the Creative Commons Attribution 4.0 License.
the Creative Commons Attribution 4.0 License.
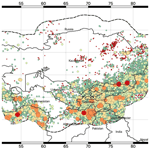
Harmonizing seismicity information in Central Asian countries: earthquake catalogue and active faults
Stefano Parolai
Natalya Silacheva
Anatoly Ischuk
Kanatbek Abdrakhmatov
Zainalobudin Kobuliev
Vakhitkhan Ismailov
Roman Ibragimov
Japar Karaev
Paola Ceresa
Paolo Bazzurro
Central Asian countries, which include Kazakhstan, the Kyrgyz Republic, Tajikistan, Turkmenistan, and Uzbekistan, are known to be highly exposed to natural hazards, particularly earthquakes, floods, and landslides. With the aim of enhancing financial resilience and risk-based investment, planning to promote disaster and climate resilience in Central Asia, the European Union, in collaboration with the World Bank and the Global Facility for Disaster Reduction and Recovery (GFDRR), launched the Strengthening Financial Resilience and Accelerating Risk Reduction in Central Asia (SFRARR) regional programme. Within this framework, a consortium of national and international scientific institutions was established and tasked with developing a regionally consistent multi-hazard and multi-asset probabilistic risk assessment. The overall goal was to improve scientific understanding on local perils and to provide local stakeholders and governments with up-to-date tools to support risk management strategies. However, the development of a comprehensive risk model can only be done with the basis of an accurate hazard evaluation, the reliability of which depends significantly on the availability of local data and direct observations.
This paper describes the preparation of the input datasets required for the implementation of a probabilistic earthquake model for the Central Asian countries. In particular, it discusses the preparation of a new regional earthquake catalogue harmonized between countries and homogenized in moment magnitude (Mw), as well as the preparation of a regional database of selected active faults with associated slip rate information to be used for the construction of the earthquake source model. The work was carried out in collaboration with experts from the local scientific community, whose contribution proved essential for the rational compilation of the two harmonized datasets.
- Article
(6393 KB) - Full-text XML
- BibTeX
- EndNote
Except for the stable continental part of Kazakhstan, Central Asia is classified as a highly seismically active region. Large historical earthquake events have occurred, mostly caused by thrust and reverse faults generated by the collision of the Eurasian and Indian plates (Ullah et al., 2015). Such a compressional regime was responsible for the development of the Cenozoic belts of Tien Shan and Pamir, which accommodate a great part of the regional deformation (e.g. Abdrakhmatov et al., 1996; Zubovich et al., 2010) and where most of the seismicity occurs, often with earthquakes with a magnitude larger than 7. Notable examples are the Verny (Ms=7.3, 1887), Chilik (Ms=8.3, 1889), Kemin (Ms=8.2, 1911), Chatkal (Ms=7.5, 1946), and Suusamyr (Ms=7.3, 1992) earthquakes (Abdrakhmatov et al., 2003). The Kyrgyz Republic alone has been hit by 18 destructive earthquakes in the last 50 years, with up to USD 6.4 billion of potential economic losses, and this total is estimated to be exceeded for residential buildings with a 10 % probability in the next 50 years (Free et al., 2018). This seismically active region formally separates the more stable regions of the Tarim basin to the south and the Kazakh platform to the north, where a more moderate intraplate seismicity is observed but which is still capable of generating significant earthquakes.
On the territory of Turkmenistan, four seismically active regions can be identified: Turkmen–Khorasan, Balkan–Caspian, Elbursky, and Gaurdak–Kugitang. Strong destructive earthquakes took place, such as the catastrophic Krasnovodsk earthquake on 8 July 1895 (M=8.2), Germab earthquake on 1 May 1929 (M=7.2), Kazanjik earthquake on 5 November 1946 (M=7.0), catastrophic Ashgabat earthquake on the night of 5–6 October 1948 (M=7.3), and Balkan earthquake on 6 December 2000 (M=7.3). The larger seismicity is observed in the Turkmen–Khorasan and Balkan–Caspian regions, with Ashgabat as the most seismically active area of the Turkmen–Khorasan region. Tajikistan is a seismically active region as well. Few destructive earthquakes are known, such as the Karatag earthquake in 1907 with MLH=7.4, the Sarez earthquake in 1911 with MLH=7.4, the Khait earthquake in 1949 with MLH=7.4, and the recent second Sarez earthquake in 2015 with Mw=7.2.
While most of the regional seismicity occurs within the first 40 km of the crust, deep earthquakes have also been observed down to 300 km depth in the Pamir–Hindu Kush area (King et al., 1999). Although reverse and thrust source mechanisms predominate due to the local tectonic regime, strike-slip and – to a lower extent – normal mechanisms (or a combination thereof) are also present.
In this paper we describe the development of the input datasets required for the implementation of the earthquake component of a new probabilistic multi-risk model for the Central Asian countries of Kazakhstan, the Kyrgyz Republic, Tajikistan, Turkmenistan, and Uzbekistan. This study builds on and updates the results of previous important regional studies such as the Global Seismic Hazard Assessment Program (GSHAP; Giardini, 1999; Ulomov and The GSHAP Region 7 Working Group, 1999) and the Earthquake Model of Central Asia (EMCA) project (see Bindi et al., 2011, 2012; Ullah et al., 2015). It also considers recent progress at the national level, including, among others, the work of Ischuk et al. (2018) and Ischuk and Mamadjanov (2014) for the Kyrgyz Republic, Tajikistan, and eastern Uzbekistan; Silacheva et al. (2018) and Mosca et al. (2019) for Kazakhstan; the Central Asia Seismic Risk Initiative (CASRI; Abdrakhmatov, 2009) for the Kyrgyz Republic; and Artikov et al. (2018) for Uzbekistan. This model is part of the EU-funded Strengthening Financial Resilience and Accelerating Risk Reduction in Central Asia (SFRARR) regional programme, managed by the World Bank in collaboration with the Global Facility for Disaster Reduction and Recovery (GFDRR). In particular, this work focuses on (i) the development of a new regional earthquake catalogue, harmonized between countries and homogenized in moment magnitude (Mw), built using the most up-to-date information available from global and local sources, and (ii) the development of a selected dataset of major active lineaments that includes slip rate information to complement the observed seismicity for the construction of a comprehensive probabilistic seismic hazard model for Central Asia. Further details on this model can be found in the companion article of this special issue (Poggi et al., 2023), where the construction of a hybrid source (distributed seismicity and finite faults) and ground motion model is extensively described, along with a thorough discussion of the results. It is important to note that the development of such regional datasets cannot occur without the contribution of experts from the local scientific community. Partnering with local government institutions and scientific agencies is also an essential step in facilitating a consensus on models for possible integration into national seismic codes. Following this concept, the programme consortium has partnered with the local scientific communities to share information and develop a review process that ranged from compiling the datasets to building the earthquake hazard model and discussing the respective results. In the following the creation process of the two main datasets is presented and discussed in detail.
Nowadays, the compilation of a modern earthquake catalogue with homogeneous magnitude information (e.g. Mw) is an essential step for the development of a probabilistic earthquake hazard model because it provides basic information for evaluating the location, magnitude, and occurrence of potentially damaging future earthquakes.
The main notable examples of the compilation and unification of earthquake catalogues in Central Asia were carried out within the framework of the international projects CASRI (from historical times until 2005) and EMCA (until 2009, Mikhailova et al., 2015). Subsequently, the available information was supplemented with new data from SEME (Seismological Experimental and Methodical Expedition) and KNDC (Kazakhstan National Data Centre) for Kazakhstan and adjacent areas to support the development of a new national seismic zonation model and seismic microzonation of Almaty. However, a revision of the EMCA catalogue (i.e. data prior to 2009) is needed. The epicentres of the earthquakes and the magnitude conversion relations used to create the catalogue, including a description of earthquake size on the moment magnitude (Mw) scale, need to be revised using the latest information. Data after 2009 may be inconsistent in the catalogues of neighbouring Central Asian countries due to differences in the development of observation networks and the use of different processing techniques.
In the following, we present the processing steps, key assumptions, and subjective decisions we made in creating a new harmonized earthquake catalogue for Central Asia (hereafter HECCA) for moment magnitude (Mw) representation. The catalogue was created by analysing and combining publicly available global earthquake information (e.g. the reviewed bulletin of the International Seismological Centre, ISC-REV, and its Global Instrumental Earthquake Catalogue, ISC-GEM; Global Centroid Moment Tensor, GCMT; USGS National Earthquake Information Centre, USGS-NEIC) with information from previous regional projects and local authorities of the states participating in the SFRARR project.
Although the catalogue represents the best current snapshot of available earthquake information for the region, we nevertheless plan to make future additions to this compilation by gradually incorporating new data from local agencies, temporary networks, and regional projects as they become publicly available. For the compilation, we used a set of freely available and open-source Python tools originally developed as part of the Global Earthquake Model Foundation to simplify and enable the process of future extensions (see https://github.com/klunk386/CatalogueTool-Lite, last access: 22 July 2024).
2.1 The harmonization approach
In order to create a harmonized dataset, it is usually necessary to collect and merge information from different sources. However, harmonizing data from different neighbouring regions and homogenizing earthquake parameters (e.g. location solutions, reported time, intensity scale) to avoid duplication is a rather complex process that requires establishing a set of objective criteria for selection, duplicate identification, merging, and conversion. This is often the case when different seismological agencies are reporting the same events but with different magnitudes (e.g. ML, Md, Ms). The same problem affects source location solutions when, for example, different networks use different earthquake phases, processing algorithms, or modelling assumptions (e.g. Earth velocity structure). It should be noted that the terms “homogenization” and “harmonization” are used in this paper in slightly nuanced contexts and with different meanings, although they are closely related and often mistakenly thought to be synonymous. While homogenization usually refers to the “process” that leads to a uniform representation, harmonization emphasizes the properties of the final product, especially in relation to its applications. In this study, for example, we have compiled a catalogue that is homogeneous in magnitude, reflecting the process of standardizing scales to achieve uniformity. However, the resulting catalogue represents a harmonized dataset across countries, specifically tailored to seismic hazard analysis.
In compiling the HECCA, we proceeded in two steps. First, information from global sources and previous regional projects was collected, reviewed, and combined into a unique base compilation (the backbone), which was then supplemented by local/national datasets provided by consortium partners. It must be emphasized that the focus of this work is primarily on improving the catalogue during the “instrumental period” (roughly after 1900 but especially after 1950, when modern analogue and then digital records became available). Rather, the historical events were imported directly from the EMCA compilation, which is considered the authoritative source for this period, without further modification.
2.2 Input datasets
Authoritative global sources of information for creating the backbone part of the catalogue include the ISC-GEM catalogue, the ISC reviewed bulletin, the HRVD-GCMT (Harvard) bulletin, the USGS-NEIC bulletin, and the GEM historical catalogue and regional events from the EMCA catalogue (Table 1). All datasets were preprocessed by filtering out events with a magnitude (any reported type) below 2 and with an epicentre outside a buffer region of about 300 km around the five target states (Fig. 1), since these events would not contribute significantly to the hazard. The national earthquake catalogues of the five local consortium partners (see Table 3) were then reviewed to supplement the backbone compilation.
Table 1Summary of catalogue sources used to create the HECCA backbone compilation (events selected within the buffer region surrounding the study area).
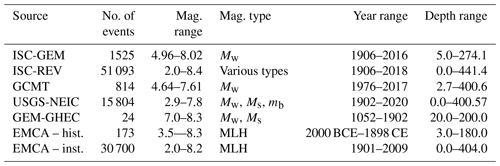
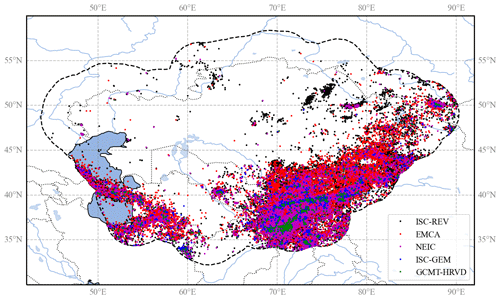
Figure 1Distribution of epicentres of earthquake events from the main sources used to assemble the backbone compilation. The investigated area includes the five Central Asian countries, plus a buffer region of about 300 km around the country borders (dashed black line).
2.2.1 ISC-GEM
The ISC-GEM global instrumental catalogue is an improved version of the bulletin of the International Seismological Centre (ISC; Storchak et al., 2013, 2015; Di Giacomo et al., 2018). The version used in this study (version 7, published 25 June 2022) currently covers the period 1904–2016. The compilation benefits from an accurate relocation of earthquake events, performed using a single location procedure and a uniform velocity model (Bondár et al., 2015), while magnitudes have been all converted to the Mw scale according to the rules defined in Di Giacomo et al. (2015). At the global level, the catalogue currently covers the magnitude range from about 5 to 9.5, with the magnitude record from 5.5 onwards being considered complete from 1935 onwards.
The ISC-GEM catalogue is the primary and authoritative global source for the backbone catalogue for Central Asia in the instrumental period. When selecting and merging events from different sources, the solutions for the ISC-GEM locality always have the highest priority over other solutions. In contrast, magnitude solutions have higher priority only when direct moment magnitude (Mw) estimates are not available (e.g. from the GCMT bulletin).
2.2.2 ISC reviewed bulletin
The reviewed version of the ISC bulletin (Storchak et al., 2017; http://www.isc.ac.uk/, last access: 22 July 2024) is used to add events not covered in the ISC-GEM ca last access: 22 July 2024 talogue, especially for magnitudes below about 5.5, which are still relevant to earthquake hazard analysis.
The ISC reviewed bulletin contains multiple location and magnitude solutions (with different magnitude types) from different reporting agencies for each event. The Central Asia selection of the bulletin consists of 51 093 events, with location solutions from 33 agencies and magnitude solutions from 108 agencies (Table 2). The ISC always provides a preferred (“prime”) location solution, which is often – but not always – the ISC's own solution. For catalogue harmonization, we use the ISC prime location when available, which is derived from the same algorithm and velocity model used for the ISC-GEM catalogue, while for defining magnitude we use a selection process based on agency prioritization rules, which are described in more detail in the next sections.
Table 2Location and magnitude solutions relative to each reporting seismological agency of the ISC reviewed bulletin in the study region.
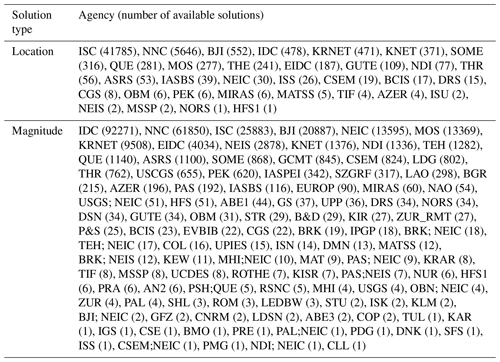
For a comprehensive list and description of reporting agency codes and magnitude types, refer to the following:
-
http://www.isc.ac.uk/iscbulletin/agencies (last access: 22 July 2024),
-
https://www.usgs.gov/natural-hazards/earthquake-hazards/science/magnitude-types (last access: 22 July 2024).
2.2.3 GCMT bulletin
The Global Centroid Moment Tensor (GCMT) catalogue (Ekstrom et al., 2012) is a collection of moment tensor solutions for earthquakes with Mw>4.5, from 1972 to 2013. While the solutions for the hypocentre come from external agencies (such as the ISC) and are therefore usually excluded from our analysis (or marked as duplicates), Mw solutions are always assumed to be authoritative reference estimates. The selection for Central Asia consists of 814 events with Mw between 4.6 and 7.6. Analysis of the moment tensor solutions for these events is also important to constrain the rupture mechanisms of the earthquake source model (see sections on defining rupture mechanisms).
2.2.4 USGS-NEIC bulletin
Although the International Seismological Centre bulletin is considered the definitive global archive of parametric earthquake data, the National Earthquake Information Centre (NEIC) of the United States Geological Survey (USGS) preliminary bulletin may provide useful additional information not yet reviewed by the ISC. The NEIC database generally has the lowest priority compared to previous compilations, both in terms of location and magnitude solutions.
2.2.5 GEM historical earthquake catalogue
As in the case of the ISC-GEM catalogue, the GEM historical earthquake catalogue (GEM-GHEC; Albini et al., 2014) is an authoritative global source of information on historical earthquakes. The catalogue covers events from about 1000 to 1903 and was compiled based on macroseismic intensity data and a review of the literature available worldwide (papers, reports, volumes). Unfortunately, the GEM-GHEC has limited coverage of Central Asia, with only 24 events reported with magnitudes greater than 7, most of which are recorded in the EMCA catalogue.
2.2.6 EMCA catalogue
The Earthquake Model of Central Asia (EMCA) catalogue (Mikhailova et al., 2015) contains information on 33 620 earthquakes that occurred in the Central Asian countries (Kazakhstan, the Kyrgyz Republic, Tajikistan, Uzbekistan, and Turkmenistan) and represents the first major effort to harmonize catalogue data in the region.
The EMCA catalogue covers a period from 1000 to 2009 and is homogenized in surface wave magnitude (MLH) for the horizontal component (Rautian et al., 2007). The MLH magnitudes are not original estimates but were converted from either body wave magnitude (mb), energy class (K), or Mpva (regional magnitude of body waves determined by the P wave recorded by short-period instruments) using empirical regression analyses.
For the harmonization process, the catalogue was divided into two main blocks, the pre-instrumental or historical (pre-1900) period and the instrumental (post-1900) period. Since the review of historical information is outside the scope of this project, all reported events prior to 1900 were considered authoritative sources for the creation of the new harmonized catalogue. In contrast, location solutions from the instrumental period were thoroughly reviewed and, where necessary, replaced with solutions from the new catalogue entries. Magnitude solutions were always considered authoritative over all other magnitude types (Ms, mb, Ml, Md) but not over Mw estimates from the moment tensor inversion and the ISC-GEM catalogue.
2.2.7 Local earthquake datasets
The earthquake records from the backbone compilation were then integrated with information from the local earthquake catalogues provided by the national seismological agencies. These datasets are the result of regional earthquake monitoring conducted with temporary and national permanent seismic networks and are an essential complement to the information available worldwide, especially for low magnitudes. The main characteristics of the national datasets reviewed for inclusion in the HECCA are listed in Table 3. It should be noted that several events of the local contributions were already available in the global sources and in the EMCA catalogue. Therefore, the selection focused on identifying and including the missing events, especially for the most recent time interval, according to the harmonization procedures described in the following sections.
2.3 Duplicate finding
To create a unique catalogue compilation, the first step is to identify the same events from the different input sources and merge them using a duplicate detection algorithm. Our approach is based on spatial and temporal matching of the reported hypocentral solutions within predefined windows, the length of which is tuned to the expected accuracy of the solution in each time range. For the current study, we determined an optimal time range of 15 s and a spatial distance of 60 km between solutions (Fig. 2). This combination allowed us to capture over 95 % of the duplicate events in the instrumental period (after 1900). Because this is an automated process, errors in identification are still possible. Because there is no unique window length that allows for all duplicate events in all catalogues to be captured without erroneously including a subset of independent events, an additional magnitude range match condition was added to reduce the likelihood of false identifications. The condition of a difference of 1 magnitude unit was introduced as the maximum allowed gap between duplicate events.
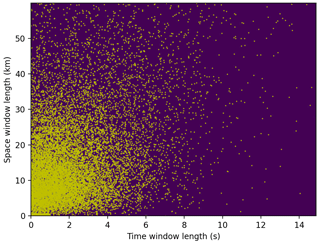
Figure 2Temporal and spatial distance of events identified as duplicates between the ISC bulletin and the EMCA catalogue. More than 95 % of the events are covered by a 15 s, 60 km window, although the majority of events are within a 5 s, 25 km difference.
Due to the limited extent of historical records (from EMCA and GEM-GHEC), the merging of historical data sources was done manually.
Once duplicate events are identified between the catalogues, the solutions are merged into a single event with multiple locations. As a final step, the preferred location solutions are then selected according to ad hoc priority rules (see Table 4 for the main backbone catalogue contributions to location solutions, sorted by priority). It is worth noting that EMCA only has a lower priority compared to other reporting sources for the location solutions. Indeed, a significant portion of EMCA events have low spatial resolution (resulting in a “gridded” pattern in the distribution of epicentres). As mentioned earlier, reporting sources such as ISC-GEM (and more recently ISC-REV) now provide reprocessed solutions that use newer and better performing algorithms and regionally consistent velocity models.
Table 4Number of events selected as preferred location solutions from the various input datasets used to create the backbone catalogue. Sources are sorted from highest (left) to lowest (right) priority.
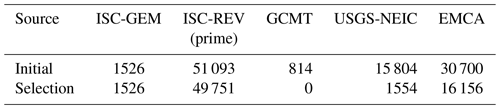
2.4 Magnitude homogenization
A key point in the harmonization process is the representation of all available earthquake events with a uniform target magnitude. In this study, we use moment magnitude (Mw; Hanks and Kanamori, 1979) as the reference type because it is directly related to earthquake size and energy and there is no saturation at high magnitudes. However, events with a direct estimate of Mw (from waveforms) are limited (e.g. post-1976 for the GCMT catalogue), so conversion from other scales is often required.
2.4.1 Agency selection
For magnitude homogenization we applied a magnitude agency selection criterion analogous to that used for the selection of the preferred location. In a first step, we examined the availability of different magnitude types from each available agency. Subsequently, the most reliable agencies were selected and sorted according to specific priority rules. Prioritization was based on magnitude type (from higher to lower priority: ) and agency-specific selection criteria. Table 5 provides the final list of magnitude types and agency priorities. Using these rules, a single magnitude estimate is then assigned to each event (Table 6).
2.4.2 Magnitude conversion
As a final step in the construction of the catalogue, all events with different magnitude types must be converted to a reference scale, in this case the moment magnitude (Mw). For the conversion, we prefer to use robust, well-tested, and globally calibrated magnitude conversion relations for the most common magnitude scales (Ms, mb, Ml), whereas, for the conversion of specific scales (Mpv and MLH) to Mw, ad hoc relations were developed using an orthogonal regression approach (e.g. Fig. 3). In these models, the saturation limits of each scale were included as an additional physical constraint on the regression model to stabilize the regression result. See Table 7 for the complete list of conversion rules.
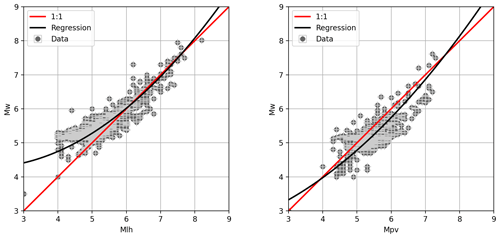
Figure 3The magnitude relationships developed for the conversion of the MLH and Mpv scales to Mw by fitting a second-degree polynomial to observed magnitude pairs using the orthogonal least-squares regression technique (Table 7).
2.5 Integration of local data
The harmonization process (duplicate identification, location selection, magnitude conversion) was first performed on the global and regional datasets to produce the backbone part of the harmonized catalogue. The inclusion of local (national) datasets in the backbone compilation was then done using the same integration criteria but in a separate phase. Merging of the different national contributions was done for each country individually so that each dataset was assumed to be authoritative for its territory and no additional priority rules were needed for selection. In addition, uniform rules for magnitude conversion were used, as indicated in Table 7.
2.6 The final compilation
The harmonized backbone catalogue for Central Asia presently consists of 77 376 events through 2020 in the range (see, e.g. Figs. 4–6), with a minimum regional completeness of about Mw 4–4.5. Of the total number of compiled events, about 10 646 are from newly recorded local data (roughly 13 % of the total). The historical period (pre-1900) is mostly covered by the EMCA catalogue, while the instrumental period has been thoroughly revised and expanded by including new homogenous location solutions from global datasets, additional magnitude conversion relations, and more recent events (e.g. after 2009) from regional datasets.
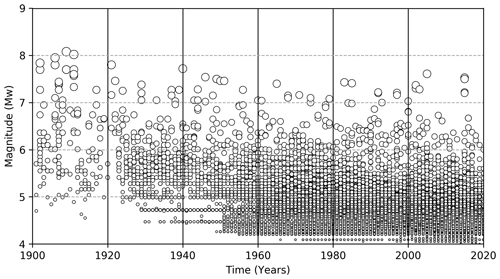
Figure 5Time–magnitude distribution of the earthquake events of the HECCA in the instrumental period (after 1900).
2.7 Declustering
Probabilistic seismic hazard analysis assumes that earthquake events are independent and that their probability distribution corresponds to a Poisson process. In reality, earthquake catalogues are characterized by a proportion of correlated events that are highly interdependent in space and time. The clustering of correlated events may be of natural origin (e.g. the aftershocks following a major event); be caused by the interference between human activity and the natural environment (e.g. geothermal exploitation, which is the extraction of thermal energy by pumping fluids from a geothermal reservoir, and carbon sequestration, which is process of capturing and storing atmospheric carbon dioxide in an already depleted reservoir); or be purely anthropogenic, such as those originating from the use of artificial sources (e.g. blasting, mining explosions). All correlated events (both natural and associated with human activity) must be removed so that the earthquake record is equivalent to a Poisson process. Declustering techniques are usually used for this purpose. What remains can be considered a collection of independent mainshocks (i.e. events with the largest magnitude in a cluster) of purely tectonic origin.
2.7.1 Natural events
In this study, aftershocks, foreshocks, and triggered events in all clusters are removed using a direct-search approach, where all events that are within a magnitude-dependent time window from the assumed mainshock (largest event in the cluster) are considered dependent and then removed from the catalogue. Several time–distance windows have been proposed in the literature. We tested the algorithms of Gardner and Knopoff (1974), Uhrhammer (1986), and Grünthal (1985), each of which provided different estimates of the relative aftershock fraction. By directly testing the performance of the three algorithms on the HECCA (e.g. Fig. 7, Table 8), both in terms of the geographic distribution of residual events and the variation in frequency of occurrence, we selected the approach of Gardner and Knopoff (1974) as that one provides the most reasonable and balanced result for Central Asia, as it is not too aggressive while being able to capture most of the dependent events.
2.7.2 Induced and artificial events
In principle, induced and artificial events caused by humans should be known from the beginning and could therefore be manually removed from the earthquake record. However, in the case of Central Asia, the record of these events is fragmented and often incomplete. Therefore, an alternative (and possibly automated) removal strategy needs to be introduced and applied. The main problem is that these events may overlap in time and space with existing background seismicity, which should not be modified to avoid a biased estimation of the local hazard.
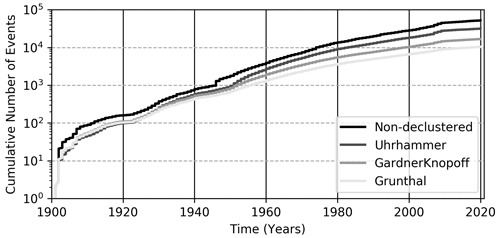
Figure 7Cumulative number of events over time for the full (non-declustered) HECCA and for the three catalogues obtained using the three considered declustering algorithms.
Here, we applied a modification of the declustering algorithm used to clean up natural aftershocks, assuming that such artificial events are also highly clustered in space and time and that, at the same time, the largest events in the cluster are likely to be of natural origin. Based on a Gardner and Knopoff (1974) window, a variable scaling factor is then applied to the spatial and temporal extent of the window until an optimal trade-off between cleaned events and remaining seismicity (compatible with the regional background) is found. After several trials, we determined the best scaling factor for the region to be 100. To avoid altering the earthquake record in areas not affected by humanmade events, the procedure is applied only to buffer regions (polygons) with known anthropogenic activity. Currently, seven polygons have been identified and reported by the local partners of the consortium, five of which are located in the inner stable cratonic part of Kazakhstan and one of which is in the more active region near the border with the Kyrgyz Republic (e.g. Fig. 8). According to the proposed procedure, 558 events were identified as anthropogenic, which is about 2 % of the original declustered catalogue.
The inclusion of finite-fault source models in a probabilistic seismic hazard assessment is now becoming standard practice (e.g. Danciu et al., 2017; Valentini et al., 2019; Poggi et al., 2020; Gómez-Novell et al., 2020), as it provides a convenient approach to better represent near-field ground motions when targeting specific and well-defined active structures, thus complementing some of the limitations of distributed seismicity models. However, accurate modelling of potentially seismogenic faults is only possible if sufficient information (fault geometry, kinematic parameters, displacement rates) is available with sufficient confidence for the area under study (e.g. clear surface expression, known segmentation, well-documented evidence of Quaternary activity or direct seismicity), which is not the case for most observed tectonic lineaments. This section presents the construction of a dataset of active faults from existing regional compilations to be used for the probabilistic seismic hazard assessment of Central Asia (Poggi et al., 2023).
3.1 The modelling strategy
The fault parameterization adopted in this study is determined by the requirements of the chosen source-modelling strategy. We use the modelling formalism of the OpenQuake engine (Pagani et al., 2014a, b), an open-source seismic hazard and risk calculation software developed, maintained, and distributed by the Global Earthquake Model (GEM) Foundation. However, finite-fault sources can be modelled in OpenQuake in different ways, depending on how accurate the fault representation should be. In this study, we use the “simple-fault” modelling approach (see the OpenQuake technical manual for more details on modelling), in which the three-dimensional fault geometry is approximated by extending the fault trace from Earth's surface to the lower seismogenic depth with an inclination equal to the dip angle (Fig. 9). The complete list of modelling parameters required for a simple fault and the corresponding values used as a reference in this work are summarized in Table 9.
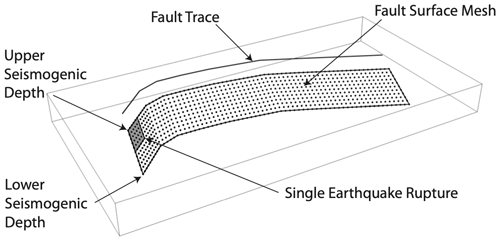
Figure 9Simple-fault source in the OpenQuake engine (modified from The OpenQuake-engine Book: Hazard, Pagani et al., 2014b).
3.2 Datasets of regional active faults
At the regional level, the most significant existing compilations that are uniform and consistent across Central Asian countries are the GEM Global Active Faults Database (GEM GAF-DB; Styron and Pagani, 2020; Fig. 10) and the Active Faults of Eurasia Database (hereafter AFEAD; Bachmanov et al., 2017; Zelenin et al., 2022; Fig. 11), which review and summarize most of the available information from published scientific studies for the target area.
In particular, the AFEAD currently contains more than 20 000 lineaments (faults, fault zones, and associated structural shapes) that show evidence of recent displacement during the Late Pleistocene and Holocene. For each mapped fault, the database reports morphological and kinematic information with quality indicators (four reliability classes from A to D, from most reliable to least reliable, as described in Bachmanov et al., 2017, and summarized in Sect. 3.3 of the AFEAD documentation website available at http://neotec.ginras.ru/index/english/database_06_eng.html, last access: 27 February 2024) and, where possible, an assessment of displacement rates (three ranks of late Quaternary movements). Conversely, only a limited number of faults from the GEM GAF-DB are sufficiently complete to be used for building fault source models (e.g. because of a lack of estimates of displacement rates). In direct comparison, these faults are also included in the AFEAD, so most of the information is shared between sources. For this reason, although the AFEAD has some local inconsistencies that require some attention (e.g. in the segmentation of faults), at the present time it is the primary information base for building the finite-fault source model for this study.
The AFEAD was then exported to an open format compatible with the GEM GAF-DB (in GeoJSON format; see the following section) to facilitate the comparison and the integration of additional information that may be derived from new local studies. Such a compilation will be made openly available to encourage further development of the area.
3.3 Format conversion and fault selection
To create the fault source model, the AFEAD was first converted to an intermediate format compatible with the GEM Global Active Faults Database. Such a format is basically required to build the OpenQuake source model using the Model Building Toolkit from GEM, as was done in Poggi et al. (2023) for the development of a probabilistic earthquake hazard model for Central Asia. Because it is in the plain-text GeoJSON format, it also has the added advantage of being easily maintained and extended using common version control tools (e.g. Git) and GIS (geographic information system) software (e.g. QGIS). However, translating the original AFEAD into the GEM format required a certain amount of interpretation, as not all parameters could be directly assigned. In addition, only a subset of the parameters from GEM are used (for a description of the GEM GAF-DB format, see https://github.com/GEMScienceTools/gem-global-active-faults, last access: 23 July 2024).
The parameter conversion rules are described in Table 10. Note that any parameters not explicitly specified in the conversion table were discarded during compilation. In addition, faults with the missing required parameterization (e.g. unknown value for the parameter SIDE) were not considered and therefore are not currently converted to the source model.
The most sensitive parameter of the conversion process is the net slip rate. The AFEAD provides an approximate and quite wide range of slip rates for each RATE class (1, 2, 3), which we converted to numerical values (in cm yr−1) by comparing them to the slip rates reported in the GEM GAF-DB and from scientific literature. However, to account for the unavoidable uncertainties associated with the conversion, three alternative rate conversion models were implemented, including a middle estimate, an upper bound, and a lower bound, with the goal of using them for hazard calculation in a logic tree structure.
Only faults with reliability classes A and B (independent evidence of activity in the form of kinematics and clear evidence of strong earthquakes) were explicitly considered, while classes C and D were discarded because of their unclear, incomplete, or inaccurate interpretation. This conservative choice could be relaxed in future analyses as additional information becomes available for lineaments from classes C and D. The selected subset consists in 1444 individual fault segments, covering most of the active shallow crust currently affected by seismicity.
This paper presents the creation of the datasets required for the calculation of a new probabilistic seismic hazard model for Central Asia under the SFRARR regional programme (Strengthening Financial Resilience and Accelerating Risk Reduction in Central Asia). The main objective was to create a preparatory collection of data based on the most complete and up-to-date information available for the territory, consistent in its methodological construction and uniform for all Central Asian countries.
The homogenization of input datasets was definitely one of the most critical and challenging steps in the analysis, as the quality, completeness, and reliability of the data required to build the model inevitably varies. Although several homogenization strategies are available (e.g. Weatherill et al., 2016), the most appropriate approach should be determined on a case-by-case basis and only after a critical analysis of the available data and thus cannot be explicitly defined in advance.
In this study, a general-to-specific approach was taken to homogenize each dataset; i.e. an initial structure was defined based on the most uniform and non-conflicting information available across the region, and progressively more granular and detailed information was introduced, selected, and ranked based on its reliability and importance to the hazard assessment. Multiple representations or interpretations of a single element that cannot be resolved by analysis were retained, as in the case of slip rate information for finite-fault modelling. This epistemic variability is then passed on in the hazard calculation to represent uncertainty (using a logic tree or parametric distributions in OpenQuake).
The assembled earthquake catalogue represents an important step toward a holistic analysis of the seismic characteristics of the region. The conversion to the Mw scale greatly simplifies the integration of future data, the compilation of which can be uniformly based on the procedures presented and discussed in detail here. The major limitation of the derived earthquake catalogue is probably its completeness level, the reduction of which is a future priority. However, this can only be done by integrating new data, i.e. by strengthening existing networks, which will also help to refine the selection of appropriate ground motion models for the region and encourage the development of new locally calibrated models. In addition, a revision of historical data, which may currently be subject to large uncertainties, should be endorsed.
As for the active-fault dataset, we started from existing regional compilations (GEM and AFEAD) that were already consistent for the whole area. Nevertheless, it should be noted that targeted studies on individual segments, either from the literature or from local scientific partners of the consortium, were noted with great interest but were not directly included in the initial source model at this time, mainly because of the scientific debate currently going on for some of these lineaments or because of the lack of complete information or the degree of uncertainty involved. Nonetheless, we consider the current selection to be robust enough to represent the major fault systems capable of producing large destructive earthquakes and thus a good starting point for later modifications by integrating local studies at higher resolution.
A well-known problem with this type of study is the long-term sustainability of the data. In this work, we adopted the strategy of making all data and the tools used to generate them freely available on platforms (see “Data availability”) that ensure their continuous accessibility. Our goal is to create dynamic input datasets (i.e. both the earthquake catalogue and the active-fault database) and hazard models that can be easily maintained and later expanded as new information becomes available. Ultimately, the goal is to provide the local scientific community with an aggregator that can be used to foster discussion and subsequently enriched with the results of targeted studies on selected elements that require specific attention.
All data presented in this paper, including the harmonized earthquake catalogue, the active-fault database, the probabilistic seismic hazard analysis (PSHA) source model files in the OpenQuake format, and the corresponding calculation results, are available under the Creative Commons Attribution 4.0 License on the World Bank data portal (https://datacatalog.worldbank.org/search/dataset/0064167/Central-Asia-earthquake-catalogue, World Bank, 2023a and https://datacatalog.worldbank.org/search/dataset/0064168/Central-Asia-seismic-fault-database, World Bank, 2023b) along with the technical reports produced during the SFRARR project.
VP was responsible for coordinating the earthquake hazard component of the SFRARR programme. All co-authors contributed to data collection and review, the model implementation, and the discussion of key results. VP prepared the manuscript with contributions from all co-authors.
The contact author has declared that none of the authors has any competing interests.
Publisher's note: Copernicus Publications remains neutral with regard to jurisdictional claims made in the text, published maps, institutional affiliations, or any other geographical representation in this paper. While Copernicus Publications makes every effort to include appropriate place names, the final responsibility lies with the authors.
The authors of this document are part of an international consortium of experts that has been hired by the World Bank to implement many of the work under the SFRARR programme. This model has been produced with the assistance of the European Union, World Bank, and GFDRR. The sole responsibility of this publication lies with the author and can in no way be taken to reflect the views of these institutions. The European Union, World Bank, and GFDRR are not responsible for any use that may be made of the information contained therein.
This article is part of the special issue “Regionally consistent risk assessment for earthquakes and floods and selective landslide scenario analysis in Central Asia”. It is not associated with a conference.
The Strengthening Financial Resilience and Accelerating Risk Reduction in Central Asia (SFRARR) programme is funded by the European Union, managed by the Global Facility for Disaster Reduction and Recovery (GFDRR), and implemented by the World Bank.
We would like to thank all the project team members; the local partners of the consortium; and the World Bank specialists, in particular Stuart Alexander Fraser and Madina Nizamitdin, for their constructive contribution to the project.
This research has been supported by the World Bank Group (consulting services contract no. 8006611 – Regionally consistent risk assessment for earthquakes and floods and selective landslide scenario analysis for strengthening financial resilience and accelerating risk reduction in Central Asia).
This paper was edited by Filippos Vallianatos and reviewed by Elif Oral and one anonymous referee.
Abdrakhmatov, K.: Establishment of the Central Asia Seismic Risk Initiative (CASRI), ISTC Project No. KR 1176, 2009, Technical Report on the Work Performed from: 1 Febbruary 2006 to 30 April 2009, Institute of Seismology, National Academia of Sciences, Bishkek, Kyrgyzstan, 2009.
Abdrakhmatov, K., Havenith, H. B., Delvaux, D., Jongmans, D., and Trefois, P.: Probabilistic PGA and Arias Intensity maps of Kyrgyzstan (Central Asia), J. Seismol., 7, 203–220, https://doi.org/10.1023/A:1023559932255, 2003.
Abdrakhmatov, K. Y., Aldazhanov, S. A., Hager, B. H., Hamburger, M. W., Herring, T. A., Kalabaev, K. B., Makarov, V. I., Molnar, P., Panasyuk, S. V, Prilepin, M. T., Reilinger, R. E., Sadybakasov, I. S., Souter, B. J., Trapeznikov, Y. A., Tsurkov, V. Y., and Zubovich, A. V.: Relatively recent construction of the Tien Shan inferred from GPS measurements of present-day crustal deformation rates, Nature, 384, 450–453, 1996.
Aki, K. and Richards, P. G.: Quantitative Seismology, Theory and Methods, in: Vol. I and II, 1st Edn., W. H. Freeman, San Francisco, 557 pp., ISBN 10:0716710587, ISBN 13:978-0716710585, 1980.
Albini, P., Musson, R. M. W., Rovida, A., Locati, M., Gomez Capera, A. A., and Viganò, D.: The global earthquake history, Earthq. Spectra, 30, 607–624, 2014.
Artikov, T. U., Ibragimov, R. S., Ibragimova, T. L., Kuchkarov, K., and Mirzaev, M. A.: Quantitative assessment of seismic hazard for the territory of Uzbekistan according to the estimated maximum ground oscillation rates and their spectral amplitudes, Geodyn. Tectonophys., 9, 1173–1188, 2018.
Bachmanov, D. M., Kozhurin, A. I., and Trifonov, V. G.: The active faults of Eurasia database, Geodyn. Tectonophys., 8, 711–736, 2017.
Bindi, D., Parolai, S., Oth, A., Abdrakhmatov, K., Muraliev, A., and Zschau, J.: Intensity prediction equations for Central Asia, Geophys. J. Int., 187, 327–337, 2011.
Bindi, D., Abdrakhmatov, K., Parolai, S., Mucciarelli, M., Grünthal, G., Ischuk, A., Mikhailova, N., and Zschau, J.: Seismic hazard assessment in Central Asia: Outcomes from a site approach, Soil Dynam. Earthq. Eng., 37, 84–91, 2012.
Bondár, I., Engdahl, E. R., Villaseñor, A., Harris, J., and Storchak, D.: ISC-GEM: Global Instrumental Earthquake Catalog (1900–2009), II. Location and seismicity patterns, Phys. Earth Planet. Inter., 239, 2–13, https://doi.org/10.1016/j.pepi.2014.06.002, 2015.
Danciu, L., Şeşetyan, K., Demircioglu, M., Gülen, L., Zare, M., Basili, R., Elias, A., Adamia, S., Tsereteli, N., Yalçın, H., Utkucu, M., Khan, M. A., Sayab, M., Hessami, K., Rovida, A. N., Stucchi, M., Burg, J-P., Karakhanian, A., Babayan, H., Avanesyan, M., Mammadli, T., Al-Qaryouti, M., Kalafat, D., Varazanashvili, O., Erdik, M., and Giardini, D.: The 2014 Earthquake Model of the Middle East: seismogenic sources, Bull. Earthq. Eng., 16, 3465–3496, https://doi.org/10.1007/s10518-017-0096-8, 2017.
Di Giacomo, D., Bondár, I., Storchak, D., Engdahl, E. R., Bormann, P., and Harris, J.: ISC-GEM: global instrumental earthquake catalog (1900–2009), III. Re-computed MS and mb, proxy MW, final magnitude composition and completeness assessment, Phys. Earth Planet. Inter., 239, 33–47, 2015.
Di Giacomo, D., Engdahl, E. R., and Storchak, D. A.: The ISC-GEM Earthquake Catalogue (1904–2014): status after the Extension Project, Earth Syst. Sci. Data, 10, 1877–1899, https://doi.org/10.5194/essd-10-1877-2018, 2018.
Edwards, B., Allmann, B., Fäh, D., and Clinton, J.: Automatic computation of moment magnitudes for small earthquakes and the scaling of local to moment magnitude, Geophys, J. Int., 183, 407–420, 2010.
Ekstrom, G., Nettles, M., and Dziewonski, A. M.: The global CMT project 2004–2010: centroid-moment tensors for 13,017 earthquakes, Phys. Earth Planet. Inter., 200–201, 1–9, 2012.
Free, M., Coates, K., Grant, D., Fourniadis, Y., Ader, T., Sousa, L., Fleming, K., Pittore, M., Moldobekov, B., and Ormukov, C.: Seismic Risk in the Kyrgyz Republic, Central Asia, in: 16th European Conference on Earthquake Engineering, 18–21 June 2018, Thessaloniki, Greece, The link to the website (discontinued) of the conference is: https://www.researchgate.net/publication/327581942_Seismic_Risk_in_the_Kyrgyz_Republic_Central_Asia (last access: July 2024), 2018.
Gardner, J. K. and Knopoff, L.: Is the sequence of earthquakes in Southern California, with aftershocks removed, Poissonian?, Bull. Seismol. Soc. Am., 64, 1363–1367, 1974.
Giardini, D. (Ed.): The global seismic hazard assessment program 1992–1999, Annali Geofis., 42, 1225–1230, https://doi.org/10.4401/ag-3780, 1999.
Gómez-Novell, O., García-Mayordomo, J., Ortuño, M., Masana, E., and Chartier, T.: Fault System-Based Probabilistic Seismic Hazard Assessment of a Moderate Seismicity Region: The Eastern Betics Shear Zone (SE Spain), Front. Earth Sci., 8, 579398, https://doi.org/10.3389/feart.2020.579398, 2020.
Grünthal, G.: The up-dated earthquake catalog for the German Democratic Republic and adjacent areas – Statistical data characteristics and conclusions for hazard assessment, in: Proceedings of the 3rd International Symposium on the Analysis of Seismicity and Seismic Risk, Liblice Castle, Czechoslovaki, Geophysical Institute of the Czechoslovak Academy of Sciences, 17–22 June 1985, Prague, Czech Republic, https://gfzpublic.gfz-potsdam.de/pubman/item/item_232591 (last access: July 2024), 1985.
Hanks, T. C. and Kanamori, H.: A moment magnitude scale, J. Geophys. Res., 84, 2348–2350, 1979.
Ischuk, A., Bjerrum, L. W., Kamchybekov, M., Abdrakhmatov, K., and Lindholm, C.: Probabilistic Seismic Hazard Assessment for the Area of Kyrgyzstan, Tajikistan, and Eastern Uzbekistan, Central Asia, Bull. Seismol. Soc. Am., 108, 130–144, 2018.
Ischuk, A. R. and Mamadjanov, Y.: Seismicity and seismic hazard of the Territory of Tajikistan, in: Earth Reality Along The Silk Road And Scientific Cooperation, 6th Urbanization Convention of Union of Architects and Engineers of the Turkic World, 3–7 September 2014, Bishkek, Kyrgyzstan, Ataturk University, Erzurum, 132 pp., https://www.kitantik.com/product/EARTHQUAKE-REALITY-ALONG-THE-SILK-ROAD-AND (last access: July 2024), 2014.
King, S. A., Khalturin, V. I., and Tucker, B. E.: Seismic Hazard and Building Vulnerability in Post-Soviet Central Asian Republics, in: '94 Proceedings of the NATO Advanced Research Workshop on Earthquake Risk Management Strategies for Post-Soviet Central Asian Republics: Avoiding Repetition of 1988 a Shakhalin Disasters, 22–25 October 1996, Almaty, Kazakhstan, https://doi.org/10.1007/978-94-017-2971-0, 1999.
Leonard, M.: Self-consistent earthquake fault-scaling relations: update and extension to stable continental strike-slip faults, Bull. Seismol. Soc. Am., 104, 1971–1988, 2014.
Mikhailova, N. N., Mukambayev, A. S., Aristova, I. L., Kulikova, G., Ullah, S., Pilz, M., and Bindi, D.: Central Asia earthquake catalog from ancient time to 2009, Ann. Geophys., 58, S0102, https://doi.org/10.4401/ag-6681, 2015.
Mosca, I., Baptie, B., Sargeant, S., and Walker, R. T.: Integrating Outcomes from Probabilistic and Deterministic Seismic Hazard Analysis in the Tien Shan, Bull. Seismol. Soc. Am., 109, 688–715, 2019.
Pagani, M., Monelli, D., Weatherill, G., Danciu, L., Crowley, H., Silva, V., Henshaw, P., Butler, L., Nastasi, M., Panzeri, L., Simionato, M., and Vigano, D.: OpenQuake-engine: an open hazard (and risk) software for the global earthquake model, Seismol. Res. Lett., 85, 692–702, 2014a.
Pagani, M., Monelli, D., Weatherill, G. A., and Garcia, J.: The OpenQuake-engine Book: Hazard, Global Earthquake Model (GEM) Technical Report 2014-08, 67 pp., https://cloud-storage.globalquakemodel.org/public/wix-new-website/pdf-collections-wix/publications/OQ Hazard Science 1.0.pdf (last access: July 2024), 2014b.
Poggi, V., Garcia-Peláez, J., Styron, R., Pagani, M., and Gee, R.: A probabilistic seismic hazard model for North Africa, Bull Earthq. Eng., 18, 2917–2951, https://doi.org/10.1007/s10518-020-00820-4, 2020.
Poggi, V., Parolai, S., Silacheva, N., Ischuk, A., Abdrakhmatov, K., Kobuliev, Z., Ismailov, V., Ibragimov, R., Karayev, J., Ceresa, P., Santulin, M., and Bazzurro, P.: Development of a regional probabilistic seismic hazard model for Central Asia, Nat. Hazards Earth Syst. Sci. Discuss. [preprint], https://doi.org/10.5194/nhess-2023-132, in review, 2023.
Rautian, T., Khalturin, K., Fujita, K., Mackey, G., and Kendall, A. D.: Origins and methodology of the Russian Energy K-Class System and its relationship to magnitude scales, Seismol. Res. Lett., 78, 579– 590, 2007.
Silacheva, N. V., Kulbayeva, U. K., and Kravchenko, N. A.: Probabilistic seismic hazard assessment of Kazakhstan and Almaty city in peak ground accelerations, Geod. Geodynam., 9, 131–141, 2018.
Storchak, D. A., Di Giacomo, D., Bondár, I., Engdahl, E. R., Harris, J., Lee, W. H. K., Villaseñor, A., and Bormann, P.: Public Release of the ISC-GEM Global Instrumental Earthquake Catalog (1900–2009), Seismol. Res. Lett., 84, 810–815, 2013.
Storchak, D. A., Di Giacomo, D., Engdahl, E. R., Harris, J., Bondár, I., Lee, W. H. K., Bormann, P., and Villaseñor, A.: The ISC-GEM global instrumental earthquake catalog (1900–2009), Phys. Earth. Planet. Inter., 239, 48–63, 2015.
Storchak, D. A., Harris, J., Brown, L., Lieser, K., Shumba, B., Verney, R., Di Giacomo, D., and Korger, E. I. M.: Rebuild of the Bulletin of the International Seismological Centre (ISC), part 1: 1964–1979, Geosci. Lett., 4, 32, https://doi.org/10.1186/s40562-017-0098-z, 2017.
Styron, R. and Pagani, M.: The GEM Global Active Faults Database, Earthq. Spectra, 36, 160–180, https://doi.org/10.1177/8755293020944182, 2020.
Uhrhammer, R.: Characteristics of Northern and Central California seismicity, Earthq. Notes, 57, 21–37, https://doi.org/10.1130/dnag-csms-neo.99, 1986.
Ullah, S., Bindi, D., Pilz, M., Danciu, L., Weatherill, G., Zuccolo, E., Ischuk, A., Mikhailova, N. N., Abdrakhmatov, K., and Parolai, S.: Probabilistic seismic hazard assessment for Central Asia, Ann. Geophys., 58, S0103, https://doi.org/10.4401/ag-6687, 2015.
Ulomov, V. I. and The GSHAP Region 7 Working Group: Seismic hazard of Northern Eurasia, Ann. Geophys., 42, 1023–1038, https://doi.org/10.4401/ag-3785, 1999.
Valentini, A., Pace, B., Boncio, P., Visini, F., Pagliaroli, A., and Pergalani, F.: Definition of Seismic Input From Fault-Based PSHA: Remarks After the 2016 Central Italy Earthquake Sequence, Tectonics, 38, 2, https://doi.org/10.1029/2018TC005086, 2019.
Weatherill, G. A., Pagani, M., and Garcia, J.: Exploring earthquake databases for the creation of magnitude-homogeneous catalogs: tools for application on a regional and global scale, Geophys. J. Int., 206, 1652–1676, 2016.
World Bank: Central Asia Earthquake Catalogue, World Bank [data set], https://datacatalog.worldbank.org/search/dataset/0064167/Central-Asia-earthquake-catalogue (last access: July 2024), 2023a
World Bank: Central Asia Seismic Fault Database, World Bank [data set], https://datacatalog.worldbank.org/search/dataset/0064168/Central-Asia-seismic-fault-database (last access: July 2024), 2023b
Zelenin, E., Bachmanov, D., Garipova, S., Trifonov, V., and Kozhurin, A.: The Active Faults of Eurasia Database (AFEAD): the ontology and design behind the continental-scale dataset, Earth Syst. Sci. Data, 14, 4489–4503, https://doi.org/10.5194/essd-14-4489-2022, 2022.
Zubovich, A. V., Wang, X., Scherba, Y. G., Schelochkov, G. G., Reilinger, R., Reigber, C., Mosienko, O.I., Molnar, P., Michajljow, W., Makarov, V. I., Li, J., Kuzikov, S. I., Herring, T. A., Hamburger, M. W., Hager, B. H., Dang, Y., Bragin, V. D., and Beisenbaev, R. T.: GPS velocity field for the Tien Shan and surrounding regions, Tectonics, 29, TC6014, https://doi.org/10.1029/2010TC002772, 2010.