, 1302–1308, 2011.
Tang, Y. M., Feng, W., and Li, Z. G.: A review of the study of loess slump, China, Adv. Earth Sci., 1, 26–36, 2015.
Wei, Q. K.: Collapse hazards and its distribution features of time and space in Shaanxi Province, China, J. Catastrophol., 10, 55–59, 1995.
Xin, C. L., Yang, G. L., Zhao, Z. P., Sun, X. H., Ma, W. Y., and Li, H. R.: Characteristics, causes and controlling of loess collapses in Beishan mountain of Tianshui city, China, Bull. Soil Water Conserv., 33, 120–123, 2013.
Yang, W. Z. and Shao, M. A.: Researches of soil moisture in Loess Plateau, Sci. Press, Beijing, 1995.
Zhang, H.: Study of water migration and strength of the loess under freezing-thawing action, PhD thesis, Xi'an University of Architecture and Technology, Xi'an, China, 2014.
Zhang, Z. H.: The compilation principle of landscape type map of Chinese Loess Plateau, China, Hydrogeology and Engineering Geology, 2, 29–33, 1983.
Zhang, Z. H.: Institute of hydrogeology and engineering geology of Chinese Academy of Geological Sciences, China, Landscape type Map and Instructions of Chinese Loess Plateau (1:500 000), Beijing, Geological Press., 1986.
Zhang, Z. Y., Wang S. T., Wang L. S., Huang R. Q., Xu Q., and Tao L. J.: Engineering geological analysis principle, Geol. Press., 2009.
Zhu, J. H.: Study on the relationship between slope geometrical morphology and landslide collapse disasters in Yan'an, PhD thesis, Chang'an University, Xi'an, China, 2014.
Zhu, Y. C., Li, J., and Ren, Z. Y.: Change tendency and relevant analysis about cultivated land and population in Loess Plateau during about 300 years, China, J. Shaanxi Norm. University (Natur. Sci. Ed.), 3, 84–89, 2011.