the Creative Commons Attribution 4.0 License.
the Creative Commons Attribution 4.0 License.
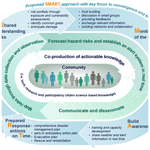
Brief communication: Inclusiveness in designing an early warning system for flood resilience
Tahmina Yasmin
Kieran Khamis
Anthony Ross
Subir Sen
Anita Sharma
Debashish Sen
Sumit Sen
Wouter Buytaert
Floods remain a wicked problem and are becoming more destructive with widespread ecological, social, and economic impacts. The problem is acute in mountainous river catchments where plausible assumptions of risk behaviour to flood exposure and vulnerability are crucial. Inclusive approaches are required to design suitable flood early warning systems (EWSs) with a focus on local social and governance context rather than technology, as is the case with existing practice. We assess potential approaches for facilitating inclusiveness in designing EWSs by integrating diverse contexts and identifying preconditions and missing links. We advocate the use of a SMART approach as a checklist for good practice to facilitate bottom-up initiatives that benefit the community at risk by engaging them at every stage of the decision-making process.
- Article
(3055 KB) - Full-text XML
- BibTeX
- EndNote
The theme for World Meteorological Day 2022 (23 March) was “Early Warning and Early Action – Hydrometeorological and Climate Information for Disaster Risk Reduction”, which emphasises the vital importance of information generation and sharing to minimise the risks from hydrometeorological extremes. Further, the United Nations secretary general announced a major initiative, to be delivered via COP 27 (UN Climate Conference): “everyone on Earth should be protected by early warning systems against extreme weather and climate change within the next five years”. These policy initiatives indicate the growing need for new information and knowledge relating to risks arising directly from hazard but also from the complex interactions with exposure and vulnerability (IPCC defined risk = hazard × exposure × vulnerability capacity to cope; see details in Cardona et al., 2012). Although our understanding of hydrological extremes, such as floods, has evolved in recent decades as we view them through the lens of hydro-complexity (Kosow et al., 2022). However, floods remain a “wicked” problem and are becoming more destructive with ecological, social, and economic impacts (i.e. source of water pollution, damages to wastewater and irrigation system, excessive erosion damaging riverbank settlements; see details in Kosow et al., 2022; Hannah et al., 2020). In mountainous regions floods are becoming more unpredictable and destructive in response to increasing climatic extremes. This is exacerbated by anthropogenic pressures which have severely modified formerly pristine, high-altitude river catchments. Furthermore, increased encroachment of riverbanks, dumping of solid and sewer waste, and rapid urbanisation has increased the proportion of low-income communities living in flood-prone areas (Mao et al., 2018; Paul et al., 2018). The lack of adequate hydrometeorological monitoring networks or early warning system in these regions causes undue damage to lives and property (Mountain-Evo, 2017; Pandeya et al., 2021). Yet prediction of risks associated with floods is difficult to achieve in such data-scarce mountainous regions.
Indeed, the most recent report of the Intergovernmental Panel on Climate Change (IPCC, 2022) highlighted the urgent need for investment in adaptation and resilience, particularly in developing regions which have been historically underfunded but are already impacted by extreme weather events. A key requirement is to improve early warning alerts of anticipated storms, heatwaves, floods and droughts. To generate such warning information for floods, systematic development of monitoring networks that utilise appropriate technologies is required. These systems should also consider social, cultural and political dimensions to identify context-specific understanding on inequality and its impact on assessing vulnerabilities and exposure, so that the warning system can ensure inclusiveness in responses following appropriate decision-making chains (Mao et al., 2018; Acosta-Coll et al., 2018). Such an integrated and interconnected monitoring system requires science, policy and local community-led approaches that can bring diverse stakeholders (i.e. gender, sex, age, socio-economic status and physical abilities) together and generate knowledge to guide their decision to propose solutions that fit the local context (Buytaert et al., 2018; Kosow et al., 2022; Roque et al., 2022; Zulkafli et al., 2017). Despite this call for an inclusive approach for generating an early warning alert system, the existing flood monitoring practices and designs are strongly technology-driven (i.e. information and communications technology – ICT) and focus less on converging with the local socio-cultural and governance context (Mao et al., 2018; Westerhoff et al., 2021). There are still questions on how, where and at what level science, policy and society may converge and facilitate bottom-up initiatives for decision-making and develop innovative solutions to address challenges posed by floods.
In this commentary, we assess potential approaches for facilitating inclusiveness in the design of a flood early warning system by integrating social, cultural and political aspects and identify preconditions and missing links.
In water and disaster research several approaches are emerging to provide concepts, tools and framings that can be used to support inclusiveness and disciplinary convergence for actionable knowledge production. The concept of knowledge co-production has emerged from science–society interaction under the umbrella of adaptive governance thinking where polycentric models and power relation received attention (see details in Buytaert et al., 2018; Paul et al., 2018; Zulkafli et al., 2017). Scholarly research has identified several potential approaches to achieve knowledge co-production under the broader umbrella of the participatory action research (PAR) including participatory modelling (Sterling et al., 2019), community-based participatory approaches (Wallerstein et al., 2017), participatory scenario analysis (Birthisel et al., 2020; Lakhina et al., 2021; Westerhoff et al., 2021), among others. More recently, citizen science has emerged with an emphasis on “knowledge cocreation and co-generation” (i.e. the interactive processes across science, policy and implementation to collaborate and to generate knowledge for supporting environmental decision-making; see further details in Buytaert et al., 2018) and new technologies, especially ICT, but limited focus on action and development. In addition, citizen science focuses more on participation by volunteers, developing trust and nurturing existing working relationships among involved actors towards knowledge co-production (Buytaert et al., 2018; Zulkafli et al., 2017).
In the contemporary disaster research literature, knowledge co-production is advocated along with participatory actions and transdisciplinary research, which laid the foundation for the participatory convergence concept to translate research into practice (Lakhina et al., 2021; Peek et al., 2020; Roque et al., 2022). Peek et al. (2020) define the participatory convergence research as “an approach to knowledge production and action that involves diverse teams working together in novel ways – transcending disciplinary and organisational boundaries – to address vexing social, economic, environmental, and technical challenges in an effort to reduce disaster losses and promote collective well-being” (p. 2). While this research approach has been identified as one of the best 10 big ideas in funding allocation and research direction by the National Science Foundation of USA (2016), there has been little exploration on the framing (i.e. methods and ethics) to apply this in practice (Westerhoff et al., 2021). Indeed, scholars are focusing on more empirical exploration of convergence research to generate ethics and methods that may deliver successful outcomes, for example, research attempting to address coping with water extremes such as floods and droughts (Lakhina et al., 2021; Roque et al., 2022; Westerhoff et al., 2021). Recently, scholars have proposed ethics that have proven useful. For example, Lakhina et al. (2021) proposed “convergence with CARE: collaboration, accountability, responsiveness and empowerment” which require community engagement and further highlight their perspective, questions and experiences while disregarding traditional hierarchical approaches. However, much hydrological research is focused on improving scientific measurements and developing technological solutions, for example, improving model uncertainty or the instruments and networks used to measure different facets of the hydrosphere (Beven et al., 2020) while being useful for advancing the discipline result in solutions that are often difficult to disseminate to local communities (Birthisel et al., 2020; Roque et al., 2022; Westerhoff et al., 2021). Earlier reviews indicate many empirical investigations on how social context, such as culture, politics and economics, has shaped water knowledge and how and what interventions influence or shape communities' responses differently (Roque et al., 2022). This emphasises a need for future research to understand the underlying principles and ethics that would facilitate bottom-up driven activities or active participation of engaged stakeholders for knowledge co-production to respond to and reshape convergence research methods.
A synthesis of the literature on flood early warning systems was reviewed to develop a schematic representation of an idealised framework for developing an inclusive early warning system (Fig. 1) (for more details see Acosta-Coll et al., 2018; Buytaert et al., 2018; Mashi et al., 2020; Paul et al., 2018; Zulkafli et al., 2017). The foundation of this schematic representation (Fig. 1) is adapted from the concept of knowledge co-generation processes (Buytaert et al., 2018) and co-design framing for environmental decision-making processes in a polycentric system (Zulkafli et al., 2017) and then applied with the key elements (i.e. risk knowledge; technical monitoring and warning service); communication and dissemination of warnings and community response capability (ISDR, 2020) identified by the World Meteorological Organization, International Strategy for Disaster Reduction (ISDR). All these concepts, in general, advocated a participatory and citizen science approach to become inclusive and generate actionable knowledge (Buytaert et al., 2018; ISDR, 2020; Paul et al., 2018; WMO, 2020). The disaster risk equation provided by the IPCC (risk = hazard × exposure × vulnerability capacity to cope) suggests that reduction in risk is dependent not only on efficient forecasting of hazard, but also on the understanding of associated exposure, vulnerability and capacity to cope by the exposed community. Therefore, in Fig 1, we represent three interdependent steps: (1) mapping the risks through data collection and observation; (2) forecasting hazard risks and establishing an alert system in real time; and (3) communication and dissemination.
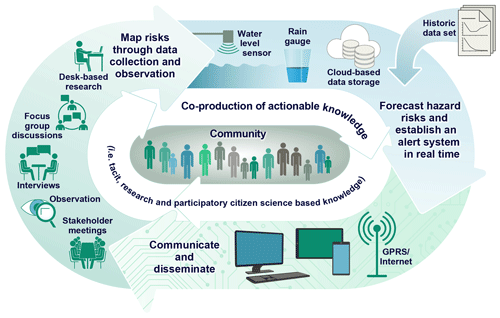
Figure 1An idealised scenario for developing a monitoring and alert system to provide an early warning of potentially life/livelihood threatening natural hazards.
3.1 Mapping the risks through data collection and observation
In this step, it is crucial to collect as much information as possible, to generate knowledge on the locality and the community at risk to design a purposeful early warning system. The knowledge generated can also inform on exposure, vulnerability and ability to cope when a disaster strikes and enable decision-makers to adjust or adapt necessary precautionary measures to respond efficiently in a timely manner (Buytaert et al., 2018; Pandeya et al., 2021). The required knowledge includes scientific measurements of the hydrological hazard, various contexts of risks information (i.e. vulnerability and exposure mapping) across the social, cultural and political domains that contribute to the risk portfolio to be more intense and having long-term consequences (Mao et al., 2018). In general, we found most studies generate information on risk through a baseline survey of exposure and vulnerability analysis via observations, interviews, focus group discussions and stakeholders' meetings. The data generated through the baseline survey focus on a variety of aspects including historical analysis; geographical aspects; environmental, social, and economic aspects; and governance structures. All these are relevant; however, what is missing here is the lens through which it is possible to explore the complexity of the risk portfolio determined through different angles of exposure and vulnerability perceived by different stakeholders. Reaction to risks in terms of exposure and vulnerability is dependent on the social, cultural and political stances of stakeholders, and thus it is highly variable (Mashi et al., 2020; Hermans et al., 2022). For instance, the communities that are living in flood-vulnerable areas might not have legal rights to do so; therefore, they might decide to tolerate that risk due to fear of eviction. Other stakeholders may be from state organisations which are not bound to provide services to this illegal settlement and, therefore, will not engage. People might not engage either as they already lost their trust in the governance system (i.e. did not receive compensation for their previous flood damage, recurring failed commitments from the political parties to reduce flood vulnerability). Previous research partly discussed these complexities (e.g. Acosta-Coll et al., 2018; Hermans et al., 2022; Mashi et al., 2020); however, solutions to these challenges are limited.
The citizen science approach, in such cases, recommends utilising social capital tools, such as building a relationship with trust across stakeholders, identifying the people with leadership qualities or local champions (i.e. community members or social activist/government/non-government employees who have some form of knowledge of flood risks and are keen to learn about the early warning system) (Acosta-Coll et al., 2018; Mashi et al., 2020). Previous research and project experiences in a similar context demonstrated conducting structured dialogue through stakeholders' meetings, focus group discussions and forming of community groups (see further details in Acosta-Coll et al., 2018; Mashi et al., 2020). However, these interactions can lead to confusion and unrealistic expectation relating to the monitoring system. Therefore, it is crucial to make plausible assumptions of risk behaviour relevant to flood exposure and vulnerability that can feed into designing the early warning system including having more focused conversations with the community at risks, specifying the aim and expected outcome of the flood monitoring system.
3.2 Forecasting hazard risks and establish an alert system in real time
This step utilises information from the previous step to identify design specifications to build the early warning system, for example, suitable sensor technology, identification of relevant variables (i.e. rainfall, water level), and suitable location(s) to install the components and transmit/receive data. In addition, decision-making on data collection attributes, such as data transmission frequency, among others is critical because there will always be a trade-off between lead time and the potential for an early warning to facilitate appropriate community responses to reduce the likelihood of life. Thus, an understanding of what the optimal lead time in a certain context should be is crucial. To enable any data processing activity, adequate monitoring of relevant variables must be undertaken at the relevant spatial and temporal resolution or scale. This scale will vary depending on the topographic complexity, land cover, geology and hydrodynamic properties of the catchment of interest (Lauden and Sponseller, 2018). If historical data are limited (often the case with mountainous and logistically challenging environments) a period of baseline data collection through the previous step is required to “get to your catchment” before establishing a monitoring network. A range of analytical tools are available, including statistical modelling and simulation, to provide robust thresholds to trigger alert levels based on the collected data. This forecasting step – i.e. predicting the likelihood of flood based on antecedent conditions – is a challenge in data-scarce regions like the Himalaya where there may be significant uncertainty associated with any alert/alarm thresholds due to insufficient training data (Mountain-Evo, 2017; Pandeya et al., 2021). Therefore, many risk assumptions are involved in this step such as over-promising for a sensor-based alert system, and if the forecasts are not accurate, there may be resentment in the community regarding the project. This raises an important question related to understanding the local context to get a good understanding on how risk management happens and what this means for the design. Moreover, how and when should the community (non-scientists) be involved in the development process? Also, what is the purpose of involving the community and other organisations and how will their involvement shape the design process? All these questions are important for the emerging disaster risk management paradigm, where leading organisations (e.g. World Meteorological Organisation (WMO)) and other humanitarian agencies (i.e. International Federation of Red Cross and Red Crescent Societies) suggest moving towards impact-based forecasting and anticipatory humanitarian actions so that context-specific risks could be identified and necessary relevant action plan could develop on time (please see further details in Red Cross Red Crescent and the UK Met Office, 2022).
Previous research has highlighted the importance of involving relevant state organisations, such as disaster management departments or meteorological organisations, at this stage (Acosta-Coll et al., 2018; Pandeya et al., 2021). However, this can potentially lead to a divergence in terms of priorities; scientists and engineers are generally focused on the success of the adopted technique and necessary data generation, while the state-led organisations might focus on bureaucracy, policy, existing government beliefs and long-term operational plans (e.g. maintenance and legacy costs). Therefore, engaging with the state departments at this stage can become very difficult (Mashi et al., 2020); nonetheless from a design perspective, understanding both contexts is very crucial for building a purposeful early warning system. Previous researchers have recommended utilising a bridging or boundary organisation that can act as a mediator and bridge the gap (Acosta-Coll et al., 2018; Mashi et al., 2020). Few projects involved local technological start-up companies or local research and development organisations. However, there is limited exploration on the community engagement at this stage who struggle to visualise such technical details in real-time application. Further, they are also missing on the crucial aspects of what levels of technical details to share and which is the right time/phase to share with the community or the state authority. This inadequate understanding of deciding the right time or phase will risk over-promising the warning alert.
3.3 Communication and dissemination
After installation of the alert system, identification of the best possible modes of dissemination is critical to further interact with the vulnerable communities and communicate the potential risks along with tentative necessary actions to minimise the risks. While this has been the most critical part, it is also one of the most interactive components in the entire scheme. New ICT technologies, such as interactive dashboard visualisations, give more flexibility in developing the visualisation to disseminate the EWS outputs in a way that can be easily understood by the community, which is a major challenge (Mashi et al., 2020; Pandeya et al., 2021). Several questions arise at this step including a strategy to ensure the alert levels reach all who are at risk, the risk information is easy to understand, and there is a desired reaction to such information. Previous research has highlighted different visualisation techniques to showcase alert levels such as text, colour coding, graphics, audio mobile messages and locational maps (Acosta-Coll et al., 2018; Pandeya et al., 2021). What may be missing in this step is what would be the best possible methods to communicate with the community at risk and understanding how they perceived and responded to such forms of alerts or warnings. Here, there needs to be communication not only with the communities but also with the responsible state authorities about how they are supporting or engaged in the decision-making processes to respond in a timely manner.
We believe that through this commentary we have raised critical questions and identified missing links in the context of disaster resilience and the development of tools to improve preparedness and response. The most important include (i) the absence of diverse contextual risk angle and community reactions; (ii) a lack of community trust in government agencies and technology focused forecasting; (iii) significant data limitations to ensure effective EWS operation and impact-based forecasting; and (iv) a lack of effective communication strategies. All these points need deeper exploration to ensure inclusive EWSs are developed in data-scarce mountainous regions or geographic regions similar in context. We acknowledge that many countries are currently implementing EWSs focusing on active community participation (Red Cross Red Crescent and the UK Met Office, 2022; International Centre for Integrated Mountain Development (ICIMOD), Aranyak and Sustainable Eco Engineering (SEE), 2022, ISDR, 2020; Mountain-Evo, 2017; WMO, 2020); however, solutions to address these missing links are limited, and thus ensuring inclusiveness and impact has remained challenging. We have highlighted the need for multiple lenses to establish and explore the complexity of the risk portfolio and thus understand the architecture of the engaged stakeholders and their behaviour. This is essential to ensure actionable knowledge is generated and bottom-up initiatives are strengthened and the capacity to respond is improved.
Based on the above discussions of key questions, missing links and design needs, we propose the “SMART convergence participatory research” approach to support the EWS development phase and provide a checklist of good practices. The SMART approach highlights crucial activity layers to incorporate into EWS development which can help guide multi-disciplinary teams (e.g. disaster risk manager, hydrologist, engineer and social scientist) (Fig. 2). This will enable the incorporation of diverse disciplinary lenses (i.e. social science and meteorological data) along with risk diversity identified by the community at risk (illegal settlement beside riverbank or slums), which is mentioned earlier as a missing link. This will support exposing vulnerability and risks from different socio-cultural, institutional and scientific contexts. Following a SMART approach will ensure inclusiveness by helping to identify and connect missing components and linkages when designing an EWS.
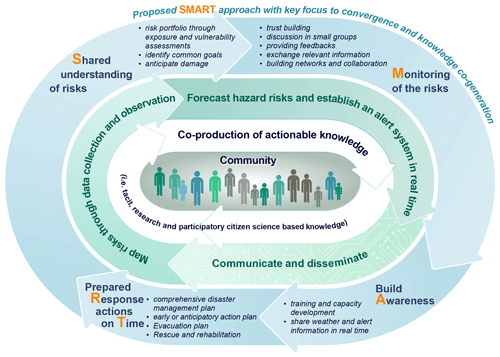
Figure 2A SMART convergence research approach to ensure inclusiveness in designing monitoring and alert system to provide early warning information to minimise disaster risks.
The first step, S, represents “Shared understanding of the risks”, ensuring all stakeholder engagements are diverse and representative (irrespective of their gender, sex, age, socio-economic status and physical abilities), and a wide range of data forms and collection methods are utilised, as stated in EWS step 1 (Fig. 1). This knowledge generated from the community will help the expert group to better understand context-specific risks with a more focused exposure and vulnerability analysis. This further helps to identify common goals and anticipate damage from the natural hazards and thus ensures impact though appropriate forecasting.
Secondly, M – representing “Monitoring of the risks” – aligned closely with establishing alert system and forecasting hazard information as stated in step 2 (Fig. 1). This includes an intersection of generated knowledge that will lead towards practising collaborative activities, such as building trust (which is key to inclusive and impact-based forecasting), exchanging critical risk information to enrich data sets, feedback and forming small groups for maintaining forecasting system.
Thirdly, A, building “Awareness” (i.e. training and capacity development activities to embed understanding of real time weather and alert information) is critical for this approach and is a continuous process throughout the development and utilisation of early warning systems, with a particular focus on EWS step 3 to support effective communication and dissemination and will further also support legacy and sustainability of the warning system in a local context.
Finally, RT – indicating pre-planning “Response actions on Time” (i.e. comprehensive disaster management plan, evacuation plan) based on the alert produced by the EWS – could be used to inform on the effectiveness of the overall EWS to minimise risks from the anticipated hazard. This will inform further on the level of knowledge produced through collaboration and how this can facilitate effective action by the community and responsible agencies.
We advocate the use of this SMART approach to facilitate bottom-up initiatives for developing an inclusive and purposeful early warning system and to benefit the community at risk by engaging them every step of the way along with including other stakeholders at multiple scales of operations (i.e. scientific and policy actors). We advocate that the SMART convergence approach along with the dominant largely top-down initiatives will contribute to developing capacity and redefining adaptation and resilience in the face of more extreme water extremes (floods, droughts) and increased uncertainty under global change.
The data used in this research were open-sourced data gathered from public databases, such as Scopus, Web of Science, and Google Scholar, as well as different United Nations agencies, the World Meteorological Organization, the Intergovernmental Panel on Climate Change (IPCC) and the ICIMOD website. All the relevant reports and project details are listed in the references.
TY: study conception and design, literature review, review data collection and analysis and interpretation of results, draft manuscript preparation. DMH: study conception and design and provided feedback and comments to refine interpretation of results. All other authors reviewed the results and provided their feedback and comments to refine the results and approved the final version of the manuscript.
The contact author has declared that none of the authors has any competing interests.
Publisher’s note: Copernicus Publications remains neutral with regard to jurisdictional claims in published maps and institutional affiliations.
This research has been supported by the Natural Environment Research Council (grant no. NERC COP26 A&R, Project Scoping Call-2021COPA&R31Hannah).
This paper was edited by Kai Schröter and reviewed by three anonymous referees.
Acosta-Coll, M., Ballester-Merelo, F., Martinez-Peiró, M., and la Hoz-Franco, D.: Real-time early warning system design for pluvial flash floods – A review, Sensors, 18, 2255, https://doi.org/10.3390/s18072255, 2018.
Beven, K., Asadullah, A., Bates, P., Blyth, E., Chappell, N., Child, S., Cloke, H., Dadson, S., Everard, N., Fowler, J. H., Freer, J., Hannah, M. D., Heppell, K., Holden, J., Lamb, R., Lewis, H., Morgan, G., Parru, L., and Wagener, T.: Developing observational methods to drive future hydrological science: Can we make a start as a community?, Hydrol. Process., 34, 868–873, https://doi.org/10.1002/hyp.13622, 2020.
Birthisel, S. K., Eastman, B. A., Soucy, A. R., Paul, M., Clements, R. S., White, A., and Dittmer, K. M.: Convergence, continuity, and community: a framework for enabling emerging leaders to build climate solutions in agriculture, forestry, and aquaculture, Climatic Change, 162, 2181–2195, https://doi.org/10.1007/s10584-020-02844-w, 2020.
Buytaert, W., Ochoa-Tocachi, B. F., Hannah, D. M., Clark, J., and Dewulf, A.: Co-generating knowledge on ecosystem services and the role of new technologies, in: Ecosystem Services and Poverty Alleviation, edited by: Schreckenberg, K., Mace, G., and Poudyal, M., Edition 1, Taylor & Francis Group, Routledge, 174–188, https://www.researchgate.net/publication/325442838_Co-generating_ knowledge_on_ecosystem_services_and_the_role_of_new_technologies/link/5b17bc5fa6fdcca67b5d86c6/download (last access: May 2022), 2018.
Cardona, O. D., Van Aalst, M. K., Birkmann, J., Fordham, M., Mc Gregor, G., Perez, R., Pulwarty, R. S., Schipper, E. L. F., and Sinh, B. T.: Determinants of risk: exposure and vulnerability, in: Managing the risks of extreme events and disasters to advance climate change adaptation: special report of the intergovernmental panel on climate change, Cambridge University Press, 65–108, https://doi.org/10.1017/CBO9781139177245.005, 2012.
Hannah, D. M., Lynch, I., Mao, F., Miller, J. D., Young, S. L., and Krause, S.: Water and sanitation for all in a pandemic, Nature Sustainability, 3, 773–775, https://doi.org/10.1038/s41893-020-0593-7, 2020.
Hermans, T. D., Šakić Trogrlić, R., van den Homberg, M. J., Bailon, H., Sarku, R., and Mosurska, A.: Exploring the integration of local and scientific knowledge in early warning systems for disaster risk reduction: a review, Nat. Hazards, 114, 1125–1152, https://doi.org/10.1007/s11069-022-05468-8, 2022.
International Centre for Integrated Mountain Development (ICIMOD), Aranyak and Sustainable Eco Engineering (SEE): Community-Based Flood Early-Warning system-India, https://unfccc.int/climate-action/un-global-climate-action-awards/winning-projects/activity-database/community-based-flood-early-warning-system-india?gclid=Cj0KCQjw--2aBhD5ARIsALiRlwBy8J63opnqOTpqi_9ciM31ONeEat2vk2S1bNk88d-IfxpVYIpld1MaAkpeEALw_wcB, last access: May 2022.
International Strategy for Disaster Reduction (ISDR): Emerging Challenges for Early Warning Systems in context of Climate Change and Urbanization, http://www.preventionweb.net/files/15689_ewsincontextofccandurbanization.pdf (last access: May 2022), 2020.
Kosow, H., Kirschke, S., Borchardt, D., Cullmann, J., Guillaume, J. H. A., Hannah, D. M., Schaub, S., and Tosun, J.: Scenarios of water extremes: Framing ways forward for wicked problems. Hydrol. Process., 36, e14492, https://doi.org/10.1002/hyp.14492, 2022.
Lakhina, S. J., Sutley, E. J., and Wilson, J.: “How do we actually do convergence” for disaster resilience? Cases from Australia and the United States, Int. J. Disast. Risk Sc., 12, 299–311, https://doi.org/10.1007/s13753-021-00340-y, 2021.
Laudon, H. and Sponseller, R. A.: How landscape organization and scale shape catchment hydrology and biogeochemistry: Insights from a long-term catchment study, Wiley Interdisciplinary Reviews: Water, 5, e1265, https://doi.org/10.1002/wat2.1265, 2018.
Mao, F., Clark, J., Buytaert, W., Krause, S., and Hannah, D. M.: Water sensor network applications: Time to move beyond the technical?, Hydrol. Process., 32, 2612–2615, https://doi.org/10.1002/hyp.13179, 2018.
Mashi, S. A., Inkani, A. I., Obaro, O., and Asanarimam, A. S.: Community perception, response and adaptation strategies towards flood risk in a traditional African city, Nat. Hazards, 103, 1727–1759, https://doi.org/10.1007/s11069-020-04052-2, 2020.
Mountain-Evo: Adaptive governance of mountain ecosystem services for poverty alleviation enabled by environmental virtual observatories, https://www.espa.ac.uk/projects/ne-k010239-1 (last access: May 2022), 2017.
Paul, J. D., Buytaert, W., Allen, S., Ballesteros-Cánovas, J. A., Bhusal, J., Cieslik, K., Clark., J., Dugar, S., Hannah, D. M., Stffel, M., Dewulf, A., Dhital, M. R., Liu, W., Nayaval, J. L., Neupane, B., Schiller, A., Smith, J. P., and Supper, R: Citizen science for hydrological risk reduction and resilience building, Wiley Interdisciplinary Reviews: Water, 5, e1262, https://doi.org/10.1002/wat2.1262, 2018.
Pandeya, B., Uprety, M., Paul, J. D., Sharma, R. R., Dugar, S., and Buytaert, W.: Mitigating flood risk using low-cost sensors and citizen science: A proof-of-concept study from western Nepal, J. Flood Risk Manag., 14, e12675, https://doi.org/10.1111/jfr3.12675, 2021.
Peek, L., Tobin, J., Adams, R. M., Wu, H., and Mathews, M. C.: A framework for convergence research in the hazards and disaster field: The natural hazards engineering research infrastructure CONVERGE facility, Front. Built Environ., 6, 110, https://doi.org/10.3389/fbuil.2020.00110, 2020.
Red Cross Red Crescent and the UK Met Office: The Future of Forecasts: Impact-Based Forecasting for Early Action, https://www.anticipation-hub.org/download/file-58, last access: August 2022.
Roque, A., Wutich, A., Quimby, B., Porter, S., Zheng, M., Hossain, M. J., and Brewis, A.: Participatory approaches in water research: A review, Wiley Interdisciplinary Reviews: Water, 9, e1577, https://doi.org/10.1002/wat2.1577, 2022.
Sterling, E. J., Zellner, M., Jenni, K., Leong, K. M., Glynn, P. D., BenDor, T. K., Bommel, T. K., Hubacek, K., Jetter, A. J., Jordan, R., Schmitt Olabisi, L., Paolisso, M., and Gray, S.: Try, try again: Lessons learned from success and failure in participatory modelling, Elementa Science of the Anthropocene, 7, 9, https://doi.org/10.1525/elementa.347, 2019.
Wallerstein, N., Duran, B., Oetzel, J. G., and Minkler, M. (Ed.): Community-based participatory research for health: Advancing social and health equity, John Wiley & Sons, ISBN 978-1-119-25885-8, 2017.
Westerhoff, P., Wutich, A., and Carlson, C.: Value propositions provide a roadmap for convergent research on environmental topics, Environ. Sci. Technol., 55, 13579–13582, https://doi.org/10.1021/acs.est.1c05013, 2021.
World Meteorological Organization (WMO): Guidelines on Multi-hazard Impact-based Forecast and Warning Services, https://library.wmo.int/?lvl=notice_display&id=21994#.YvN5LnbMKUk (last access: August 2022), 2020.
Zulkafli, Z., Perez, K., Vitolo, C., Buytaert, W., Karpouzoglou, T., Dewulf, A., De Bièvre, B., Clark, J., Hannah, D. M., and Shaheed, S.: User-driven design of decision support systems for polycentric environmental resources management, Environ. Modell. Softw., 88, 58–73, https://doi.org/10.1016/j.envsoft.2016.10.012, 2017.