the Creative Commons Attribution 4.0 License.
the Creative Commons Attribution 4.0 License.
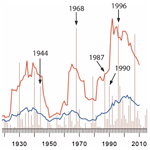
Geo-historical database of flood impacts in Alpine catchments (HIFAVa database, Arve River, France, 1850–2015)
Eva Boisson
Bruno Wilhelm
Emmanuel Garnier
Alain Mélo
Sandrine Anquetin
Isabelle Ruin
In France, flooding is the most common and damaging natural hazard (CCR, 2021). Global warming is expected to exacerbate flood risk and could be more pronounced in the European Alps which are experiencing a high warming rate, likely to lead to heavier rainfall events. Alpine valleys are densely populated, potentially increasing exposure and vulnerability to flood hazard. The study of historical records is highly relevant to understand long-term flood occurrence and related socio-economic impacts in relation to changes in the flood risk components (i.e. hazard, exposure and vulnerability).
To this aim we introduce the newly constituted database of Historical Impacts of Floods in the Arve Valley (HIFAVa) located in the French northern Alps starting in 1850. This quite unique database reports historical impacts related to impact events occurrences in a well-documented Alpine catchment that encompasses both hydrological and socio-economical diversity.
After a complete description of the database (collection, content and structure), we explore the distribution of the recorded impacts with respect to their characteristics and evolution in both time and space. The analysis reveals that small mountain streams and particularly glacial streams caused more impacts (67 %) than the main river. While an increase in heavy rainfall and ice melt are expected to enhance flood hazard in small Alpine catchments, this finding calls for greater attention to flood risk assessment and management in small catchments. The analysis also reveals an increasing occurrence of impacts from 1920 onwards, for which possible factors are discussed. Further work is, however, needed to conclude on the respective contribution of the source effect, the increase in flood hazard, or the exposure of goods and people.
On the mainland French territory flood is the most common and damaging natural hazard in terms of economic cost and number of municipalities concerned (Ministère de la Transition écologique, 2020). In highland regions, these events can be caused, among others, by summer thunderstorms, rain on thawed soils, rain on snow or by glacial lake outburst (Merz and Blöschl, 2003). The topography induces flood events with highly contrasted dynamics; from sudden events with large sediment transport in the upstream small catchments to multi-day events flooding large parts of the valley floor. This diversity of hydrological dynamics adds to the complexity in flood risk management. Furthermore, climate change is expected to increase extreme precipitation (Min et al., 2011) that could in turn increase flooding (Gobiet et al., 2014; Blöschl et al., 2020). This is especially the case for the European Alps where an increase in summer heavy rainfalls (Giorgi et al., 2016; Ménégoz et al., 2020) may threaten densely populated mountainous valleys which are especially vulnerable to climate extremes (IPCC, 2019). With its long history of flooding, the densely populated Arve valley located in the northern French Alps is indeed prone to experience increased flood risk as a result of global warming in the future.
Historical records constitute a source of reliable data to characterize past hydrological events because they contribute to give a comprehensive representation of these events and of their changes over long timescales in spite of the lack of instrumental data (Garnier and Desarthe, 2013; Barriendos et al., 2014; Wetter, 2017; Macdonald and Sangster, 2017; Wilhelm et al., 2019). They also allow us to apprehend changes in flood risk since they document how societies were impacted by past flooding events. Here, we consider impacts according to the IPCC (2012) definition to be all types of outcomes for humans, society and ecosystems occurring in the aftermath of a physical phenomenon, i.e. any disturbance, damage, casualties or destruction described in the historical archives and related to a flood event.
The historical analysis of past events is useful for the study of catastrophe as we can hypothesize that these remarkable events are etched in the community's memory (Papagiannaki et al., 2013). Indeed, it is because these events have impacted society that they are recorded in the historical records, i.e. have left a “social signature”. Those high-impact events can come close to the notion of a catastrophe as they can lead to societal upheaval (Soanes and Stevenson, 2009), sometimes deleterious but also beneficial (behavioural change promoting prevention) (Garnier, 2017). High-impact events are by nature rare, often resulting in a lack of available data (e.g. description of the event, time, extent, damages caused). However, historical analysis allows a social and spatial-temporal contextualization of the data (Giacona et al., 2017), making the reconstruction (date, impacts) of major flood events possible (Barriendos et al., 2003, 2019) and attesting to the social apprehension of the phenomenon (Gil-Guirado et al., 2016).
Numerous historical databases were built to document past flood occurrence and magnitude, such as the Prediflood database (Barriendos et al., 2014), and some, like the database from Thoumas (2019), allow us to analyse the climatic fluctuations. In contrast to these latter databases focusing on hydrological events, some databases gather information on the socio-economic impacts of floods such as the APAT database (Lastoria et al., 2006), the press database on natural hazards and climate change from Llasat et al. (2009), the database of high-impact weather events in Greece from Papagiannaki et al. (2013), the EUFF database (Petrucci et al., 2019) and the SMC-Flood database (Gil-Guirado et al., 2019). Some databases stand out like the participative flood database ORRION (Giacona et al., 2019), the online information resources of the Chronology of British Hydrological Events (Black and Law, 2004) or the database built by the RISC-KIT project (Garnier et al., 2018) which aim at developing methods and management approaches and explore trajectories of vulnerability.
To our knowledge there are no similar works published about flood impacts identified in the archives over an historical timescale (i.e. over longer time periods with only the last few decades covered by instrumental data) and in a mountainous catchment. There are numerous databases on flood impacts, but most of them refer either to the instrumental period (Schlögl et al., 2021) or to other hazards than flooding (Zgheib et al., 2020; Papagiannaki et al., 2013; Giacona et al., 2017) or cover a larger area (Barriendos et al., 2014; Macdonald and Sangster, 2017).
Floods, as natural hazards, are naturally occurring physical phenomena and can, when certain conditions are met, cause harm to societies. They can be interpreted as a social construction (Beck, 1992) since exposure of human activities and social vulnerability play a large role in the severity of the impacts. Flood impact databases, constituted of historical records, can be considered as the expression of society's concerns, risk perceptions (fear, habit) and values (based on reported impacts). The recording of flood impacts, or the failure to record them, provides a subjective measure of the events that were considered worth reporting for various reasons across historical periods. Flood impacts result from the interaction between the natural phenomenon and the dynamics of exposure and vulnerability. As vulnerability we understand the potential of various exposed goods, activities or people constituting a given territory to be damaged (Leone and Vinet, 2006). We consider the vulnerability as a dynamic system articulated to numerous physical and societal factors (Antoine, 2011; Terti et al., 2015). This system can evolve in time and space (Cutter, 2003). Major natural disasters, such as floods, are often displayed as unforeseeable events, whereas the historical facts give evidence of the contrary (Garnier, 2016, 2019). Yet society's vulnerability may increase as past disasters are forgotten, leading to a “society of risk” (Garnier, 2019). The historical approach allows consideration and exploration of the trajectories of hazard and vulnerability in response to changes in climate, land use and flood risk management (Gil-Guirado et al., 2016).
The present paper introduces a newly constituted database of flood impacts of the Arve River and its tributaries (northern French Alps). The database called “Historical Impacts of Floods in the Arve Valley” (HIFAVa) covers all impacts caused by hydrological events that have occurred since 1850.
The study of this database, probably the first one documenting flood impacts over an historical timescale in a mountainous catchment, ultimately aims at analysing the interactions between social and natural dynamics engendering these impacts. In this paper we present the dataset and first results of the impacts analysis with respect to their nature and evolution in both time and space.
2.1 Description of the physical setting of the Arve River
The Arve River is located in the northern French Alps (Fig. 1), flowing from the high elevations of the Mont Blanc summit (4810 m a.s.l.) to the Swiss lowlands (330 m a.s.l.), where it flows into the Rhône River. The surface area of its catchment is 2164 km2 with the largest part higher than 1000 m a.s.l. The main tributaries of the Arve River are the Giffre, the Borne, the Menoge and the Foron rivers.
Since 1850, i.e. the start date of the studied period, almost all the current diking systems were already in place (Mougin, 1914; Gex, 1924; Peiry and Bravard, 1989; ACTHYS-Diffusion, 2017), and from 1880 onward, most of the dyke construction work was completed, and their nature did not significantly change after this date. As shown by the study of ACTHYS-Diffusion (2017), most of the 21st century's development of the diking systems of the Arve and the Borne rivers protecting the city of Bonneville concern the construction of weirs to fight against generalized stream incision due to the important extraction of materials in the rivers. Some repair works were carried out on dikes during the 20th century and new works (development of weirs, raising of dikes) only happened at the beginning of the 2000s. Unfortunately, this study is limited to the area of Bonneville and has not been replicated in the rest of the territory.
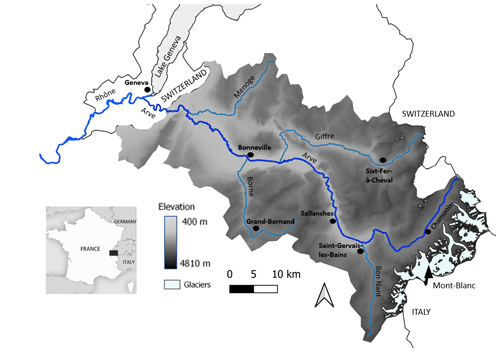
Figure 1The Arve catchment location, topography, main hydrological network and the studied cities (sources: IGN, 2017, 2015; SITG, 2020; GADM, 2018).
Due to large differences in altitude between high- and lowlands, the Arve flows can be defined by two hydrological regimes following an upstream to downstream continuum:
-
The upstream part of the catchment (down to the city of Sallanches; Fig. 1) has a glacio-nival regime due to the numerous glacial tributaries flowing from the Mont Blanc massif (Viani et al., 2018). Low flows occur in winter and early spring (December to March) and high flows in summer (maximum in July and August) because of the strong contribution of ice melt (Bernard, 1900). Floods mainly occur in summer due to the synchronicity of both ice melt and intense sub-daily rain storms. In this part of the catchment, the flood plain is narrow, and the slope inclination is high.
-
At lower elevations (i.e. downstream Sallanches) the regime becomes more nival downward. Low waters mainly occur in winter and reach the highest levels between late spring and early summer with the snowmelt. Between Sallanches and Bonneville, floods mainly occur in summer and autumn due to the conjunction of intense rain storm, snowmelt and, to a lesser extent, ice melt contribution. Downstream of Bonneville, floods occur at any time of the year due to various hydro-meteorological interplays. The valley floor is wide and may be affected by extended flooding.
2.2 Socio-economic setting and land use
There are 106 municipalities located in the Arve catchment, with major population growth since 1850. For instance, the population has been multiplied by a factor of 3 in about 170 years at Chamonix (2304 to 8611 inhabitants between 1848 and 2016) and by a factor of 34 in Annemasse (1047 to 35 712 inhabitants between 1848 and 2017) (INSEE, 2019). These numbers also hide large seasonal variations related to tourism activities. This is particularly the case in Chamonix where the number of residents increases up to 10 times in high season. Most inhabitants live in the valley floor and foothills with most of the farming, industrial and tourism activities, as well as the main transportation routes and urban areas. Since 1965 and the opening of the Mont Blanc Tunnel highway, the Arve valley has been a major trans-Alpine route connecting France and Italy.
The socio-economic setting of the valley follows an upstream–downstream distribution pattern. The period from 1850 to 1913 experienced great tourist development (thermal bath of Saint-Gervais-les-Bains and mountaineering in Chamonix). The economy around Chamonix is essentially based on mountain tourism. In 1921, 250 000 tourists visited Chamonix each year (Gex, 1924). In 2015 the lodging capacity in the valley reached about 416 400 equivalent tourist beds. This part of the valley has undergone rapid urbanization. In 1804, the discovery and exploitation of spring water for hydrotherapy in Saint-Gervais-les-Bains (Gex, 1924) fostered the construction of tourist accommodations. Around Bonneville, the valley is a densely populated corridor characterized by an old metal working industry, born from the manufacture of watches and nearby hydropower resources. The smaller valleys of the Arve tributaries are sparsely populated, and the economy is based on tourism and farming. Due to the attractiveness of the city of Geneva, the valley from Bonneville to the Rhône River confluence is characterized from the 1960s onwards by a major population growth, extended industrial areas and strong urbanization. Between the town of Cluses and Geneva the valley floor is almost continuously built-up.
3.1 Collecting data from historical archives
Mélo et al. (2015) undertook historic research to develop a flood chronology and hydro-meteorological circumstances of the flooding events which happened in the Arve catchment between the 18th and the 21th century. As sources are more abundant and richer in information over the last 165 years (1850–2015), this period was defined as the studied time frame of the HIFAVa database. Only events that triggered impacts and that were mentioned in at least two sources were integrated in the database. Since 2015, data have been further collected to complete the preliminary dataset from Mélo et al. (2015).
Information on flood-related impacts were collected from various sources. Primary sources range from handwritten archives like municipal acts to departmental archives (e.g. reports of the Préfecture and of town councils). Secondary and tertiary sources are respectively made up of published documents (newspapers, reports, books) and pre-existing databases. The database of historical records providing a chronological and synthetic layout of the data is composed of the following (Fig. 2 and Table A1):
-
Manuscript materials. Most of the manuscripts are kept in the departmental archives (Archives Départementales de Haute-Savoie: ADHS) or in the municipal archives (Archives municipals: AM) of the towns of Chamonix, Cluses and Bonneville. The departmental and municipal archives also contain records older than the Savoy annexation by France in 1860. The records can be private or from a public institution.
-
Printed materials. Articles from scientific journals and books used in this study have been published since 1914. They mostly correspond to analyses of the regional hydrology (Mougin, 1914; Mestrallet, 1986) but also focus on single hydrological events (Pardé 1931; Rougier, 2002; Goy, 2002) and risk assessment (Douvinet et al., 2011).
Open-access online municipal risk prevention plans (Plan de Prévention des Risques: PPR; and Plan de Prévention du Risque Inondation: PPRI) (Préfecture de la Haute-Savoie, 2021) as preventive regulatory documents have been used to delineate risk areas and often compile historical flood events that affected municipalities.
-
Newspapers. Most of the newspapers used are regional, but one is published at national level (Le Figaro) and another is printed in Geneva, Switzerland (Le Journal de Genève). Most of the newspapers can be found online or are kept in the departmental or municipal archives. Newspapers describe the causes and consequences of the flood events and sometimes provide instrumental data and illustrations. They also contain information concerning the public authority response and past discussions.
-
Other records. The national database of historical flooding (Base de Données Historiques sur les Inondations: BDHI) gathering floods events considered as “remarkable” in the French territory (Ministère de la Transition Ecologique, 2015; Boudou, 2015) was also used.
The database created by the department of Restoration of Mountainous Areas (Restauration des Terrains en Montagne: RTM) from the public institution managing the French public forests (Office National des Forêts: ONF). The ONF–RTM database gathers transcriptions of observations of the RTM officials, as well as information collected from diverse primary sources. These data (labelled RTM in the HIFAVa database) are freely available through the open-access and online ONF–RTM database (RTM and ONF, 2012). Some specific ONF–RTM reports are also included in the HIFAVa. The RTM database was built to assist the management of small tributaries.
A movie made in 1990 by the RTM is also mentioned as a source.
Some records are from the Syndicat Mixte d'Aménagement de l'Arve et de ses Affluents (SM3A), which has been the institution in charge of the management of the Arve River and its tributaries since 1994.
3.2 Characteristics of the HIFAVa database
The database has been built using the free and open-source relational database management system PostgreSQL and is accessible through its package pgAdmin.
HIFAVa contains 916 distinct flood impacts caused by 321 flood events. The primary key is the impact ID. Therefore, each impact is recorded as a unique line and described through various variables (Tables 1 and A2). The river that triggered the flood is mentioned when possible (94 % of cases). For instance, no river name has been attributed to the impacts related to overland flow in January 1979. The accuracy of the impact location varies from specific addresses (house, bridge, neighbourhood) to the municipality scale. When the source is not accurate enough to distinguish distinct locations of several impacts, they are all referenced under a unique impact ID. In other words, sometimes numerous impacts caused by a single flood event are registered under a distinct ID because it was possible to localize each impact precisely (at the hamlet scale). Sometimes we can only localize the impacts at the municipality scale, meaning that all impacts are registered under the same ID. The severity of an event can not be estimated by the number of ID registered in the database. The most recent sources are often highly informative, allowing impacts to be more precisely located.
Impacts occurring on the same day on a given river are expected to be caused by the same flood event. As a result, the date is the key used to connect each impact to a flood event. This “flood event” definition has been extrapolated to impacts occurring on the same day in different catchments, assuming that two impacts occurring the same day can be caused by the same hydrometeorological event given the moderate surface area of the Arve catchment. The accuracy of the date is rated on a certainty scale (hour, day, month, year). Based on information contained in the records, we distinguish when possible the hydrometeorological events (e.g. rainfall, intense and short rainfall, melting of snow, frozen soil, glacial outburst, wet period before the event) which caused the flood and the different flood types (e.g. river flooding, overland flow, sediment transport) leading to the impacts.
3.3 Text mining
The flood impacts of the HIFAVa were categorized through a text quantitative content analysis with the KH Coder software (Higuchi, 2015). The description of the impacts comes from comments contained in the records. The most frequent words were gathered in order to determine representative categories of the database content. A category is made of several words assigned to a coding rule. Categories were inspired by the flash flood impact severity scale of Diakakis (2020). This analysis led to the following seven categories with examples of the assigned words:
-
transport network: e.g. “road”, “bridge”, “railway”, “street”;
-
urbanized areas and residential buildings: e.g. “house”, “town”, “basement”;
-
natural environment: e.g. “forest”, “field”, “yield”, “sediment transport”;
-
protection infrastructures and dams: “dikes” and “dam”;
-
industrial, commercial and recreational facilities: e.g. “mill”, “factories”, “golf”, “camping”, “hotel”, “school”;
-
critical installations: “drain”, “power transformer”;
-
victims: “dead”, “injured”, “missing”, “evacuee”.
In this section, we present the detailed content of the database, the results of the preliminary analyses and the first key findings.
4.1 Evolving sources over time
During the studied period, the diversity and the quantity of sources in which mentions of impacts were found fluctuate (Fig. 2). Among the existing databases used, the BDHI database for instance continuously covers the studied period but was sporadically informative since it only contains two mentions of impact events in the Arve catchment: the Bon Nant River in 1892 and the Borne River in 1987 (Fig. 2a). By contrast, the SM3A database appears later (1979) in the studied time frame.
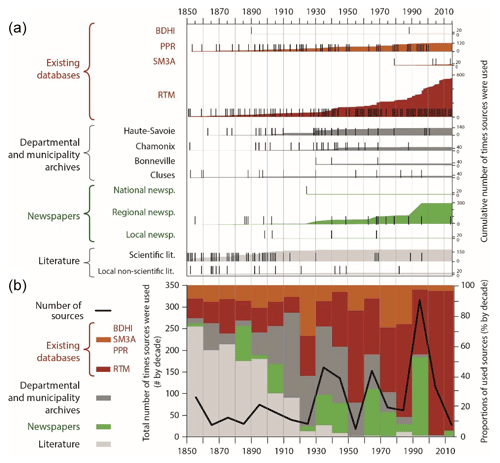
Figure 2(a) Number and (b) percentage of mentions from the studied sources documenting flood impacts since 1850 in the Arve valley. In panel (a), the ticks indicate each mention of impacts, and the coloured areas display the cumulative number of mentions.
RTM (38 % of the mentions from all sources), regional newspapers (20 %) and scientific literature (13 %) constitute the main sources of information on past flood impacts throughout the studied time period. Sources from the literature are particularly useful (54 %) to document the period between 1850 and 1910. Then, materials from the departmental and municipality archives are abundant between 1900 and 1970, especially those from Haute-Savoie (20 % of the total registered sources for this period) and Chamonix (7 %). Between 1940 to 2015, the RTM represents 58 % of the mentions from all sources describing the impacts (Fig. 2b).
One of the evolutions of the sources is the increase in newspaper articles mentioning flood impacts. Following the 1881 French press freedom law, the 1880–1889 decade marks the emergence of articles recording natural hazards, such as flood impacts (Ferenczi, 1996). However, the 1855 flood in Bonneville was already reported by the Swiss newspaper, Le Journal de Genève.
Although a few sources (e.g. the municipal archives of Sallanches) remain to be examined, we consider that most of the main sources (newspapers, existing databases and public archives) have been analysed. As a result, we are confident that no event that was deemed damageable by local communities was missed, and we consider that we have a comprehensive view of past flood impacts since 1850 over the whole Arve catchment.
4.2 Changes in impacts over time and space
From 1850 to 1920, the number of impacts fluctuates, and only 4 years are considered remarkable with more than 15 impacts (1852, 1878, 1895 and 1910). From 1920, years with 15 or more impacts become more frequent (1930, 1940, 1944, 1968, 1979, 1987, 1990, 1996, 1997 and 2007), and the total amount of impacts per year reaches the maximum value of 54 in 1996 (Fig. 3). The decennial moving average of the impacts' number highlights an overall increase over the 165 years, punctuated by periods with less frequent impacts (in 1910–1923, 1950–1960 and 1975–1980).
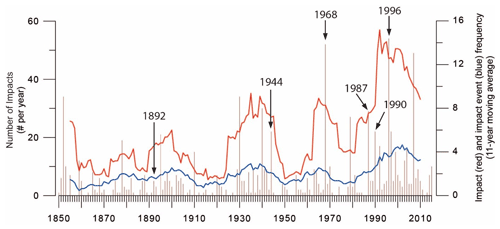
Figure 3Yearly occurrence of impacts and decennial moving averages of impacts (red curve) and associated impact events (blue curve). Events discussed in the article are labelled with their year of occurrence.
The number of recorded impact events – i.e. flood events recorded in the historical sources because of the impacts they caused – stays relatively stable between 1.5 and 3 events per year on average until 1990; then it rises up to 4.5 events per year. As hydrological data are available from 1950 for the Arve River, it is not possible to assess whether the increase in impact events was a function of increased flood occurrence or changes in vulnerability or recording.
When analysing the spatial distribution of the flood impacts, we can see that they are spread over the entire catchment (Fig. 4a). Chamonix and Bonneville experience, however, respectively 24 % and 12.5 % of total impacts recorded in the Arve catchment. These high numbers may be due to the fact that these towns are both among the most densely populated and the closest to the Arve River. The impacts caused by the Arve River floods represent 33 % of all recorded impacts, and its two main tributaries, the Giffre and the Borne rivers, have only caused 8 % of the recorded impacts (Fig. 4b). In fact, most impacts are due to small torrential streams (53 %). Among them, almost a third are related to glacial tributaries, while these tributaries are localized only in the uppermost part of the catchment near Chamonix. For instance, small torrential tributaries such as the Arveyron, the Grépon (left bank tributary close to Chamonix) or the Bon Nant alone caused more impacts than the Borne River itself.
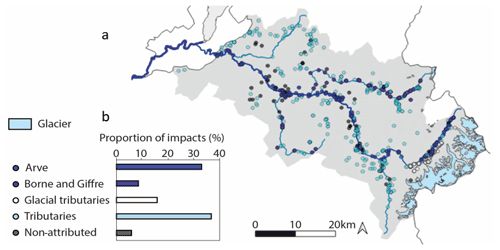
Figure 4(a) Location and (b) distribution of flood impacts caused by the Arve River and its tributaries. The category “non-attributed” corresponds to the impacts for which it was not possible to attribute a river either because events are related to overland flows or because the source did not mention the river (sources: IGN, 2015, 2017; SITG, 2020; GADM, 2018; HIFAVa).
The Arve tributaries produced disasters characterized by numerous instances of major flood damage. Among them, the 1987 Borne River flooding in its uppermost part washed away the municipal campsite of the village of the Grand-Bornand, causing 23 casualties and heavy economic losses (Meunier, 1990). In addition, the 1892 glacial lake outburst from the Tête Rousse glacier in the Bon Nant River (which literally translated means “Good Stream”) swept away the thermal bath of Saint-Gervais-les-Bains (Fig. A1) and 33 houses, causing at least 175 casualties. The glacier was drained in 2010 and is today closely monitored to avoid such a brutal and disastrous natural event (Garambois et al., 2016; Vincent et al., 2012).
All these high-impact events are due to sudden, highly dynamic summer floods of tributaries, often aggravated by large sediment transport. Some towns located along the Arve River – such as Sallanches – are more prone to tributary floods because embankments have been built and efficiently prevent impacts from the Arve. In contrast, there are very few impacts recorded in high altitude locations probably due to the sparsity of human settlements.
4.3 Potential drivers of changes in the number and distribution of impacts
The increase in the number of impacts starting in the 1920s and well-marked from the 1960s onwards can be explained by multiple factors, such as an increase in flood occurrence and/or magnitude, a source effect, an increase in exposure of goods and people, a deterioration of the diking systems, a break in the risk memory transmission, an evolution of the risk perception or an evolution of the local political risk management. Due to the lack of available data regarding changes in flood hazard, protection infrastructures, and the risk memory, perception, and management, we only explore whether source effect and changes in exposure may explain the observed increase in impacts.
To decipher the potential source effect in the increase in impacts particularly noticeable since 1960, maps of the impacts by sources have been drawn for the periods before and after 1960 (Fig. 5). In addition to the noticeable increase in impacts, this date marks a strong change in the Arve valley economy, from a rather homogeneous agricultural society to an industrial society exploiting the river bed for material extractions. After 1960, the Arve watershed also experienced a strong tourist development and a rapid demographic expansion. From 1850 to 1959, three main sources describe 64 % of the impacts (literary records 28 %, RTM 18 %, and departmental and municipal archives 18 %), and for 29 % the information comes from more than one source. The impacts are mainly gathered along the Arve and the Giffre rivers, especially in the valley of Chamonix and between the towns of Cluses and Bonneville.
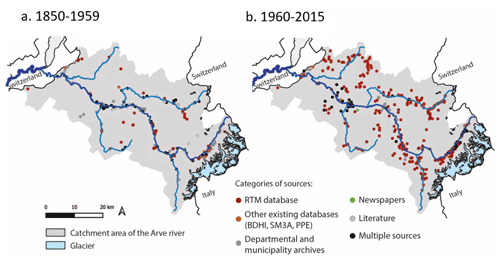
Figure 5Comparison of the spatial distribution of impacts by categories of sources in the Arve catchment during the periods (a) 1850–1959 and (b) 1960–2015 (sources: IGN, 2015, 2017; SITG, 2020; GADM, 2018; HIFAVa).
For the second period (1960–2015), the RTM reports 65 % of the impacts, and 20 % come from multiple sources, while departmental and municipal archives and the PPR/PPRI describe 5 % each. Information coming only from literature sources decreases substantially (122 described impacts in the first period to 3 impacts in the second); SM3A records start in 1979 and only document the Giffre and the Bon Nant rivers. The distribution of the impacts is much more scattered across the whole catchment than during the first period (Fig. 5). The impacts are not gathered along the Arve River since most of them result from small tributaries. Impacts described by more than one source are located in the valley of Chamonix and around Bonneville probably because these economic and tourist centres arouse interest in many sources (newspapers, departmental and municipal archives, and RTM). In addition, the strong emergence of the RTM from 1940 onwards (Fig. 2) can explain the rise in documented impacts caused by small tributaries (Fig. 5). Following the floods of the Rhône, the Loire and the Garonne in 1854, the 1858 law against urban flooding placed flood control at the heart of the national legislation for the first time. In the following, the RTM department was formed for the reforestation of mountain slopes in order to prevent the recurrence of major floods. The department became quickly efficient and since 1860 has collected numerous reports. Built for the study and management of small tributaries, the RTM database became the main source of information from 1930 onwards for the HIFAVa database. Hence, the strong emergence of the RTM source among the others may have played a role in the observed increase in impacts since 1920, even more noticeable since 1960.
The rise in the number of impacts per flood may be partly explained by the fact that distinct impact types in the same location were reported and therefore referenced under a distinct impact ID, while they were not differentiated in previous periods. Recent sources seem to provide more accurate information on the impacts and their locations. In older sources, impacts are most of the time documented at the city scale (21 % of the impacts for the first period and 10 % for the second period). Thereby, all these impacts are stored in the database in a single line with an uncertainty code for the impact location corresponding to the municipal level. In most recent sources, impacts' locations are described more accurately, allowing them to be recorded at a resolution up to the building scale. As a result, impacts are stored in as many lines as impact locations can be identified, with an uncertainty code for the impact's location corresponding to the building or neighbourhood level (85 % of the impacts for the second period). For example, in 1996, 53 impacts were recorded for the same event, and 50 of them were located in distinct places in Chamonix. The rise in impacts can also be due to numerous impacts in different locations, like the flood of 1990 which impacted six towns in two different sub-catchments (the Arve and the Giffre catchments). However, in order to overcome the bias induced by the recording of impacts according to their location, we aggregated the impacts at the municipality level. That is to say, all the impacts reported for a given municipality that were caused by the same event (thus the same day) are recorded under the same line in the database. This results in 562 “aggregated” impacts instead of 916 impacts initially. From these data, we redraw Fig. 3 (Fig. A2) comparing the moving average of impacts and associated events. We can see that in both figures the trends of increasing impacts are similar. There is an increase in impacts (here starting as soon as the 1890s). Thus, the way the impacts are stored in the database (by location or by municipality) affect the absolute values of impact per year but not the observed temporal changes over time.
Changes in exposure and vulnerability related to land use are another potential explanation of the rise in the number of impacts (Magnan et al., 2012; Garnier and Desarthe, 2013; Camuffo et al., 2020). In fact, major population growth happened, especially in Bonneville and Chamonix, and led to a strong and fast urban sprawl in the flood plain between the 1950s and the 2010s as shown by aerial photographs (Fig. 6). They also show the vanishing of the alluvial forest (Dufour and Piégay, 2006) and cultivated fields to the benefit of urbanization in both towns. Upstream, in Chamonix, the demographic expansion dates back to the early 20th century with the flourishing of mountain tourism. In downstream towns – e.g. Bonneville and Annemasse – the expansion starts in the 1950s because of the economic attractiveness of Geneva.
Besides these numbers, the urban expansion and the growth in tourism entail the arrival of new residents in the valley (INSEE, 2020) unaware of the local hazard history. The valley narrowness, the demand for land and the loss of memory of past events have led to a rise in construction within historical flood-prone areas, resulting in an increased exposure. For instance, in 1944 recently built houses in Chamonix were washed away by the Grépon River. The same situation also happened during the 1968 flood that destroyed a new residential area in Bonneville. To explore to what extent the urban growth may have resulted in an increased exposure explaining the observed rise of impacts, we use the population growth data as a first-order proxy of urban growth. In Chamonix, the number of impacts increases from year to year, somehow mirroring the population growth (Fig. 6). Therefore, population exposure might be one explanation for the increased number of impacts. One can, however, notice the decrease in impacts in the early 2000s due to the heightening of the dikes after the 1996 flood. In Bonneville, the link between the number of impacts and the population growth is not as clear as in Chamonix. This may partly result from the absence of major floods since 1968. Therefore, an increasing exposure might locally explain the increase in impacts. Further studies should however reconstruct diachronic maps of land use to assess in a finer way the urban growth in flood-prone areas and its link with changes in the number of impacts. Overall, the potential role of an increased exposure is not excluding the indirect source effect (emergence and dominance of the RTM source), but both factors can be combined and complement each other.
4.4 Nature of the flood impacts
The quantitative analysis of text content reveals the distribution of the impact categories by river and illustrates the diversity of the catchment in terms of land use and economic development (Fig. 7). This analysis of text content is particularly relevant because it allows us to overcome the database scarcity of quantitative information on the severity of the flood. Indeed, it is difficult to estimate the severity of a flood event as the flow rate and water height are only mentioned in rare cases. However, according to Barriendos et al. (2019) a link can be made between the nature of the impacts and the severity of the triggering phenomena. In order to establish this link, we also need to consider the various dynamics of the flooding phenomena as fast flooding generally affects smaller surfaces but in a more violent manner than slow floods of the main rivers. Therefore, they may trigger a lower number of impacts, but the level of destruction of the impacted element might be more important. Of course, the level of destruction also depends on the sensitivity and physical vulnerability of the exposed element; it is why categorizing impacts by their nature might help in exploring the question of the flood severity.
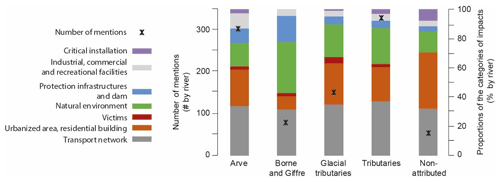
Figure 7Distribution of flood impact categories according to the river types. The class “non-attributed” gathers the impacts that could not be assigned to a river (e.g. overland flows).
The categories used for the analysis are partly inspired from a recent paper from Diakakis (2020) and refined based on textual analysis of the words used to describe the impacts in the database. The number of occurrences of the words determining the category is here named as the number of mentions. For the overall Arve catchment, damage to the transport network is the most frequent (406 mentions), followed by damage to urbanized areas (286) and the natural environment (253). Impacts on the transport network are in proportion equally distributed among river types (all around 30 %). Impacts on industrial facilities are mainly caused by the Arve and the Giffre rivers as their wider valleys allow for the installation of these facilities. The Giffre and the Borne rivers have caused the least impacts specifically on urban areas probably because of less dense population and of an economy based on farming and tourism activities.
Most of the mentions of victims refer to impacts caused by tributaries (20 out of 28) characterized by faster hydrological responses. They have caused 80 % of the deadly impacts registered since 1850 in the whole catchment, e.g. the 1892 glacial lake outburst and the 1987 flood of the Borne River in Grand-Bornand. The mentions of victims of the Borne River should belong to the small tributary class as the impacts occurred in the uppermost part of the catchment. As shown by Jonkman et al. (2005) and Ruin et al. (2008) high mortality rates are mostly due to the suddenness and violent responses of small catchments affecting people in the open air (like for campers).
To track potential changes in the nature of impacts since 1850, they are scrutinized by decade over the last 165 years (Fig. 8). Impacts on transportation networks have been present in every decade since 1850, but they increase after 1930, as well as impacts on urbanized area and natural environment categories. It was not until 1960 that impacts on the industrial, commercial and recreational facilities category increased. Mentions about critical installations (sewers and water pipes) are recorded for the first time in the 1960s. Mentions of victims are present in almost every decade.
Nevertheless, no major evolution of the impacts' categories can be seen except the emergence of mentions of critical installations in the 1930s. A more in-depth analysis will be conducted later on to define severity classes based on the nature of the impacts and also to identify whether there is an evolution of the lexicon used to describe the impacts.
The data representation of Fig. 8 does not allow us to visualize the evolution of the absolute values. For instance, at the catchment scale there is an increase in the number of mentions of the victim category with 18 mentions during the period 1960–2015 compared to 10 for the period 1850–1959 (Fig. 8). This increase is not easily noticeable when looking at proportions of impact categories because of the strong augmentation in the total number of mentions since 1930. Yet, apart from the disaster in the Grand-Bornand in 1987 (Borne River), since 1931 all the mentions of victims have referred to evacuations, rescues or injuries.
The number of impacts has been almost multiplied by 2 since 1920. Mentions of impacts on urbanized areas during 1960–2015 have been multiplied almost by 4 compared to the 1850–1930 period. This agrees with the evolution of the land use due to the demographic growth, i.e. the observed vanishing of forest areas and cultivated land to the benefit of urbanization (Fig. 6). The mentions of impacts to the natural environment for the period 1850–1930 are more than double compared to the period 1960–2015. During the first period, mentions of impacts to the natural environment refer mainly to forest, field and crops, while after 1960 there is no mention of field or crops, and most of the mentions are about gullying, deposition of sediments and banks.
This paper describes and analyses historical data documenting 916 flood impacts associated with 327 flood events that occurred between 1850 and 2015 in the Arve valley, an Alpine catchment characterized by a high hydrological and socio-economic diversity. This dataset is stored in the HIFAVa database fully described in this paper.
A first spatial and temporal analysis of this dataset allowed us to identify three key findings and research perspectives:
-
First is the predominance of impacts due to torrential tributaries. There are two main types of flood events causing impacts in the Arve catchment, i.e. floods related to the main river and those related to the smaller mountain streams. Floods from these small streams are characterized by sudden and fast hydrological responses and most of the time by high volumes of sediment transport, making hazard management difficult. They caused two thirds of the 916 recorded impacts with numerous casualties, such as the Bon Nant (1892) and the Borne (1987) river floods. In contrast, slow rising, day-long floods of the Arve River affect larger areas and trigger large economic consequences but no casualties. The 1968 flood event affecting a large part of the Arve catchment is an exemplary case of this flood type. As suggested by Ruin et al. (2008), the number of impacts caused by torrential streams being much higher than those triggered by the main rivers calls for a greater attention to flood risk assessment and management in small catchments.
-
Second is the rise in the number of impacts starting in 1920 and well-marked from 1960 onwards. This increase in impacts may be explained by various factors. Based on the available data, we discussed the potential source effect and changes in exposure. It appeared that the emergence and dominance of the RTM among the other sources, as well as an increased exposure linked to urban expansion, may play a role at some places. However, exposure was assessed through the population growth as a first-order proxy of urban expansion. A more detailed study of changes in land use based on, for example, old maps and aerial photography is necessary to confirm this preliminary result.
-
Third is the evolution of the impacts' nature (increase in impacts on urbanized area) mirroring the land use changes and probably due to the urban expansion linked to the large demographic growth in the catchment area. Further work is required to explore the evolution of the vocabulary used to describe those impacts across centuries in order to evaluate how this evolution might relate to changes in what the societies value and care for across history. Another path of future research concerns the identification of severity classes based on the description of the nature and extent of the damage in order to be able to characterize the level of impact on a given territory, to allow a classification of past events according to their intensity, and to identify the most significant ones.
Moreover, the other drivers that may have induced the observed increase in impacts still need to be investigated. The lack of gauge data (available only for the main river since 1950) precludes the study of potential links between the increased number of impacts and changes in flood occurrence and/or magnitude over the whole catchment. In contrast, data on the risk memory and its transmission within the society can be acquired and analysed to explore the evolution of the territory's vulnerability through time.
Table A1List of the historical records – or their origin – used to provide information about flood events and impacts collected in the HIFAVa database. The date mentions the year of publication for books and period covered by the newspapers.
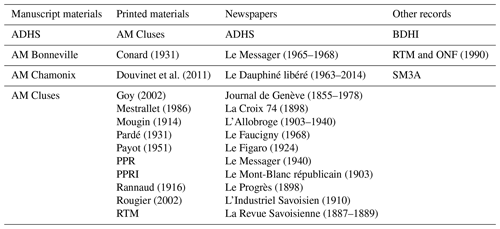
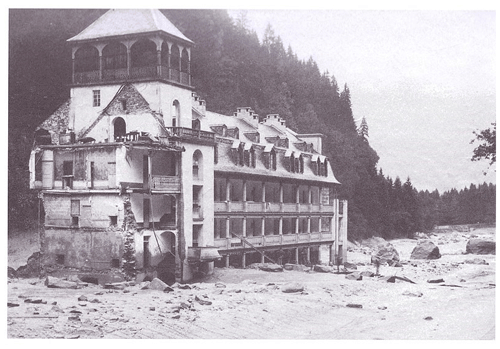
Figure A1The wiped-out thermal bath of Saint-Gervais-les-Bains after the debris flow of the 12 July 1892 (thermal bath establishment of Saint-Gervais, via https://www.thermes-saint-gervais.com/, last access: 24 February 2022).
Raw data were generated at the University of Grenoble Alpes. Derived data supporting the findings of this study are available from the corresponding author Eva Boisson on request.
AM collected the historical data. EB wrote the manuscript with support from BW, IR and EG. SA helped supervise the project. All authors provided critical feedback and helped shape the research, analysis and manuscript.
The contact author has declared that neither they nor their co-authors have any competing interests.
Publisher's note: Copernicus Publications remains neutral with regard to jurisdictional claims in published maps and institutional affiliations.
We would like to thank the Syndicat Mixte d'Aménagement de l'Arve et de ses Affluents who shared its database of events and impacts.
This research has been supported by the RHAAP project funded under the programme Labex OSUG@2020 (grant no. ANR-10-LABX-56) and by the project Labex ITEM CrHistAl. It is also part of the PICS research project and has been supported by the Agence Nationale de la Recherche in the framework of the “Investissement d'avenir” programme (grant no. ANR-15-IDEX-02).
This paper was edited by Sven Fuchs and reviewed by Neil Macdonald and one anonymous referee.
ACTHYS-Diffusion: Etude pour la restauration des systèmes d'endiguement de l'Arve et du Borne, Fiches d'information historique (FIH) par système d'endiguement, report, 304 pp., 2017.
Antoine, J.-M.: Vulnérabilité et adaptation des sociétés montagnardes à la torrentialité au cours du Petit Âge Glaciaire dans les Pyrénées, Journal is Sud-Ouest Européen (SOE), 32, 53–66, https://doi.org/10.4000/soe.685, 2011.
Barriendos, M., Coeur, D., Lang, M., Llasat, M. C., Naulet, R., Lemaitre, F., and Barrera, A.: Stationarity analysis of historical flood series in France and Spain (14th–20th centuries), Nat. Hazards Earth Syst. Sci., 3, 583–592, https://doi.org/10.5194/nhess-3-583-2003, 2003.
Barriendos, M., Ruiz-Bellet, J. L., Tuset, J., Mazón, J., Balasch, J. C., Pino, D., and Ayala, J. L.: The “Prediflood” database of historical floods in Catalonia (NE Iberian Peninsula) AD 1035–2013, and its potential applications in flood analysis, Hydrol. Earth Syst. Sci., 18, 4807–4823, https://doi.org/10.5194/hess-18-4807-2014, 2014.
Barriendos, M., Gil-Guirado, S., Pino, D., Tuset, J., Pérez-Morales, A., Alberola, A., Costa, J., Balasch, J. C., Castelltort, X., Mazón, J., and Ruiz-Bellet, J. L.: Climatic and social factors behind the Spanish Mediterranean flood event chronologies from documentary sources (14th–20th centuries), Global Planet. Change, 182, 102997, https://doi.org/10.1016/j.gloplacha.2019.102997, 2019.
Beck, U.: Risk Society: Towards a New Modernity, Sage, London, 272 pp., ISBN 9780803983465, 1992.
Bernard, C.: Restauration et conservation des terrains en montagne: les terrains et les paysages torrentiels (Haute-Savoie), Impr. nationale (Paris), 70 pp., https://gallica.bnf.fr/ark:/12148/bpt6k6439249q/f1.item.r=arve inondation (last access: 8 March 2022), 1900.
Black, A. R. and Law, F. M.: Development and utilization of a national web-based chronology of hydrological events/Développement et utilisation sur internet d'une chronologie nationale d'événements hydrologiques, Hydrolog. Sci. J., 49, 237–246, https://doi.org/10.1623/hysj.49.2.237.34835, 2004.
Blöschl, G., Kiss, A., Viglione, A., Barriendos, M., Böhm, O., Brázdil, R., Coeur, D., Demarée, G., Llasat, M. C., Macdonald, N., Retsö, D., Roald, L., Schmocker-Fackel, P., Amorim, I., Bělínová, M., Benito, G., Bertolin, C., Camuffo, D., Cornel, D., Doktor, R., Elleder, L., Enzi, S., Garcia, J. C., Glaser, R., Hall, J., Haslinger, K., Hofstätter, M., Komma, J., Limanówka, D., Lun, D., Panin, A., Parajka, J., Petrić, H., Rodrigo, F. S., Rohr, C., Schönbein, J., Schulte, L., Silva, L. P., Toonen, W. H. J., Valent, P., Waser, J., and Wetter, O.: Current European flood-rich period exceptional compared with past 500 years, Nature, 583, 560–566, https://doi.org/10.1038/s41586-020-2478-3, 2020.
Boudou, M.: Approche multidisciplinaire pour la caractérisation d'inondations remarquables: enseignements tirés de neuf évènements en France (1910–2010), thesis manuscript, Geography, Université Paul Valéry -Montpellier I, Lyon, 462 pp., 2015.
Caisse centrale de réassurance (CCR): Les catastrophes naturelles en France, Bilan 1982–2020, 116 pp., https://catastrophes-naturelles.ccr.fr/-/bilan-cat-nat-1982-2020-1 (last access: 8 March 2022), 2021.
Camuffo, D., della Valle, A., and Becherini, F.: A critical analysis of the definitions of climate and hydrological extreme events, Quatern. Int., 538, 5–13, https://doi.org/10.1016/j.quaint.2018.10.008, 2020.
Conard, G.: Morphologie de la vallée de Chamonix et de ses abords, Annales de géographie, 40, 226, https://doi.org/10.3406/geo.1931.11179, 1931.
Cutter, S. L.: The Vulnerability of Science and the Science of Vulnerability, Ann. Assoc. Am. Geogr., 93, 1–12, https://doi.org/10.1111/1467-8306.93101, 2003.
Diakakis, M., Deligiannakis, G., Antoniadis, Z., Melaki, M., Katsetsiadou, N. K., Andreadakis, E., Spyrou, N. I., and Gogou, M.: Proposal of a Flash Flood Impact Severity Scale for the classification and mapping of flash flood impacts, J. Hydrol., 590, 125452, https://doi.org/10.1016/j.jhydrol.2020.125452, 2020.
Douvinet, J., Defossez, S., Anselle, A., and Denolle, A.-S.: Les maires face aux plans de prévention du risque inondation (PPRI), L'espace géographique, 40, 31–46, https://doi.org/10.3917/eg.401.0031, 2011.
Dufour, S. and Piégay, H.: Forêts riveraines des cours d'eau et ripisylves: spécificités, fonctions et gestion, Rev. For. Fr., 4, 339–350, https://doi.org/10.4267/2042/6704, 2006.
Ferenczi, T.: L'invention du journalisme en France, Naissance de la presse moderne à la fin du XIXème siècle, Payot, Paris, 274 pp., ISBN 978-2-228-88998-8, 1996.
Global Administrative Areas GADM: GADM database of Global Administrative Areas, version 2.0, https://gadm.org/ (last access: 8 March 2022), 2018.
Garambois, S., Legchenko, A., Vincent, C., and Thibert, E.: Ground-penetrating radar and surface nuclear magnetic resonance monitoring of an englacial water-filled cavity in the polythermal glacier of Tête Rousse, Geophysics, 81, 131–146, https://doi.org/10.1190/geo2015-0125.1, 2016.
Garnier, E.: Genève face à la catastrophe 1350–1950. Un retour d'expérience pour une meilleure résilience urbaine, Slatkine, Genève, Suisse, 195 pp., ISBN 978-2-8321-0747-8, 2016.
Garnier, E.: Xynthia, February 2010: Autopsy of a Foreseable Catastrophe, in: Management of the Effects of Coastal Storms: Policy, Scientific and Historical Perspectives, John Wiley & Sons, Chichester, UK, 111–148, https://doi.org/10.1002/9781119116103.ch3, 2017.
Garnier, E.: Lessons learned from the past for a better resilience to contemporary risks, Disaster Prev. Manag., 28, 778–794, https://doi.org/10.1108/dpm-09-2019-0303, 2019.
Garnier, E. and Desarthe, J.: Cyclones and Societies in the Mascarene Islands 17th–20th Centuries, AJCC, 2, 1–13, https://doi.org/10.4236/ajcc.2013.21001, 2013.
Garnier, E., Ciavola, P., Spencer, T., Ferreira, O., Armaroli, C., and Mclvor, A.: Historical analysis of storm events: Case studies in France, England, Portugal and Italy, Coast. Eng., 134, 10–23, 2018.
Gex, F.: La Haute-Savoie aujourd'hui et il y a 100 ans avec un tableau de la population par commune de 1801 à 1921, Librairie M. Dardel, Chambéry, 276 pp., https://gallica.bnf.fr/ark:/12148/bpt6k98010284/f1.image.r=arve inondation 1924.
Giacona, F., Eckert, N., and Martin, B.: A 240-year history of avalanche risk in the Vosges Mountains based on non-conventional (re)sources, Nat. Hazards Earth Syst. Sci., 17, 887–904, https://doi.org/10.5194/nhess-17-887-2017, 2017.
Giacona, F., Martin, B., Furst, B., Glaser, R., Eckert, N., Himmelsbach, I., and Edelblutte, C.: Improving the understanding of flood risk in the Alsatian region by knowledge capitalization: the ORRION participative observatory, Nat. Hazards Earth Syst. Sci., 19, 1653–1683, https://doi.org/10.5194/nhess-19-1653-2019, 2019.
Gil-Guirado, S., Espín-Sánchez, J.-A., and Del Rosario Prieto, M.: Can we learn from the past? Four hundred years of changes in adaptation to floods and droughts. Measuring the vulnerability in two Hispanic cities, Climatic Change, 139, 183–200, https://doi.org/10.1007/s10584-016-1768-0, 2016.
Gil-Guirado, S., Pérez-Morales, A., and Lopez-Martinez, F.: SMC-Flood database: a high-resolution press database on flood cases for the Spanish Mediterranean coast (1960–2015), Nat. Hazards Earth Syst. Sci., 19, 1955–1971, https://doi.org/10.5194/nhess-19-1955-2019, 2019.
Giorgi, F., Zsolt Torma, C., Coppola, E., and Ban, N.: Enhanced summer convective rainfall at Alpine high elevations in response to climate warming, Nat. Geosci., 9, 584–589, https://doi.org/10.1038/ngeo2761, 2016.
Gobiet, A., Kotlarski, S., Beniston, M., Heinrich, G., Rajczak, J., and Stoffel, M.: 21st century climate change in the European Alps – A review, Sci. Total Environ., 493, 1138–1151, https://doi.org/10.1016/j.scitotenv.2013.07.050, 2014.
Goy, J.: Autour de la catastrophe de l'établissement thermal de Saint-Gervais-les-Bains en 1892: problèmes d'histoire des catastrophes naturelles., Les pouvoirs publics face aux risques naturels dans l'histoire, MSH-Alpes, Grenoble, 39–49, ISBN 2-914242-08-5, 2002.
Higuchi, K.: KH Coder, https://khcoder.net/en/ (last access: 28 February 2022), 2015.
Institut national de la statistique et des études économiques (INSEE): Historique des populations légales. Recensements de la population 1968–2019, https://www.insee.fr/fr/statistiques/2522602 (last access: 28 February 2022), 2019.
Institut national de la statistique et des études économiques (INSEE): Haute-Savoie : la plus forte croissance démographique de métropole, https://www.insee.fr/fr/statistiques/3689656, last access: 1 August 2020.
Institut National de l'information Géographique (IGN): GEOFLA, IGN [data set], https://geoservices.ign.fr/geofla, 2015.
Institut National de l'Information géographique et forestière (IGN): BDCarthage, IGN [data set], http://services.sandre.eaufrance.fr/telechargement/geo/ETH/BDCarthage/FXX/2014/ 2017.
IPCC: Managing the risks of extreme events and disasters to advance climate change adaptation, A Special Report of Working Groups I and II of the Intergovernmental Panel on Climate Change, Intergovernmental Panel on Climate Change, 582 pp., https://doi.org/10.13140/2.1.3117.9529, 2012.
IPCC: The Ocean and Cryosphere in a Changing Climate, Intergovernmental Panel on Climate Change, Genève, Suisse, 765 pp., in press, 2019.
Jonkman, S. N. and Kelman, I.: An Analysis of the Causes and Circumstances of Flood Disaster Deaths: An Analysis of the Causes and Circumstances of Flood Disaster Deaths, Disasters, 29, 75–97, https://doi.org/10.1111/j.0361-3666.2005.00275.x, 2005.
Lastoria, B., Simonetti, M. R., Casaioli, M., Mariani, S., and Monacelli, G.: Socio-economic impacts of major floods in Italy from 1951 to 2003, Adv. Geosci., 7, 223–229, https://doi.org/10.5194/adgeo-7-223-2006, 2006.
Leone, F. and Vinet, F.: La vulnérabilité, un concept fondamental au cœur des méthodes d'évaluation des risques naturels, in: La vulnérabilité des sociétés et des territoiresface aux menaces naturelles, GESTER, 9–27, ISBN 2-84269-727-8, 2006.
Llasat, M. C., Llasat-Botija, M., and López, L.: A press database on natural risks and its application in the study of floods in Northeastern Spain, Nat. Hazards Earth Syst. Sci., 9, 2049–2061, https://doi.org/10.5194/nhess-9-2049-2009, 2009.
Macdonald, N. and Sangster, H.: High-magnitude flooding across Britain since AD 1750, Hydrol. Earth Syst. Sci., 21, 1631–1650, https://doi.org/10.5194/hess-21-1631-2017, 2017.
Magnan, A., Duvat, V., and Garnier, E.: Reconstituer les “trajectoires de vulnérabilité” pour penser différemment l'adaptation au changement climatique, Nat. Sci. Soc., 20, 82–91, https://doi.org/10.1051/nss/2012008, 2012.
Mélo, A., Wilhelm, B., Giguet-Covex, C., and Arnaud, F.: Construire une chronique d'inondations: évènements hydrologiques et histoire climatique dans le bassin de l'Arve (Alpes du Nord, France) entre les XVIIIe et XXIe siècles, Revue archéologique de l'est, 40, 411–419, 2015.
Ménégoz, M., Valla, E., Jourdain, N. C., Blanchet, J., Beaumet, J., Wilhelm, B., Gallée, H., Fettweis, X., Morin, S., and Anquetin, S.: Contrasting seasonal changes in total and intense precipitation in the European Alps from 1903 to 2010, Hydrol. Earth Syst. Sci., 24, 5355–5377, https://doi.org/10.5194/hess-24-5355-2020, 2020.
Merz, R. and Blöschl, G.: Regional flood risk – what are the driving processes?, in: Water Resources Systems – Hydrological Risk, Management and Development, International Association of Hydrological Sciences, 40–58, ISBN 1-901502-32-5, 2003.
Mestrallet, M.: Des torrents et des hommes, Trois siècles d'histoire à Samoëns, Imprim'off7, Marignier, 150 pp., ISBN 9782950146700, 1986.
Meunier, M.: La catastrophe du Grand Bornand: crue torrentielle du Borne le 14 juillet 1987, Rev. Géogr. Alp., 78, 103–114, 1990.
Min, S.-K., Zhang, X., Zwiers, F. W., and Hegerl, G. C.: Human contribution to more-intense precipitation extremes, Nature, 470, 378–381, https://doi.org/10.1038/nature09763, 2011.
Ministère de la Transition écologique: Prévention des risques naturels, https://www.ecologique-solidaire.gouv.fr/prevention-des-risques-naturels (last access: 8 March /2022), 2020.
Ministère de la Transition Ecologique: Base de Données Historiques sur les Inondations, https://www.ecologique-solidaire.gouv.fr/prevention-des-risques-naturels (last access: 8 March 2022), 2015.
Mougin, P.: Les torrents de la Savoie, Société d'histoire de la Savoie, Grenoble, 1251 pp., ISBN 9782842061746, 1914.
Papagiannaki, K., Lagouvardos, K., and Kotroni, V.: A database of high-impact weather events in Greece: a descriptive impact analysis for the period 2001–2011, Nat. Hazards Earth Syst. Sci., 13, 727–736, https://doi.org/10.5194/nhess-13-727-2013, 2013.
Pardé, M.: Les crues de l'Arve en octobre 1930, Rev. Géogr. Alp., 19, 495–497, https://doi.org/10.3406/rga.1931.4782, 1931.
Payot, P.: Au royaume du Mont-Blanc, Imprimerie Plancher, Bonneville, 305 pp., 1951.
Peiry, J. L. and Bravard, J. P.: Evolution naturelle d'un remplissage sédimentaire intramontagnard et impacts des aménagements contemporains: L'exemple de la vallée de l'Arve (74), Houille Blanche, 3–4, 221–225, https://doi.org/10.1051/lhb/1989018, 1989.
Petrucci, O., Aceto, L., Bianchi, C., Bigot, V., Brázdil, R., Pereira, S., Kahraman, A., Kılıç, Ö., Kotroni, V., Llasat, M. C., Llasat-Botija, M., Papagiannaki, K., Pasqua, A. A., Řehoř, J., Rossello Geli, J., Salvati, P., Vinet, F., and Zêzere, J. L.: Flood Fatalities in Europe, 1980–2018: Variability, Features, and Lessons to Learn, Water, 11, 1682, https://doi.org/10.3390/w11081682, 2019.
Préfecture de la Haute-Savoie: Données communales: plans de prévention des risques naturels (PPRN), Les services de l'Etat en Haute-Savoie, http://www.haute-savoie.gouv.fr/Politiques-publiques/Environnement-risques-naturels-et-technologiques/Prevention-des-risques-naturels/Donnees-communales-plans-de-prevention-des-risques-naturels (last access: 8 March 2022), 2021.
Rannaud, M.: Histoire de Sixt, edited by: Abry, J., Annecy, France, 672 pp., 1916.
Rougier, H.: Les inondations du 24 juillet dans la haute vallée de l'Arve: faits et conséquences à tirer pour l'aménagement du territoire., Les pouvoirs publics face aux risques naturels dans l'histoire, MSH-Alpes, Grenoble, 51–64, ISBN 978-2-914242-08-0, 2002.
RTM and ONF: La catastrophe du Grand-Bornand, movie, 1990.
RTM and ONF: Base de Données RTM, https://rtm-onf.ign.fr/ (last access: 8 March 2022), 2012.
Ruin, I., Creutin, J.-D., Anquetin, S., and Lutoff, C.: Human exposure to flash floods – Relation between flood parameters and human vulnerability during a storm of September 2002 in Southern France, J. Hydrol., 361, 199–213, https://doi.org/10.1016/j.jhydrol.2008.07.044, 2008.
Schlögl, M., Fuchs, S., Scheidl, C., and Heiser, M.: Trends in torrential flooding in the Austrian Alps: A combination of climate change, exposure dynamics, and mitigation measures, Climate Risk Management, 32, 100294, https://doi.org/10.1016/j.crm.2021.100294, 2021.
SITG: GEOMOL-GEOPOTENTIELS, https://ge.ch/sitg/actualites/geomol-donnees-de-base-potentiels-unites-geologiques (last access: 8 March 2022), 2020.
Soanes, C. and Stevenson, A.: Catastrophe, Concise Oxford English Dictionary, 11 (revised), Oxford University Press, ISBN 9780199558452, 2009.
Terti, G., Ruin, I., Anquetin, S., and Gourley, J. J.: Dynamic vulnerability factors for impact-based flash flood prediction, Nat. Hazards, 79, 1481–1497, https://doi.org/10.1007/s11069-015-1910-8, 2015.
Thoumas, P.: Histoire des crues du Roubion (Drôme, France) depuis 1501 AD, une approche hydrologique des fluctuations climatiques sur cinq siècles, physio-geo, 14, 87–111, https://doi.org/10.4000/physio-geo.8984, 2019.
Viani, A., Condom, T., Sicart, J.-E., Rabatel, A., Gascoin, S., Ranzi, R., and Wimez, M.: Impact of the glacier retreat and snow melt on the seasonal cycle of streamflow of the Arve catchment since the 1960s (Northern French Alps), EGU General Assembly, Vienna, Austria, April 2018, 1, 13288 pp., https://meetingorganizer.copernicus.org/EGU2018/EGU2018-13288.pdf (last access: 9 March 2022), 2018.
Vincent, C., Descloitres, M., Garambois, S., Legchenko, A., Guyard, H., and Gilbert, A.: Detection of a subglacial lake in Glacier de Tête Rousse (Mont Blanc area, France), J. Glaciol., 58, 866–878, https://doi.org/10.3189/2012JoG11J179, 2012.
Wetter, O.: The potential of historical hydrology in Switzerland, Hydrol. Earth Syst. Sci., 21, 5781–5803, https://doi.org/10.5194/hess-21-5781-2017, 2017.
Wilhelm, B., Ballesteros Canovas, J. A., Macdonald, N., Toonen, W., Baker, V., Barriendos, M., Benito, G., Brauer, A., Corella, J. P., Denniston, R., Glaser, R., Ionita, M., Kahle, M., Liu, T., Luetscher, M., Macklin, M., Mudelsee, M., Munoz, S., Schulte, L., St. George, S., Stoffel, M., and Wetter, O.: Interpreting historical, botanical, and geological evidence to aid preparations for future floods, Wires Water, 6, e1318, https://doi.org/10.1002/wat2.1318, 2019.
Zgheib, T., Giacona, F., Granet-Abisset, A.-M., Morin, S., and Eckert, N.: One and a half century of avalanche risk to settlements in the upper Maurienne valley inferred from land cover and socio-environmental changes, Global Environ. Change, 65, 102149, https://doi.org/10.1016/j.gloenvcha.2020.102149, 2020.