the Creative Commons Attribution 4.0 License.
the Creative Commons Attribution 4.0 License.
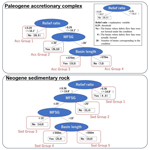
Morphological characteristics and conditions of drainage basins contributing to the formation of debris flow fans: an examination of regions with different rock strength using decision tree analysis
Ken'ichi Koshimizu
Satoshi Ishimaru
Fumitoshi Imaizumi
Gentaro Kawakami
Debris flows cause severe disasters that can result in human casualties and the collapse of houses. The establishment of early warning systems in basins with high debris flow risks is needed to reduce the negative impacts of debris flow disasters. Because debris flows often form debris flow fans near the mouths of valleys, debris flow fans are regarded as important topographical elements that indicate the occurrence of debris flows. The presence or absence of a debris flow fan makes it possible to clarify the morphological conditions of the contributing area that has generated debris flows. These morphological conditions may depend on rock strength, which controls the weathering activity and grain size of sediments. In this study, we investigated the morphological conditions of a drainage basin that contribute to the formation of debris flow fans using decision tree analysis. The analysis was conducted at two sites with clear differences in rock strength due to geological processes: Neogene sedimentary rock and Paleogene accretionary complex sites. As a result of decision tree analysis using data sets containing a total of 158 basins, the thresholds of morphological parameters needed for forming debris flow fans differed depending on the geological features. When the relief ratio was less than 0.29 at the Paleogene accretionary complex site, coarse-grained sediments were less likely to pass out of the valley, resulting in the absence of debris flow fans. On the other hand, at Neogene sedimentary rock sites, short basins were determined to form debris flow fans, even if the relief ratio was less than 0.36, because the sediments were fine-grained and tended to flow downstream. In contrast, morphological factors that influence the presence or absence of debris flow fans were common at both sites. The first, second, and third most important morphological factors were the relief ratio, most frequent slope gradient, and basin length, respectively. Therefore, these morphological factors are considered important in evaluating debris flow risks. This study demonstrates that the decision tree analysis is an effective tool for determining the hierarchy and threshold of morphological factors that classify the presence or absence of debris flows that reach valley mouths.
- Article
(8477 KB) - Full-text XML
- BibTeX
- EndNote
Debris flows cause severe disasters that can result in human casualties, the collapse of houses, and floods owing to the large sediment volume, high kinematic energy, and significant deposition of large numbers of sediments (Dowling and Santi, 2014; Badoux et al., 2016). The establishment of early warning systems in basins with high debris flow risks is needed to reduce the impacts of debris flow disasters. Because debris flows often form debris flow fans near the mouths of incised valleys, debris flow fans are regarded as critical topographical elements that indicate the occurrence of debris flows (De Haas et al., 2015, 2018). Therefore, the presence or absence of a debris flow fan makes it possible to identify the topographic conditions of the contributing area that generated debris flows. These topographic conditions can be used to evaluate debris flow risks before conducting detailed field surveys.
Many studies have been conducted to clarify the relationship between the formation of fans and the morphological features of drainage basins (Guzzetti et al., 1997; De Scally and Owens, 2004). Other studies have classified debris flow basins and debris flood basins based on the morphological features of drainage basins and fans (Wilford et al., 2004; Ilinca, 2021).
Morphological factors of drainage basins that affect fan formation include the basin area, maximum basin length, basin relief, slope gradient, and relief ratio in the maximum catchment length direction (Meyer and Wells, 1997; De Scally and Owens, 2004; Wilford et al., 2004; Rowbotham et al., 2005; Zhou et al., 2016). Basin area is a factor that affects sediment supply activity (De Scally and Owens, 2004). There is a positive correlation between the basin and fan areas (Melton, 1965; Guzzetti et al., 1997; Giles, 2010; Benvenuti et al., 2016) and a strong negative correlation between the basin area and fan gradient (Gómez-Villar and García-Ruiz, 2000; Crosta and Frattini, 2004; De Scally and Owens, 2004; De Haas et al., 2015). Debris flows terminate before the valley mouth more easily if the maximum basin length above the valley mouth is greater (Wilford et al., 2004). The amount of uplift affects the basin relief; i.e., erosion and sediment supply are more active in basins with higher basin relief (Zhou et al., 2016). The slope gradient describes the steepness of the overall slope in the basin and is a factor in controlling sediment supply activity (Rowbotham et al., 2005). The relief ratio, the channel gradient along the direction of maximum basin length, represents morphological conditions that promote sediment supply and control the sediment delivery ratio outside of the valley (Meyer and Wells, 1997).
The morphological features of drainage basins have also been used to determine the types of sediment transfer that form fans (Crosta and Frattini, 2004; Wilford et al., 2004; De Scally et al., 2010; Ilinca, 2021). For example, Wilford et al. (2004) reported that debris flow and debris flood could be effectively discriminated from a combination of the Melton ratio (basin relief divided by the square root of the basin area) and basin length. In addition, De Scally et al. (2010) pointed out that the ruggedness of the basin (Melton ratio and relief ratio along the maximum catchment length direction) and fan gradient (e.g., the gradient in the upper part and average gradient) are important morphological factors for distinguishing between debris flow deposits and debris flood deposits. However, the thresholds dividing the two processes differ depending on the geology (De Scally et al., 2010; Ilinca, 2021). Crosta and Frattini (2004) also classified debris flows and debris floods based on morphological conditions in the drainage basin using the discriminant analysis and logistic regression analysis. They reported that some debris flood basins were misclassified as debris flow basins owing to the differences in the erodibility on the bedrock. In other words, the dominant types of sediment movement processes differ depending on the difference in geology. Therefore, it is necessary to determine the morphological conditions of debris flow occurrence in each geological setting. However, the importance and threshold of morphological factors within drainage basins in forming debris flow fans have only been investigated in a few regions (Crosta and Frattini, 2004; De Scally et al., 2010; Ilinca, 2021). In addition, the commonalities among different geologies have not yet been sufficiently determined. These are important for improving the accuracy of debris flow risk evaluation.
In recent years, various machine-learning analyses have been performed to identify the factors that cause natural disasters. Decision tree analysis is machine learning that uses a tree structure to explain the estimation procedure and order of important explanatory variables from a large data set (Witten and Frank, 2005). Therefore, it is useful to clarify the morphological conditions contributing to debris flows and landslides (e.g., Saito et al., 2009; Zhang et al., 2019). If the presence or absence of debris flow fans and morphological factors of the drainage basin are used as the objective and explanatory variables, respectively, it is possible to determine the morphological conditions contributing to the formation of debris flow fans in each geology. Furthermore, considering the morphological conditions of the drainage basin forming debris flow fans as the conditions generating debris flows, the risk of debris flow occurrence can be evaluated even in basins where debris flow fans have disappeared owing to the effects of erosion by mainstream rivers and artificial land formation.
The purpose of this study is to identify the morphological conditions of basins that contribute to the formation of debris flow fans at sites with different geology. In this study, we selected the Neogene sedimentary rock and the Paleogene accretionary complex (trench-fill turbidites) sites in Hokkaido, northern Japan. These sites have significantly different rock strengths due to rock formation processes. In addition, based on decision tree analysis and field surveys, we identified the commonalities and differences in morphological conditions that affect the formation of debris flow fans due to differences in the rock strength. Because many debris flow fans remain without significant artificial modification at the study sites, it is easy to evaluate the differences in the formation conditions of the debris flow fan between the two geological properties. We have selected decision tree analysis because it can objectively provide a hierarchy and threshold of morphological factors that classify the presence or absence of debris flow fans.
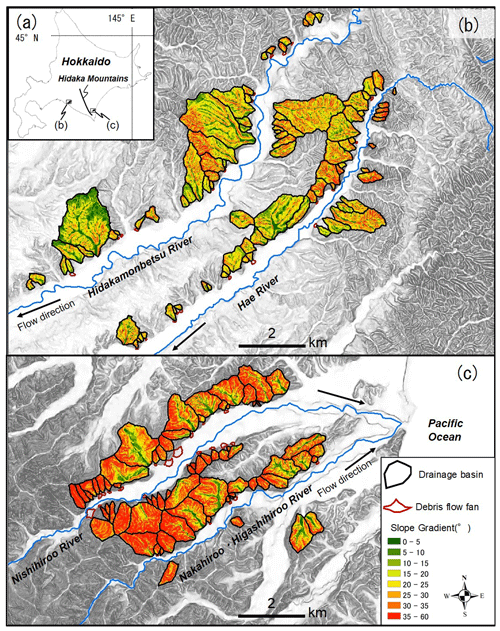
Figure 1Map of the study sites. (a) Location of study sites is indicated by black areas. (b) Slope gradient of drainage basins at the Neogene sedimentary rock site. (c) Slope gradient of drainage basins at the Paleogene accretionary complex site. Shaded areas in the background indicate steepness of topography.
The study sites were located in the southeastern and western parts of the Hidaka Mountains in Hokkaido, northern Japan (Fig. 1a). The geology of the study sites consists of two geologies: Neogene sedimentary rock at the western site with an area of 161 km2 (Fig. 1b) and a Paleogene accretionary complex at the southeastern site with an area of 59 km2 (Fig. 1c). The geological settings at the study sites were assessed using the 1:200 000 seamless digital geological map v2 (Geological Survey of Japan, 2022). Climate conditions may not clearly affect differences in sediment transfer processes between the two sites because they are located in the same region.
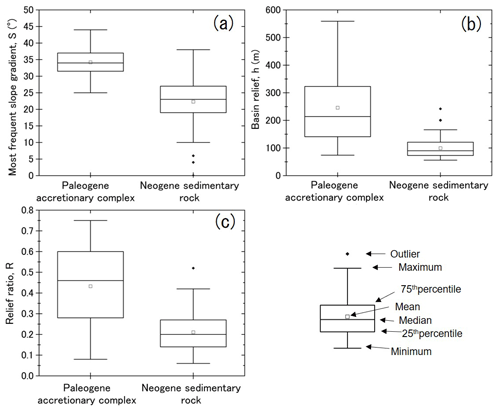
Figure 2Topographic properties of each drainage basin at the Paleogene accretionary complex site and the Neogene sedimentary rock site: (a) most frequent slope gradient, (b) basin relief, (c) relief ratio.
The Paleogene accretionary complex site located southeast of the Hidaka Mountains corresponds to the Nakanogawa group (coherent facies) (Nanayama, 1992) and is mainly composed of sandstone and slate (Suzuki et al., 1959). In contrast, the Neogene sedimentary rock at the western study site consists mainly of diatomaceous siltstone, sandstone, and conglomerate of the Nina Formation (Imai and Sumi, 1957). According to field surveys, the rocks of the western study area (Neogene sedimentary rock site) were underlain by soft rocks highly slaked by weathering. On the other hand, the rocks of the southeastern study area (the Paleogene accretionary complex site) were found to be hard rocks without evidence of significant weathering. The unconfined compressive strength of Neogene sedimentary rock is less than 7.4 MPa across all lithologies. In contrast, sandstone and slate of the Paleogene accretionary complex exhibit strengths ranging from 7.4 to 64.8 MPa. Although rock strength varies even in the same geological unit due to differences in accretion and metamorphic grade (Moore and Saffer, 2001), the rock strength at Paleogene accretionary complex site tends to be higher than that at the Neogene sedimentary rock site. In addition, based on the field survey of slope failures, the grain size of the deposits from slope failures at the Neogene sedimentary rock site was much finer than that at the Paleogene accretionary complex site.
At the Paleogene accretionary complex site, three large rivers (Nishihiroo, Nakahiroo, and Higashihiroo) flow from the southwest to the northeast (Fig. 1c). Small valleys of tributaries (hereafter drainage basins) are arranged along these rivers. The drainage basins of analyzed valleys range from 8300 to 1 220 000 m2. At the Neogene sedimentary rock site, the Hae and Hidakamonbetsu rivers flow from the northeast to the southwest (Fig. 1b). Drainage basins (which were analyzed in this study) with areas of 12 000 to 2 110 000 m2 are arranged along the two rivers. At both study sites, there were many valleys with and without debris flow fans at their mouths.
The Paleogene accretionary complex site has a steeper topography than the Neogene sedimentary rock site (Figs. 1b, c, and 2a). The minimum slope gradient of the Paleogene accretionary complex site approximately corresponds to the median slope gradient of the Neogene sedimentary rock site (Fig. 2a). The basin reliefs of the Paleogene accretionary complex site were often larger than those of the Neogene sedimentary rock site (Fig. 2b). In addition, these basin reliefs range from small to large, and the variation is greater than that at the Neogene sedimentary rock site (Fig. 2b). Many of the relief ratios at the Paleogene accretionary complex sites are larger than those at the Neogene sedimentary rock sites (Fig. 2c). In addition, these relief ratios ranged from small to large, and the variation was greater than that at the Neogene sedimentary rock site (Fig. 2c). Furthermore, the slope gradient maps show that gentle slopes, which are not affected by incision by channel networks, are more widely distributed at the Neogene sedimentary rock site than at the Paleogene accretionary complex site (Fig. 1b and c). Although rock strength would have a large variation even in a same geology, differences in the topographic features between the two study sites indicate that the rock strength is significantly different between the two sites.
The uplifting rate in the Late Quaternary at Paleogene accretionary complex site (0.1 mm yr−1) is lower than that at the Neogene sedimentary rock site (0.5 mm yr−1) (Yoshiyama, 1990). Most of the debris flow fans have been formed on valley plains or Holocene terraces in the studied area. In other words, the target debris flow fans have been constructed during the Holocene with warm and wet climates similar to the current conditions.
Geographic Information System (GIS) and decision tree analyses were performed according to the flowchart shown in Fig. 3. First, debris flow fans and the boundaries of drainage basins at the study sites were delineated using published topographic maps and digital elevation models (DEMs). After calculating the morphological parameters in each basin, a decision tree analysis was performed using the presence or absence of debris flow fans as the objective variable and the morphological variables of the drainage basin as the explanatory variables. The morphological properties of the drainage basins were calculated using ArcGIS 10.8 (Esri Inc.). DEMs with a grid size of 10 m built from the topographic map of the Geospatial Information Authority of Japan (1:25 000 topographic map) were used to calculate the morphological variables.
Field surveys were conducted in 18 and 8 basins in the areas of Paleogene accretionary complex (field surveys from 17–18 November 2021) and Neogene sedimentary rock (field surveys from 15–16 November 2021), respectively, to validate the extraction of debris flow fans from topographic maps. The characteristics of the basins, such as grain size distribution, debris flow history, and morphology of fan deposits, were also assessed by field surveys.
3.1 Selection of the range of the drainage basins
The boundary of the drainage basins at each study site was extracted from 10 m grid DEMs using the Spatial Analyst tool of ArcGIS 10.8. The lower end of the drainage basins was set at the valley mouth, where the narrow valley-shaped topography formed by tributaries changes to a wide and flat terrain affected by the fluvial processes of the main rivers. The basin boundaries automatically extracted from the DEMs were checked by overlapping with 1:25 000 topographic maps of the Geospatial Information Authority of Japan using GIS software. Basins with an area of 8 000 m2 or less have a topography that resembles a hillslope more than a watershed. In addition, many of these small basins have talus slopes, rather than debris flow fans, in their lower parts. Hence, the lower limit of the basin area analyzed in this study was set to 8 000 m2.
3.2 Extraction of debris flow fans
Because this study focuses on debris flow fans, fan-shaped sedimentary landforms near the valley mouth, which have a slope gradient similar to common debris flow fans, were extracted from 1:25 000 topographic maps using GIS. De Haas et al. (2018) found that the slope gradients of debris flow fans determined by field observations are often greater than 5°. Takase et al. (2002) reported that the slope gradient of debris flow fans in Japan generally ranges from 5 to 22°. Fan-shaped topography gentler than this gradient range is likely affected by debris flooding, whereas topography steeper than this range is possibly a talus cone. For this reason, we extracted fan-shaped sedimentary topographies, which have a main-axis gradient of 5–22°, as debris flow fans. It was difficult to extract small-scale debris flow fans with a relative height of less than the contour interval (10 m) in this analysis. Hence, we analyzed debris flow fans exceeding 10 m.
3.3 Morphological variables of drainage basins
Six morphological properties in drainage basins that potentially contribute to the formation of debris flow fans were selected based on previous studies (Meyer and Wells, 1997; De Scally and Owens, 2004; Wilford et al., 2004; Rowbotham et al., 2005; Zhou et al., 2016) and field surveys. The six selected morphological properties were the following: (1) basin area, (2) basin length, (3) basin relief, (4) most frequent slope gradient (hereafter MFSG), (5) relief ratio, and (6) channel gradient within 100 m upstream from the valley mouth (hereafter channel gradient) (Fig. 4). The properties were calculated from the 10 m grid DEMs using ArcGIS 10.8.
The basin area was calculated using a geometric calculation tool in ArcGIS. The basin length was defined as the straight horizontal distance from the valley mouth to the furthest point in the basin. The basin length was likely to be shorter than the actual distance because the curve of the channel was ignored. Basin relief was obtained from the difference between the highest and lowest elevations. The MFSG was the highest frequency of integer slope gradient classes with an interval of 5°, calculated using the zonal statistic tool of ArcGIS. In this study, the class “5 degrees” indicated the slope gradient range from 1 to 5°, whereas the class “10 degrees” indicated 6 to 10°. Because the analysis was performed with a 10 m grid, steep microtopography smaller than the grid size (e.g., landslide scarps and channel banks) was classified into the gentler gradient class. The relief ratio was calculated as the relative height divided by the maximum horizontal basin length. The relative height used in the calculation of the relief ratio was the difference in altitude between the highest and lowest points along the maximum basin length. The channel gradient above the valley mouth was the relative height from the valley mouth to 100 m upstream, divided by 100 m. We assume this channel gradient affected the sediment transfer type at the valley mouth. If the length of the section for calculating this channel gradient is shorter than 100 m, the influence of local topographies, such as step pools, becomes significant. In addition, if the length of the section was longer, the middle channel reaches of the valley were included in the calculation section for some small basins. Therefore, 100 m was considered appropriate for calculating the channel gradient. Because the width of streams is usually smaller than the grid size of DEMs (10 m), part of the calculated channel gradient may be affected by the valley-side slopes. Because the DEM was created from the 10 m interval contour lines, the maximum error in the elevation value used for calculating morphological properties was approximately 10 m.
3.4 Decision tree analysis
Decision tree analyses with the presence or absence of debris flow fans as the objective variable and the morphological properties of the drainage basin as the explanatory variable were conducted using Weka 3.9.5, an open-source data mining tool developed mainly by Waikato University (Witten and Frank, 2005; The University of Waikato, 2008). The J48 algorithm, a re-implementation of the C4.5 algorithm (Witten and Frank, 2005), was used to construct the tree structure. Based on the decision trees obtained at the Paleogene accretionary complex site and the Neogene sedimentary rock site, we discuss the morphological conditions in the drainage basin that contribute to the formation of debris flow fans. Variables in the higher-order tree structure are considered more important (Pal and Mather, 2003; Witten and Frank, 2005; Bou Kheir et al., 2008; Schneevoigt et al., 2008).
In the topographic map analysis, it was impossible to determine whether debris flow fans were lost because of erosion by the main rivers. In addition, the minimum distance from the fan apex (the drainage basin mouth) to the fan toe of the debris flow fan at the study sites was approximately 50 m. Therefore, if the distance from the valley mouth to the main river is less than 50 m, erosion by the main rivers may affect the presence or absence of debris flow fans. For this reason, basins were excluded from the analysis if the distance from the valley mouth to the river was less than 50 m.
4.1 Paleogene accretionary complex site
4.1.1 Field survey
Field surveys revealed almost no fan-shaped topography with a slope gradient of gentler than 5° (debris flood fans) near the drainage basin mouth, whereas many fans with a slope gradient steeper than 5° (debris flow fans) were found (Fig. 5a). Debris flow fans are primarily composed of stony debris flow deposits. Most debris flow fans and channel deposits in the valley were not covered by vegetation (Fig. 5b). Recent debris flow deposits that were not fully covered by vegetation were found on debris flow fans (Fig. 6a and b). Therefore, erosion and deposition of sediments by debris flows still occur repetitively. As a result of photographic analysis of the grain size within a 1 m grid on the debris flow deposits, more than 95 % were composed of cobbles (maximum particle size of 24 cm). In many valleys, slope failures occur on rocky slopes with well-developed joints (joint spacing of 5–25 cm) which continue to produce sediments. Large gravel with a diameter exceeding 1 m was produced from these rocky slopes.
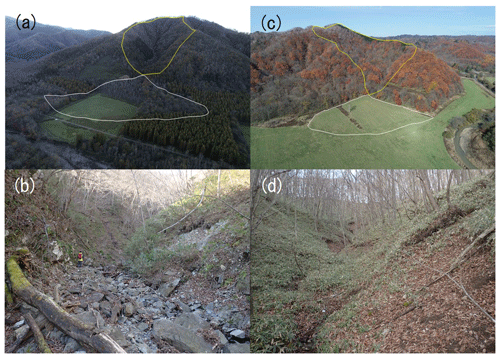
Figure 5Debris flow fans and the channel of the drainage basins at both sites. (a) A debris flow fan at the Paleogene accretionary complex site. Solid white and yellow lines are the debris flow fan and drainage basin boundaries, respectively. (b) Channel condition of the drainage basins at the Paleogene accretionary complex site. (c) A debris flow fan at the Neogene sedimentary rock site. Solid white and yellow lines are the debris flow fan and drainage basin boundaries, respectively. (d) Channel condition of the drainage basins at the Neogene sedimentary rock site.
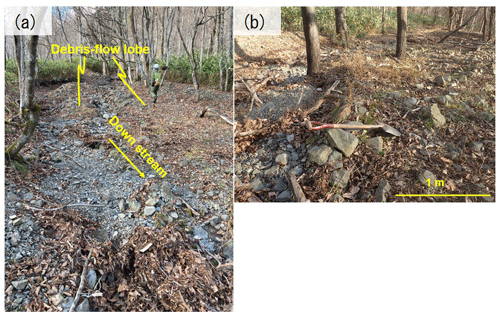
Figure 6Recent debris flow deposits on a debris flow fan of the Paleogene accretionary complex site: (a) debris flow lobe, (b) boulders entrained by debris flows.
The presence or absence of debris flow fans estimated by topographic map analysis was demonstrated by field surveys in 14 basins (78 % of 18 surveyed basins). All four basins wrongly classified by topographic map analysis were missing debris flow fans on the topographic maps but were identified by the field survey. Field surveys in these four basins revealed that fluvial processes from the main river partially eroded the debris flow fans.
4.1.2 Decision tree analysis
A total of 68 drainage basins were extracted using the GIS analysis. As a result of the topographic map interpretation, the numbers of basins with and without debris flow fans were 26 and 42, respectively. The decision tree was composed of three variables and four leaves (Fig. 7). The decision tree analysis successfully classified the presence or absence of debris flow fans in 53 basins (78 % of the 68 analyzed basins). The results of the decision tree analysis differed from those of the topographic map interpretation in 15 basins (hereafter, incorrect basins).
The relief ratio was the most important morphological factor (top hierarchy factor). The first decision indicates that debris flow fans were not formed under the condition of a relief ratio ≤ 0.29 (16.1°) (Acc Group 1 in Fig. 7). In the basins of Acc Group 1, the channel above the valley mouth was gentler than that at 5°. Field surveys have determined that debris flows terminate before reaching the valley mouth. The second most important morphological factor was MFSG. The second decision indicates that debris flow fans were formed under the conditions of relief ratio > 0.29 and MFSG ≤ 35° (Acc Group 2 in Fig. 7). Field surveys of the Acc Group 2 basins revealed that the channel deposits in these basins were poorly covered by vegetation (Fig. 5b). In addition, recent debris flow deposits have been identified in debris flow fans (Fig. 6a and b). According to the GIS analysis, the upper part of the basins with an MFSG ≤ 35° did not experience significant downward dissection. Of the 26 basins classified into Acc Group 2, 10 were incorrect basins, and debris flow fans were not identified in the topographic maps. In these incorrect basins, the channel gradient above the valley mouth was less than 5°. The basin length was the third most important morphological factor (the lowest hierarchy factor). The third decision indicates that debris flow fans were formed under the conditions of a relief ratio > 0.29, MFSG > 35°, and basin length ≤ 570 m (Acc Group 3 in Fig. 7). The downward dissection was apparent in the upper part of the basins classified as Acc Group 3. Five basins in Acc Group 3 (15 basins in total) were incorrect basins in which debris flow fans were not identified in the topographic maps. The formation of a stream channel was presumed based on the shape of the contours around the fan apex of the incorrect basins. Field surveys have revealed that this was a debris flow fan eroded by fluvial processes. The fourth decision indicates that debris flow fans were not formed under the conditions of a relief ratio > 0.29, MFSG > 35°, and basin length > 570 m (Acc Group 4 in Fig. 7).
4.2 Neogene sedimentary rock site
4.2.1 Field survey
Field surveys revealed that the number of fan-shaped topographies with a slope gradient of less than 5° at the Neogene sedimentary rock site was higher than that of the Paleogene accretionary complex site. Many of these field surveys found no micro-landforms, such as debris flow snouts or lateral levees. There was only a little gravel on the surfaces of the fans. Additionally, a stratified structure was identified in the cross-sectional plane of the fan in some basins. Therefore, these gentle fan-shaped topographies were likely formed by fluvial processes such as bed load and suspended sediment. Unlike the Paleogene accretionary complex site, most riverbed sediments and debris flow fans at the Neogene sedimentary rock site were covered with vegetation. Recent erosion and deposition of sediments were not observed in the field surveys (Fig. 5c and d). As a result of photographic analysis of the grain size within a 1 m grid on the debris flow deposits, the Neogene sedimentary rock site was finer than the Paleogene accretionary complex site; approximately 70 % were cobble (maximum particle size of 14 cm) and approximately 30 % were gravel, sands, and silts. Fine-grained sediments were produced from strongly weathered outcrops with joint spacing of 1–15 cm on valley walls in many basins.
The presence or absence of debris flow fans classified by topographic map interpretation agreed with the field survey in seven out of eight surveyed basins. In the basin that did not agree with the classification, the field survey revealed that the fan was small and slightly gentler than 5°. Thus, this incorrectly classified fan was likely a debris flood fan.
4.2.2 Decision tree analysis
A total of 90 drainage basins were extracted using GIS analysis. As a result of the topographic map interpretation, the numbers of basins with and without debris flow fans were 24 and 66, respectively. The decision tree was composed of four variables and five leaves (Fig. 8). The results of the decision tree analysis agreed with those of the topographic map analysis in 77 of the 90 analyzed basins (86 %), whereas the remaining 13 basins were incorrect.
The relief ratio was the most important morphological factor (top hierarchy factor). The first decision indicates that debris flow fans were formed under the condition of a relief ratio > 0.36 (19.8°) (Sed Group 1 in Fig. 8). The second most important morphological factor was MFSG. The second decision indicates that debris flow fans were not formed under the conditions of relief ratio ≤ 0.36 and MFSG > 25° (Sed Group 2 in Fig. 8). Field surveys in the Sed Group 2 basins revealed that the channel deposits in the valley were covered with vegetation, indicating low sediment transfer activity in the channel. In addition, according to the GIS analysis of these basins, the areas with slope gradients > 25° were mainly located on the slopes in the lower part of the basin formed by significant downward dissection. The third most important morphological factor was the MFSG. The third decision indicates that debris flow fans were not formed under the conditions of relief ratio ≤ 0.36 and MSFG ≤ 15° (Sed Group 3 in Fig. 8). The fourth most important morphological factor (the lowest hierarchy factor) was basin length. The fourth decision indicates that debris flow fans were formed under the following conditions: relief ratio ≤ 0.36, 15° < MFSG ≤ 25°, and basin length ≤ 550 m (Sed Group 4 in Fig. 8). The area with a slope gradient of 15–25° was located on the slope above the convex slope break. Of the 25 basins in Sed Group 4, 9 were incorrect basins, and debris flow fans were not identified in the topographic maps. A fan-shaped topography with a slope gradient of <5° was identified at the mouth of these basins. The fifth decision indicates that debris flow fans were not formed under the following conditions: relief ratio ≤ 0.36, 15° < MFSG ≤ 25°, and basin length > 550 m (Sed Group 5 in Fig. 8).
5.1 Interpretation of drainage basins using decision tree analyses
5.1.1 Paleogene accretionary complex site
Debris flow fans were not formed in the Acc Group 1 basins, which had low relief ratios and gentle channels just above the valley mouth (Fig. 7). Debris flows are likely to terminate in the valley because debris flows terminate easily in gentle channel sections (Benda and Cundy, 1990; Takase et al., 2002; De Haas et al., 2018). In Acc Group 2, slopes gentler than 35° were distributed in the upper part of the basin where downward dissection was not evident in the topography. A thick layer of weathered soil accumulates in areas where downward dissection has not yet progressed (Brosens et al., 2020). Therefore, thick regolith, a potential debris flow material, still remains on such gentle slopes, possibly facilitating the occurrence of debris flows. GIS analysis revealed that the channel gradient above the valley mouth in the incorrect basins in Acc Group 2 was less than 5°, suggesting that debris flow fans were not formed in these basins because of the termination of debris flows in the gentle channel sections (Takase et al., 2002; De Haas et al., 2018). The Acc Group 3 basins had steep terrains (MFSG > 35°) and short basin lengths (≤570 m). In the Acc Group 3 basins, unlike the Acc Group 2 basins, significant downward dissection had already reached upstream of the basin. Therefore, the remaining regolith, which can become debris flow material in the future, is limited. Jakob (2021) defined such basins as supply limited basins. However, because of the short length of the channel, once a landslide-initiated debris flow occurs, it reaches the mouth of the basin. As a result, debris flow fans exist in the Acc Group 3 basins. In the Acc Group 4 basins, landslide sediment did not reach the valley mouth as a debris flow because of the long basin length, resulting in absence of debris flow fans (Calvache et al., 1997; Sorriso-Valvo et al., 1998; Gómez-Villar and García-Ruiz, 2000; Crosta and Frattini, 2004). In addition, debris floods are more important than debris flows in basins with long basin lengths (Wilford et al., 2004; De Scally et al., 2010; Welsh and Davies, 2011).
5.1.2 Neogene sedimentary rock site
Because the Sed Group 1 basins had a high relief ratio and a short basin length, the produced sediments easily reached the valley mouth as debris flows, forming debris flow fans. Previous studies have reported that the threshold of relief ratio needed for debris flow occurrence ranges from 0.25 to 0.37 (Wilford et al., 2004; De Scally et al., 2010; Ilinca, 2021). The results obtained in this study (0.36) were within the range of this threshold.
The absence of debris flow fans in the Sed Group 2 and 3 basins was likely affected by the supply-limited characteristics of the basins (Bovis and Jakob, 1999; Jakob, 2021). In the Sed Group 2 basins, the topography indicates past significant dissection and poor remaining regoliths. In the Sed Group 3 basins, terrains are likely too gentle (MFSG ≤ 15°, relief ratio ≤ 0.36) to supply debris flow material to the channel. In the basin of Sed Group 4, the MFSG ranged from 15 to 25°, and slopes with such steepness were primarily distributed above the convex slope break. Therefore, hillslopes still store unstable sediments and are potential sources of debris flow material. In addition, because the basin length is short, the produced sediment easily reaches the outside of the basin as debris flows, forming debris flow fans. Gentle fluvial fans with slopes of less than 5° at the basin mouths were identified in the incorrect basins of Sed Group 4, indicating that fluvial processes evacuated the sediments. In Sed Group 5, debris flow fans were not formed because of the longer basin length than in Sed Group 4. As with the Paleogene accretionary complex site, landslide sediments were deposited in the valley and did not reach the valley mouth as debris flows. In addition, debris floods are more important sediment transfer processes than debris flows in basins with longer basin lengths (Wilford et al., 2004; De Scally et al., 2010; Welsh and Davies, 2011).
5.2 Commonalities in morphological conditions contributing to the formation of debris flow fans
Decision trees of the Neogene sedimentary rock (soft rock) site and the Paleogene accretionary complex (hard rock) site include the relief ratio, MFSG, and basin length (Figs. 7 and 8, Table 1). In addition, the hierarchy was in the order of relief ratio, MFSG, and basin length at both sites. Saito (1998) also pointed out that the relief ratio is the most important morphological factor affecting the presence or absence of fans. Wang et al. (2019) reported that the relief ratio is the most important morphological property for debris flow occurrence. Our decision tree analysis applied to 158 basins confirmed that the relief ratio is the most important factor in the formation of debris flow fans, regardless of the rock hardness. The relief ratio is a morphological factor that affects the sediment supply and evacuation from the valley (De Scally et al., 2010; Wang et al., 2019). Hence, the relief ratio is likely to be the most important factor controlling the formation of debris flow fans, regardless of geology. The MFSG and basin length are important factors affecting debris flow occurrence (Figs. 7 and 8, Table 1) (Rowbotham et al., 2005; De Scally et al., 2010). The MFSG likely affects regolith thickness (Brosens et al., 2020) and sediment supply activity, whereas the basin length controls the ratio of sediments reaching the valley mouth as debris flow. The threshold of basin length was not significantly different between the two geologies, and fewer debris flow fans were formed in basins with longer basin lengths. This trend is likely common regardless of geological settings because similar trends have also been reported in other regions (e.g., Wilford et al., 2004; De Scally et al., 2010; Welsh and Davies, 2011).
Table 1Summary of the analysis results at each study site.
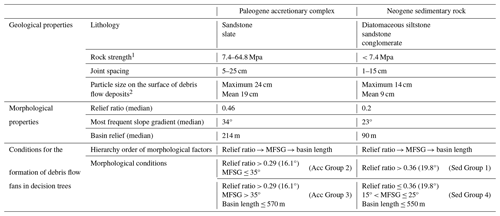
1 The Schmidt rock hammer used in this study has a measurement limit of 7.4 Mpa or less. Measurements were carried out on all lithologies listed in the table, and the measured values were organized by Neogene sedimentary rock and Paleogene accretionary complex areas. 2 There were 10 clasts selected by size within a 1 m grid.
Basin area and basin relief, which characterize the entire basin, were not selected as factors determining the decision tree in both of geologic units. These morphological variables are affected by areas that do not directly contribute to the occurrence of debris flows. Consequently, regardless of the geology, the morphological factors that control sediment supply activities and the ratio of sediments reaching the valley mouth as debris flow are more important for the formation of debris flow fans than morphological features of the entire basin.
5.3 Differences in morphological conditions contributing to the formation of debris flow fans
Paleogene accretionary complex sites have higher bedrock strength, coarser grain distribution of bed sediments, and higher relief ratio than Neogene sedimentary rock sites (Fig. 2c). The relief ratio in harder rock basins is generally high because of low erodibility of valley side slopes (Schmidt and Montgomery, 1995; Kühni and Pfiffner, 2001; Moore et al., 2009). Basins with coarser bed materials have higher relief ratios and steeper channel gradients (Hack, 1957; Fratkin et al., 2020). In other words, the differences in geology affect rock strength and grain size of bed sediments, resulting in different relief ratios (Hack, 1957; Schmidt and Montgomery, 1995; Kühni and Pfiffner, 2001; Moore et al., 2009; Fratkin et al., 2020).
The threshold of morphological factors that determine the presence or absence of debris flow fans differed between the two geological units (Figs. 7 and 8, Table 1). Debris flow fans were not formed at the Paleogene accretionary complex site when the relief ratio was 0.29 (16.1°) or less (Acc Group 1). The Paleogene accretionary complex site contained coarse-grained sediments. If the relief ratio is small, coarse sediments are not easily transported downstream, which makes it difficult to form debris flow fans. On the other hand, debris flow fans were formed in short basins at Neogene sedimentary rock sites, even if the relief ratio was less than 0.36 (Sed Group 4). In our field observations, the sediments (gravelly clasts) on the debris flow fan exhibited a maximum particle size of 14 cm at the Neogene sedimentary rock site. These clasts were sourced from weathered soft rocks with dense fractures spaced at intervals of 1–15 cm. In contrast, at the Paleogene accretionary complex site, which consisted of relatively hard rocks with sparsely developed joints spaced at 5–25 cm, the maximum particle size of the debris flow fan was 24 cm. This suggests that sediments from the Neogene region can be easily transported downstream even with low relief ratios. Previous studies reported that lower limits of relief ratio for forming debris flow fans vary depending on geology (Wilford et al., 2004; De Scally et al., 2010; Ilinca, 2021). Our study implies that the difference in the threshold of relief ratio reflects the variations in rock strength and the resultant dominant gravel size among the studied sites. Therefore, it is considered that the difference in the relief ratio affects the threshold.
Next to the relief ratio, the most important morphological factor is the MFSG at both geological sites. However, the formation threshold of the debris flow fan at the Paleogene accretionary complex sites (35°) is higher than that at the Neogene sedimentary rock site (15 to 25°; Figs. 7 and 8, Table 1). Slope gradients of terrains that have poorly dissected basins are less than 35° and 15 to 25° in the Paleogene accretionary complex and Neogene sedimentary rock, respectively (Figs. 1 and 2a). Such differences in the dominant gradients among geologies have also been reported by Korup (2008). Therefore, it is considered that the different thresholds of the MFSG between the two geologies are affected by the slope gradients of areas where significant dissection has not yet progressed.
In this study, we investigated the morphological conditions of a drainage basin that contribute to the formation of debris flow fans using decision tree analysis. The analysis was conducted at two sites with clear differences in rock strength due to geological processes: Neogene sedimentary rock (soft rock) and Paleogene accretionary complex (hard rock) sites. Debris flow fans and the boundaries of drainage basins at the study sites were extracted using topographic maps and DEMs. Field surveys were conducted to validate the extraction of debris flow fans from topographic maps and to check the current activity of the debris flow in selected basins. After calculating the morphological parameters in each basin, a decision tree analysis was performed using the presence or absence of debris flow fans as the objective variable and the morphological variables of the drainage basin as the explanatory variables. As a result, important morphological factors affecting the presence or absence of debris flow fans were common regardless of geology. At both sites, the first, second, and third most important morphological factors were the relief ratio, most frequent slope gradient, and basin length, respectively. These morphological factors are considered important in the evaluation of debris flow risks.
In contrast, the threshold of the morphological parameters needed for forming debris flow fans differed depending on the geological features. This might be due to the difference in physical properties of the two geological features. In other words, at the Paleogene accretionary complex site, when the relief ratio was less than 0.29, coarse-grained sediments were less likely to flow downstream, resulting in the absence of debris flow fans. On the other hand, short basins at Neogene sedimentary rock sites were determined to have debris flow fans even if the relief ratio was less than 0.36 because the sediments were fine-grained and tended to be transported downstream.
Field surveys and analyses of topographic maps and DEMs revealed that morphological conditions determined by decision tree analysis can control the types and activities of sediment transfer processes at the study site. Therefore, this study demonstrated that the decision tree analysis is an effective tool for obtaining the hierarchy and threshold of morphological factors that determine the presence or absence of debris flows fans for each geology. The commonalities and differences in the morphological conditions for forming debris flow fans in different geologies could be identified using the decision tree analysis. Morphological conditions for forming debris flow fans are essential in evaluating debris flow risks because the absence or presence of debris flow fans is highly affected by debris flow at the valley mouth. Therefore, it is possible that the morphological conditions obtained in this study can be used to assess debris flow risks in basins in which debris flow fans have been lost because of artificial land formation and fluvial processes. Furthermore, this study will be useful for designing an early warning system in basins with high risk of debris flow.
The corresponding author has all of the data analyzed in this study. Most of the data can be shared upon agreement of the authors. For further information, please contact the corresponding author.
All of the authors designed the study. KK conducted the decision tree analysis and compiled the paper. SI contributed to the morphological analysis. FI contributed to the interpretation of the results. GK contributed to the geological interpretation. All of the authors conducted field survey and were involved in reviewing and editing the paper.
The contact author has declared that none of the authors has any competing interests.
Publisher's note: Copernicus Publications remains neutral with regard to jurisdictional claims made in the text, published maps, institutional affiliations, or any other geographical representation in this paper. While Copernicus Publications makes every effort to include appropriate place names, the final responsibility lies with the authors.
The authors acknowledge Roy C. Sidle and Takashi Kimura for their constructive comments that improved this paper.
This research has been supported by the Japan Society for the Promotion of Science (JSPS) grant no. 21K05674.
This paper was edited by Mario Parise and reviewed by Roy Sidle and Takashi Kimura.
Badoux, A., Andres, N., Techel, F., and Hegg, C.: Natural hazard fatalities in Switzerland from 1946 to 2015, Nat. Hazards Earth Syst. Sci., 16, 2747–2768, https://doi.org/10.5194/nhess-16-2747-2016, 2016.
Benda, L. E. and Cundy, T. W.: Predicting deposition of debris flows in mountain channels, Can. Geotech. J., 27, 409–417, https://doi.org/10.1139/t90-057, 1990.
Benvenuti, M., Bonini, M., and Morini, A.: Tectonic control on the Late Quaternary hydrography of the Upper Tiber Basin (Northern Apennines, Italy), Geomorphology, 269, 85–103, https://doi.org/10.1016/j.geomorph.2016.06.017, 2016.
Bou Kheir, R., Chorowicz, J., Abdallah, C., and Dhont, D.: Soil and bedrock distribution estimated from gully form and frequency: a GIS-based decision-tree model for Lebanon, Geomorphology, 93, 482–492, https://doi.org/10.1016/j.geomorph.2007.03.010, 2008.
Bovis, M. J. and Jakob, M.: The role of debris supply conditions in predicting debris flow activity, Earth Surf. Proc. Land., 24, 1039–1054, https://doi.org/10.1002/(SICI)1096-9837(199910)24:11<1039::AID-ESP29>3.0.CO;2-U, 1999.
Brosens, L., Campforts, B., Robinet, J., Vanacker, V., Opfergelt, S., Ameijeiras-Mariño, Y., Minella, J. P. G., and Govers, G.: Slope gradient controls soil thickness and chemical weathering in subtropical Brazil: Understanding rates and timescales of regional soilscape evolution through a combination of field data and modelling, J. Geophys. Res.-Earth, 125, e2019JF005321, https://doi.org/10.1029/2019JF005321, 2020.
Calvache, M .L., Viseras, C., and Fernández, J.: Controls on fan development – evidence from fan morphometry and sedimentology; Sierra Nevada, SE Spain, Geomorphology, 21, 69–84, https://doi.org/10.1016/S0169-555X(97)00035-4, 1997.
Crosta, G. B. and Frattini, P.: Controls onmodern alluvial fan processes in the central Alps, northern Italy, Earth Surf. Proc. Land., 29, 267–293, https://doi.org/10.1002/esp.1009, 2005.
De Haas, T., Kleinhans, M. G., Carbonneau, P. E., Rubensdotter, L., and Hauber, E.: Surface morphology of fans in the high-Arctic periglacial environment of Svalbard: Controls and processes, Earth-Sci. Rev., 146, 163–182, https://doi.org/10.1016/j.earscirev.2015.04.004, 2015.
De Haas, T., Densmore, A. L., Stoffel, M., Suwa, H., Imaizumi, F., Ballesteros-Cánovas, J. A., and Wasklewicz, T.: Avulsions and the spatio-temporal evolution of debris-flow fans, Earth-Sci. Rev., 117, 53–75, https://doi.org/10.1016/j.earscirev.2017.11.007, 2018.
De Scally, F. A. and Owens, I. F.: Morphometric controls and geomorphic responses on fans in the Southern Alps, New Zealand, Earth Surf. Proc. Land., 29, 311–322, https://doi.org/10.1002/esp.1022, 2004.
De Scally, F. A., Owens, I. F., and Louis, J.: Controls on fan depositional processes in the schist ranges of the Southern Alps, New Zealand, and implications for debris-flow hazard assessment, Geomorphology, 122, 99–116, https://doi.org/10.1016/j.geomorph.2010.06.002, 2010.
Dowling, C. A. and Santi, P. M.: Debris flows and their toll on human life: a global analysis of debris-flow fatalities from 1950 to 2011, Nat. Hazards, 71, 203–227, https://doi.org/10.1007/s11069-013-0907-4, 2014.
Fratkin, M. M., Segura, C., and Bywater-Reyes, S.: The influence of lithology on channel geometry and bed sediment organization in mountainous hillslope-coupled streams, Earth Surf. Proc. Land., 45, 2365–2379 https://doi.org/10.1002/esp.4885, 2020.
Geological Survey of Japan: AIST, Seamless digital geological map of Japan V2 , https://gbank.gsj.jp/seamless/ (last access: 17 June 2022), 2022.
Giles, P. T.: Investigating the use of alluvial fan volume to represent fan size in morphometric Studies, Geomorphology, 121, 317–328, https://doi.org/10.1016/j.geomorph.2010.05.001, 2010.
Gómez-Villar, A. and García-Ruiz, J. M.: Surface sediment characteristics and present dynamics in alluvial fans of the central Spanish Pyrenees, Geomorphology, 34, 127–144, https://doi.org/10.1016/S0169-555X(99)00116-6, 2000.
Guzzetti, F., Marchetti, M., and Reichenbach, P.: Large alluvial fans in the north-central Po Plain (northern Italy), Geomorphology, 18, 119–136, https://doi.org/10.1016/S0169-555X(96)00015-3, 1997.
Hack, J. T.: Studies of longitudinal stream profiles in Virginia and Maryland, US Geological Professional Paper 294-B, US Geological Survey, 45–97, https://doi.org/10.3133/pp294B, 1957.
Ilinca, V.: Using morphometrics to distinguish between debris flow, debris flood and flood (Southern Carpathians, Romania), Catena, 197, 104982, https://doi.org/10.1016/j.catena.2020.104982, 2021.
Imai, I. and Sumi, Y.: Geological Map of Japan, Tomikawa with Explanatory Text, Hokkaido Dev. Agency, 52 pp., https://www.gsj.jp/data/50KGM/PDF/GSJ_MAP_G050_04055_1958_D.pdf (last access: 17 June 2022), 1957.
Jakob, M.: Landslides in a changing climate, in: Landslide Hazards, Risks, and Disasters, 2nd Edn., Hazards and Disasters Series, edited by: Davies, T., Rosser, N., and Shroder, J. F., Elsevier B.V., 505–579, https://doi.org/10.1016/C2018-0-02502-5, 2021.
Korup, O.: Rock type leaves topographic signature in landslide-dominated mountain ranges, Geophys. Res. Lett., 35, L11402, https://doi.org/10.1029/2008GL034157, 2008.
Kühni, A. and Pfiffner, O.: The relief of the Swiss Alps and adjacent areas and its relation to lithology and structure: topographic analysis from a 250-m DEM, Geomorphology, 41, 285–307, https://doi.org/10.1016/S0169-555X(01)00060-5, 2001.
Melton, M. A.: The geomorphic and paleoclimatic significance of alluvial deposits in southern Arizona, J. Geol., 73, 1–38, https://doi.org/10.1086/627044, 1965.
Meyer, G. A. and Wells, S. G.: Fire-related sedimentation events on alluvial fans, Yellowstone National Park, USA, J. Sediment Res., 67, 776–791, https://doi.org/10.1306/D426863A-2B26-11D7-8648000102C1865D, 1997.
Moore, J. C. and Saffer, D.: Updip limit of the seismogenic zone beneath the accretionary prism of southwest Japan: an effect of diagenetic to low-grade metamorphic processes and increasing effective stress, Geology, 29, 183–186, 2001.
Moore, J. R., Sanders, J. W., Dietrich, W. E., and Glaser, S. D.: Influence of rock mass strength on the erosion rate of alpine cliffs, Earth Surf. Proc. Land., 34, 1339–1352, https://doi.org/10.1002/esp.1821, 2009.
Nanayama, F.: Stratigraphy and facies of the Paleocene Nakanogawa Group in the southern part of central Hokkaido, Japan, J. Geol. Soc. Jpn., 98, 1041–1059, https://doi.org/10.5575/geosoc.98.1041, 1992.
Pal, M. and Mather, P.: An assessment of the effectiveness of decision tree methods for land cover classification, Remote. Sens. Environ., 86, 554–565, https://doi.org/10.1016/S0034-4257(03)00132-9, 2003.
Rowbotham, D., de Scally, F. A., and Louis, J.: The identification of debris torrent basins using morphometric measures derived within a GIS, Geograf. Ann. A, 87, 527–537, https://doi.org/10.1111/j.0435-3676.2005.00276.x, 2005.
Saito, H., Nakayama, D., and Matsuyama, H.: Comparison of landslide susceptibility based on a decision-tree model and actual landslide occurrence: The Akaishi Mountains, Japan, Geomorphology, 109, 108–121, https://doi.org/10.1016/j.geomorph.2009.02.026, 2009.
Saito, K. (Ed.): Alluvial Fans of Japan, Kokon Shoin, Tokyo, 280 pp., ISBN 4772250182, 1998.
Schmidt, K. M. and Montgomery, D. R.: Limits to relief, Science, 270, 617–620, https://doi.org/10.1126/science.270.5236.617, 1995.
Schneevoigt, N., van der Linden, S., Thamm, H., and Schrott, L.: Detecting Alpine landforms from remotely sensed imagery. A pilot study in the Bavarian Alps, Geomorphology, 93, 104–119, https://doi.org/10.1016/j.geomorph.2006.12.034, 2008.
Sorriso-Valvo, M., Antronico, L., and Le Pera, E.: Controls on modern fan morphology in Calabria, southern Italy, Geomorphology, 24, 169–187, https://doi.org/10.1016/S0169-555X(97)00079-2, 1998.
Suzuki, M., Hashimoto, S., Asai, H., and Matsushita, K.: Geological Map of Japan, Rakkodake with Explanatory Text, Hokkaido Dev. Agency, 63 pp., https://www.hro.or.jp/upload/29695/kushiro67.pdf (last access: 17 June 2022), 1959.
Takase, Y., Tomura, K., Fujimori, S., and Suzuki, T.: Relationships between Roundness of Gravel and Gradient of Talus, Alluvial Cones and Alluvial Fans, Trans. Japan. Geomorphol. Union, 23, 101–110, https://drive.google.com/file/d/1pKGMixYbt2dZM-1T0CVKptZ61ZyP05Bc/view (last access: 17 June 2022), 2002.
The University of Waikato: WEKA, http://www.cs.waikato.ac.nz/~ml/weka/index.html (last access: 17 June 2022), 2008.
Wang, N., Cheng, W., Zhao, M., Liu, Q., and Wang, J.: Identification of the Debris Flow Process Types within Catchments of Beijing Mountainous Area, Water, 11, 638, https://doi.org/10.3390/w11040638, 2019.
Welsh, A. and Davies, T.: Identification of alluvial fans susceptible to debris-flow hazards, Landslides, 8, 183–194, https://doi.org/10.1007/s10346-010-0238-4, 2011.
Wilford, D. J., Sakals, M. E., Innes, J. L., Sidle, R. C., and Bergerud, W. A.: Recognition of debris flow, debris flood and flood hazard through watershed morphometrics, Landslides, 1, 61–66, https://doi.org/10.1007/s10346-003-0002-0, 2004.
Witten, I. H. and Frank, E. (Eds.): Data Mining: Practical Machine Learning Tools and Techniques, in: 2nd Edn., Elsevier, 560 pp., ISBN 0120884070, 2005.
Yoshiyama, A.: Late Quaternary Crustal Movement around the Hidaka Mountains, Hokkaido, Japan, Quatern. Res., 28, 369–387, https://doi.org/10.4116/jaqua.28.369, 1990.
Zhang, Y., Ge, T., Tian, W., and Liou, Y.-A.: Debris Flow Susceptibility Mapping Using Machine-Learning Techniques in Shigatse Area, China, Remote Sens., 11, 2801, https://doi.org/10.3390/rs11232801, 2019.
Zhou, W., Tang, C., Asch, T. W. J., and Chang, M.: A rapid method to identify the potential of debris flow development induced by rainfall in the catchments of the Wenchuan earthquake area, Landslides, 13, 1243–1259, https://doi.org/10.1007/s10346-015-0631-0, 2016.