the Creative Commons Attribution 4.0 License.
the Creative Commons Attribution 4.0 License.
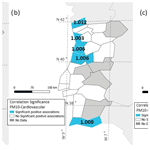
Impact of large wildfires on PM10 levels and human mortality in Portugal
Patricia Tarín-Carrasco
Sofia Augusto
Laura Palacios-Peña
Nuno Ratola
Pedro Jiménez-Guerrero
Uncontrolled wildfires have a substantial impact on the environment, the economy and local populations. According to the European Forest Fire Information System (EFFIS), between 2000 and 2013 wildfires burned up to 740 000 ha of land annually in the south of Europe, Portugal being the country with the highest percentage of burned area per square kilometre. However, there is still a lack of knowledge regarding the impacts of the wildfire-related pollutants on the mortality of the country's population. All wildfires occurring during the fire season (June–July–August–September) from 2001 and 2016 were identified, and those with a burned area above 1000 ha (large fires) were considered for the study. During the studied period (2001–2016), more than 2 million ha of forest (929 766 ha from June to September alone) were burned in mainland Portugal. Although large fires only represent less than 1 % of the number of total fires, in terms of burned area their contribution is 46 % (53 % from June to September). To assess the spatial impact of the wildfires, burned areas in each region of Portugal were correlated with PM10 concentrations measured at nearby background air quality monitoring stations. Associations between PM10 and all-cause (excluding injuries, poisoning and external causes) and cause-specific mortality (circulatory and respiratory) were studied for the affected populations using Poisson regression models. A significant positive correlation between burned area and PM10 was found in some regions of Portugal, as well as a significant association between PM10 concentrations and mortality, these being apparently related to large wildfires in some of the regions. The north, centre and inland of Portugal are the most affected areas. The high temperatures and long episodes of drought expected in the future will increase the probabilities of extreme events and therefore the occurrence of wildfires.
- Article
(2975 KB) - Full-text XML
-
Supplement
(100 KB) - BibTeX
- EndNote
Wildfires have a considerable impact on the environment and humans worldwide. Climate change has lately been identified as a very important variable in this matter (Gillett et al., 2004) since the future projections suggest an increase in the number of droughts, heat waves and dry spells (Turco et al., 2019). Global warming will produce changes in temperature and precipitation patterns leading to a higher prevalence and severity of wildfires (Settele et al., 2014; Bowman et al., 2017) and consequently impacting future air quality (Schär et al., 2004). In fact, this could not only extend the burned area in chronically impacted areas (Ciscar et al., 2014) but also affect new ones, like Sweden in the summer of 2018 (Lidskog et al., 2019). According to the 2016 European Forest Fire Information System (EFFIS) report (San-Miguel-Ayanz et al., 2017), the south of Europe (Portugal, Spain, France, Italy and Greece) is the area most affected by wildfires from 1980 until today, considering Europe, the Middle East and North Africa. In the last decades, Portugal was by far the country with the largest burned area, almost 50 %, between the southern European countries (Parente et al., 2018). Although there has been a slight decreasing trend in the burned area in this region since 2000 after an increasing period in the previous 20 years (European Environment Agency, https://www.eea.europa.eu/data-and-maps/indicators/forest-fire-danger-3/assessment, last access: 30 June 2020), recent extreme events like the 2017 fires in Portugal and the 2018 fires in Greece which resulted in a severe loss of human lives are confirming the worst-case projections.
Uncontrolled wildfires emit numerous pollutants derived from the incomplete combustion of biomass fuel which cause damage to human health, particularly the cardiovascular and respiratory systems (World Health Organization, 2010). Examples include particulate matter (PM), carbon monoxide, methane, nitrous oxide, nitrogen oxides, volatile organic compounds (VOCs) and other secondary pollutants (Cascio, 2018) that are released mainly into the atmosphere but can be transported to many other environmental compartments. Moreover, they can affect the physicochemical properties of the atmosphere, like, for instance, the interaction of PM with solar radiation which can prompt a modification of the temperature depending on the characteristics of the aerosol (Trentmann et al., 2005). Consequently, some of these chemicals are regulated by the European Directive 2008/50/EC of 21 May 2008 of the European Parliament and of the Council on Ambient Air Quality and Cleaner Air for Europe, which establishes threshold values for a safe air quality. But although wildfire emissions are a crucial parameter for the local air quality (Knorr et al., 2016), where in some cases there are already chronically exposed populations due to the frequency and dimension of the events, they are not contained by political borders and can also affect areas far from the ignition points due to the atmospheric transport of the pollutant plumes. A number of studies (e.g. Lin et al., 2012; Im et al., 2018; Liang et al., 2018; Augusto et al., 2020, among others) report the influence of natural and anthropogenic emissions on air quality composition across different countries, especially PM and tropospheric O3. For wildfires it is also important to take into account some factors which influence the plume dispersion, such as the duration and space evolution of the fire event and the meteorological conditions associated with it (Lazaridis et al., 2008). An increase in cardiovascular and respiratory morbidity and mortality are some of the impacts these contaminants can have on humans (Johnston et al., 2012; Tarín-Carrasco et al., 2019). For instance, there is strong evidence for the relationship between PM in general and mortality, especially from cardiovascular diseases, for both long-term and short-term exposure (Anderson et al., 2012). Although some studies corroborate the existence of a link between the exposure to wildfire-related air pollutants and hospital admissions, visits to emergency clinics or even respiratory morbidity (Liu et al., 2015; Reid et al., 2016), the impacts on human health are difficult to quantify and the real effects still poorly known.
Concerning PM, a recent study focusing on 10 southern European cities revealed that cardiovascular and respiratory mortality associated with PM10 (particles with an aerodynamic diameter below 10 µm) was higher on days affected by wildfires' smoke than in smoke-free days (Faustini et al., 2015). The authors also found that PM10 from forest fires increased mortality more than PM10 from other sources. So, the estimation of mortality due to exposure to wildfire-generated pollutants is key to manage health resources and the necessary public funds towards prevention and remediation in setting up appropriate policies and protocols (Rappold et al., 2012).
The two main factors to take into account for the wildfire's effects are the location and, most importantly, the size of the fire event (characterised by the respective burned area). When the wildfire occurs close to a large conurbation, the population exposed is higher. But as Analitis et al. (2012) showed in their study, small fires do not seem to have an effect on mortality, whereas medium and large episodes (with burned areas > 1000 ha) have a significant impact on human health, which increases with the size of the fire. Aiming to enhance the knowledge on the effects of wildfires on human health, this study describes the pattern of wildfires in Portugal for 16 years (2001–2016) and assesses the impact of those events on the country's population mortality during the fire season (June, July, August and September). In this work, the focus is placed on indirect effects of pollutants emitted by wildfires, namely assessing the influence of wildfire-generated PM10 on the Portuguese population mortality. The relationship between the burned area of large wildfires and PM10 and this same pollutant and mortality was studied. The Nomenclature of Territorial Units for Statistics (NUTS) level 3 (NUTS III) geographical division has been used to be able to compare the effects of the fires in different parts of the country. Finally, monthly deaths due to all-cause (excluding injuries, poisoning and external causes) and cause-specific mortality (cardiovascular and respiratory) for all ages for each NUTS III region have been studied. These causes have been selected due to their well-known connection with air pollution.
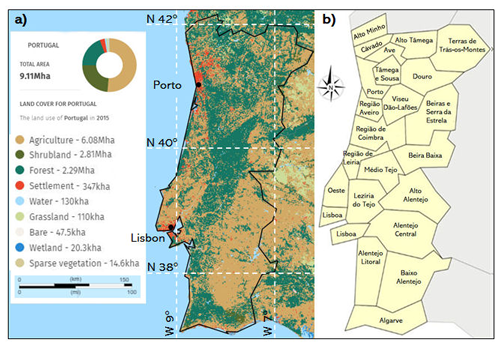
Figure 1(a) Land cover in mainland Portugal in 2015 (Global Forest Watch, https://www.globalforestwatch.org, last access: 10 October 2020; (b) mainland Portugal NUTS III regions.
In this study, the effects of short-term exposure to pollutants due to wildfires on human mortality were quantified. The forest fire pollutant emissions were estimated for the period 2001–2016 during the summer months (June–July–August–September) in mainland Portugal (23 NUTS III regions and more than 10 million people). In Portugal, large forest fires usually occur during the months of June, July, August and September, which correspond to the time of the year with the highest temperatures and driest conditions. By focusing our study only on these 4 months, we can have enough data to perform a valid statistical treatment, while avoiding a strong influence of PM10 from other sources in colder months (such as home heating or traffic). At the same time, we do not include in the analysis the deaths due to, for instance, cold and flu that could become confounding factors.
For the quantification, two steps were followed. First, an assessment of the incidence, patterns and variations of burned area on a large time frame and spatially integrated by NUTS III was done on the levels of air pollutants. PM10 and burned area were correlated through linear regression, while the mortality data and PM10 were correlated with Poisson regression. Data were processed and ordered by NUTS region and by month and year. Finally, the correlation between the pollutants emitted by forest fires, the wildfire-burned area and the different causes of mortality during the period 2001–2016 for the summer months were studied. The study is focused on PM since it is one of the main pollutants emitted by wildfires, which can increase PM concentrations by up to 50 % and more (Lazaridis et al., 2008). Moreover, there is a clear relation with several effects on human health (including mortality), in particular with respiratory and circulatory diseases (Kollanus et al., 2016; Reid et al., 2016; Liang et al., 2018). There was not enough PM2.5 data collected from the Portuguese air quality management network to establish a correlation (only 20 stations measure PM2.5 on the mainland). For all these reasons this study focuses on PM10.
2.1 Target area
At 89 015 km2 (9.11 Mha) mainland Portugal accounts for over 96 % of the country's area and hosts over 10 million inhabitants in the west Iberian Peninsula (southwestern Europe). With the largest urban areas along the west Atlantic coast, particularly around the capital (Lisbon) – more to the south – and the second largest city (Porto) in the north (see Fig. 1a), the country has most of its mountain ranges in the north, reaching 1993 in Serra da Estrela. Although showing a Mediterranean climate, this topographic display leads to various climate patterns throughout the country, with increasing temperature and decreasing rainfall from northwest to southeast (Moreira et al., 2011; Oliveira et al., 2017). In terms of land cover, Fig. 1a shows a predominance of agriculture in 2015 (over 50 % and mainly in the south), followed by forests and shrublands, which comprise 43 % of the territory (mainly in the north and southwest). This, combined with high temperatures in the summer months, represents a potential fire hazard, which unfortunately has been often proved true almost every summer for many years.
2.2 Datasets
2.2.1 NUTS III boundary data
The target domain was divided by NUTS (Nomenclature of Territorial Units for Statistics) level 3 (Fig. 1a) for Portugal's mainland. NUTS is a geocode standard developed by the European Union for referencing the subdivisions of countries for statistical purposes. The geocode is divided in three levels (I, II, III) which are established by each EU member country. NUTS III regions from mainland Portugal (in total, 23) at a 1:60 million scale were retrieved from the Eurostat web page (Eurostat, 2019) and treated with QGIS3 software.
Table 1Number of wildfires and burned area (BA) by year for the period 2001–2016 in mainland Portugal. From left to right: number of occurrences (occ.) when the burned area is larger than 1000 ha; sum of the burned area for fires larger than 1000 ha; total burned area caused by all fires; percentage of burned area caused by large fires; and index between burned area and the number of occurrences.
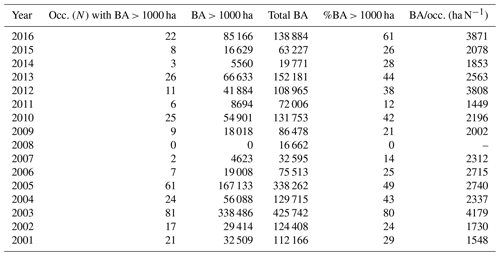
2.2.2 Wildfire data
The wildfire data, collected for the period from 2001 to 2016, were obtained from the Portuguese Institute for Nature Conservation and Forests (https://www.icnf.pt/, last access: 1 September 2019). For this study only forest fires were considered, and from them, only those with more than 1000 ha of total burned area (which we denominated large fires) were selected. In total, there were 323 events under that category (less than 1 % of the number of total fires), which were responsible for 46 % of the total burned area. Table 1 shows the yearly variability during the studied period in the number of occurrences, total burned area or the contribution of large fires to the burned area in mainland Portugal. Although other studies have shown a relationship with high temperatures and drought periods (Turco et al., 2019), the data in Table 1 suggest that it is not possible to perceive a yearly pattern of wildfires in the country. For instance, in 2008 no large fires occurred, whereas 2003 accounted for the highest number of occurrences (81), which were responsible for 80 % of the total burned area in that year. But the latter contribution was as low as 12 % in 2011 and had a mean percentage for the whole period of 34 %.
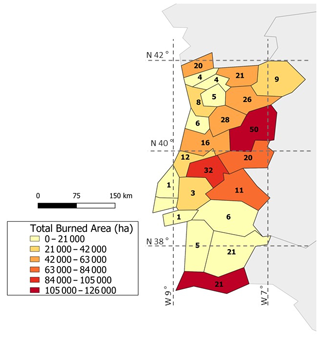
Figure 2Total burned area per NUTS III region from June to September in the period 2001–2016. The numbers inside each NUTS III region represent the respective number of large fires (> 1000 ha) in the same period.
Considering only June, July, August and September 2001–2016 (the months with the highest temperatures and drier conditions when more than 86 % of the total fires and 311 of the 323 large fires – 96 % – occurred), these data were divided by month and year, and the respective monthly and yearly sums were considered for each NUTS III level region. All NUTS III regions had at least one large fire during the study period. In terms of burned area 929 766 ha of forest were lost in mainland Portugal from June to September (2001 to 2016), with about 53 % due to large fires (> 1000 ha). Figure 2 represents the number of large fires and the burned area they were responsible for by NUTS III region.
The north and centre of Portugal present the most extensive forest cover in the country (Nunes et al., 2019), particularly abundant in pine and eucalyptus trees, two highly combustible species that have been associated with extreme wildfire events (Maia et al., 2014). Consequently, both areas show the highest number of large fires and respective burned area (Beiras e Serra da Estrela and Médio Tejo being the most affected NUTS III regions) but also with Alto Alentejo and Algarve (more to the south; see Fig. 1) among the NUTS III regions with a higher incidence. Additionally, dense Mediterranean forests over hard-to-reach mountains can also be found in these areas, which when combined enhance the difficulty of the firefighting efforts. Algarve, despite being located on the south coast, also has some mountains with forests surrounded by a considerably dry and arid terrain, especially in the summer (Nunes et al., 2019), leading to a burned area of 112 764 ha, the second highest at the NUTS III level. Beiras e Serra da Estrela is the region which presented the largest burned area in the summer months from 2001 to 2016 (almost 117 000 ha) and the highest number of large fires (50). On the other hand, Oeste and A.M. Lisbon (A.M. signifies metropolitan area; mainly non-forested areas) are the NUTS III regions with a smaller number of large fires – only one during the target time frame. More detailed information can be found in Table SM1 in the Supplement.
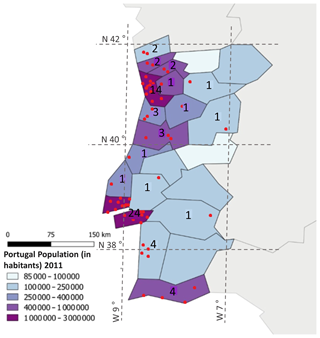
Figure 3Population of each NUTS III region according to the 2011 Census (https://www.ine.pt, last access: 16 October 2019) and respective number of monitoring stations for PM10. Red dots indicate the location of the air quality monitoring stations used in this study.
2.2.3 Pollution data
The information available on the levels of pollutants was obtained from the Portuguese Environment Agency air quality network (https://qualar.apambiente.pt/qualar/index.php, last access: 10 October 2020), established to monitor the concentrations of pollutants according to the European legislation requirements (European Directive 2008/50/EC of 21 May 2008). The locations of the air quality stations are irregularly scattered throughout the country, with a stronger presence in the most populated areas (Fig. 3). The isolation of pollutant emissions due to burned biomass is quite complicated as it depends on parameters such as vegetation type, the weather conditions at the moment the fire is taking place and the contribution of other sources, among others.
Considering all the pollutants measured at the background stations, PM10 was the one with a potentially higher link to forest fires. Although some stations also measured PM2.5, the coverage in this case was insufficient to draw any significant correlations. The main anthropogenic sources of PM10 include road traffic, industrial activities and home heating. In this study, to minimise the influence of non-wildfire causes for the PM10 concentrations, we selected only background stations (encompassing urban and semi-urban ones, which are located within urban areas but with minimum influence of road traffic, and rural stations). Therefore, urban stations with road traffic influence and stations close to industrial complexes were not selected. The influence of home heating was already minimised by selecting the summer period as our target time frame.
As done before for the wildfire data, here the time range considered was also from 2001 to 2016 and only the months of June to September, with monthly means used for the correlations. Concentrations of PM10 were obtained for mainland Portugal at all types of background stations (a total of 91 which cover 17 NUTS III regions, as shown in Fig. 3). Given the uneven coverage of the target domain, most stations are located in the metropolitan areas of Oporto (14 stations) and Lisbon (24 stations) and in the rest of the coastal areas, where the higher population (NUTS III regions commonly above 250 000 inhabitants; Fig. 3) demands a tighter control of the air quality, but where, in turn, not a lot of large wildfires occur due to the urbanised land use. For the NUTS III regions with more than one station a mean between all the PM10 concentrations in each NUTS region was calculated.
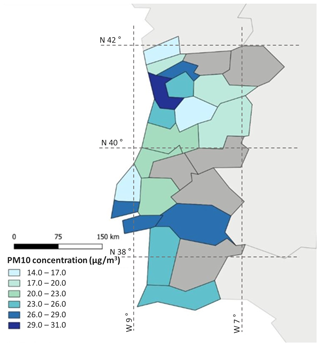
Figure 4Mean concentration of PM10 by NUTS III region from June to September in the period 2001–2016 in mainland Portugal.
Figure 4 shows the mean concentration of PM10 by NUTS III region from June to September in the period 2001–2016 at the stations available in mainland Portugal. The highest mean concentrations of PM10 during the period 2001–2016 (June to September only) were observed in Oporto, with 31 µg m−3; followed by Lisbon, Alentejo Central and Ave, with levels ranging from 26 to 29 µg m−3 (Fig. 4). Conversely, the NUTS III regions which present the lowest mean values, between 14 and 17 µg m−3, are Oeste, Alto-Minho and Viseu Dão-Lafões. Despite these values, no NUTS region in Portugal exceeds the threshold value of PM10 (40 ) established by the European Directive 2008/50/EC.
2.2.4 Mortality data
Mortality data covering the period from 2001 to 2016 were obtained from Statistics Portugal (https://www.ine.pt, last access: 16 October 2019). Monthly death counts due to all-cause (International Classification of Diseases ICD-10, codes A00–R99), excluding injuries, poisoning and external causes, and cause-specific mortality – cardiovascular (codes I00-I99) and respiratory (J00–J99) – were collected for each NUTS III region of Portugal, comprising residents of all ages. These mortality causes were selected since they have been reported previously in the literature as being important in their connection with air pollution (Hoek et al., 2013; Liu et al., 2015; Kollanus et al., 2016; Münzel et al., 2018), in particular with particulate matter (PM). Other relevant mortality causes, such as chronic obstructive pulmonary disease (COPD, codes J40–J45) and asthma (ICD-10, code J47), were also considered. However, since in many months and in the NUTS III target time period there were no deaths for COPD and asthma, it was not possible to obtain a data series large enough to correlate with PM10 and wildfire series.
2.3 Statistical analysis
2.3.1 Correlations between PM10 and burned area
The correlations between PM10 and the total burned area by large fires per month for each NUTS III region were estimated using Pearson correlations coefficients (Pearson and Galton, 1895). The Pearson approach, used to correlate two continuous variables having a normal distribution, is widely found in studies of air pollution (e.g. Pallarés et al., 2019; Rahman et al., 2019; Rovira et al., 2020, among many others). Results were considered statistically significant if the p value was p<0.05. Correlations were performed using the detrended data series of burned area and PM10 in order to remove the strong seasonal cycle of these variables and avoid spurious correlations. The detrending method follows Tarín-Carrasco et al. (2019), using the first-time difference time series.
2.3.2 Associations between burned area, PM10 and mortality
The associations of monthly average PM10 levels, and the occurrence of large wildfires (burned area > 1000 ha), with mean monthly mortalities (all-cause and respiratory and cardiovascular causes) were studied for the months of June, July, August and September for the period between 2001 and 2016. The estimates of the effects were obtained for each NUTS III region using Poisson regression models (Faustini et al., 2015; Islam and Chowdhury, 2017). Poisson coefficients can correlate a count variable (such as the number of deaths) with a continuous variable. The results were expressed as the relative risk (RR) of all-cause, cardiovascular and respiratory mortalities with a 95 % confidence interval (95 % CI). All regression models were performed using IBM SPSS Statistics 25.0 software.
3.1 Relationship between burned area and particulate matter
For the correlation between the burned area from large fires and PM10, a significant positive correlation was found for 7 (out of 13 with available data) of the NUTS III regions studied, represented by the dotted areas in the map of Fig. 5. For Oeste, Região de Leiria, Beira Baixa, Médio Tejo, Cávado, Ave, Terras de Trás-os-Montes, Alto Tâmega and the four Alentejo NUTS (Alto Alentejo, Alentejo Central, Alentejo Litoral and Baixo Alentejo), there were not enough pollutant and/or burned area data to establish statistical relationships. The correlations are strongest for Cávado, Ave, Tâmega e Sousa, Região de Aveiro and Viseu Dão-Lafões, with correlation coefficients above 0.75 at a confidence level of 0.95, followed by Alto Tâmega and Beiras e Serra da Estrela between 0.5 and 0.74. As expected, all these areas are in the north and centre of mainland Portugal, in line with the denser forest cover.
Finally, in Alto Minho, A.M. Porto, Douro, Região de Coimbra, Região de Leiria and Algarve no significant correlations were found. The limited number of stations in those areas (which means less data to correlate), their location (closer or farther from the large wildfire spots) and uneven distribution, as well as the contribution of other sources to the PM10 levels, can be some of the explanations. The location of the air monitoring stations may play a key role in these correlations, especially when they are scarcer, but for these NUTS regions this is a good indication of where the influence of wildfires on the emissions of PM10 is likely to be stronger. In fact, some authors have reported a contribution of wood burning to the PM10 load even in urban environments, where the presence of other PM sources tends to be higher (Fuller et al., 2014; Perrino et al., 2019).
3.2 Impact of wildfires on mortality
3.2.1 Mortality overview
The mortality counts for the period 2001–2016 (for the months of June to September, 64 months) in mainland Portugal are presented in Table 2 for each NUTS III region and all-cause and cardiovascular- and respiratory-related deaths. Results show that almost 30 % of all-cause and cardiovascular mortality occur during the extended summer (June, July, August and September), as do 26 % of the respiratory mortality.
Algarve, Alto Minho, Alto Alentejo and A.M. Lisbon are the NUTS regions with the higher percentage of all-cause mortality for the studied months, but the NUTS regions with more per capita incidences are Beira Baixa, Alto Alentejo, Baixo Alentejo and Beiras e Serra da Estrela, areas with lower population density and with mean higher age than the rest of the country.
With respect to cardiovascular mortality, the NUTS III regions which present a high incidence are Algarve, Terras de Trás-os-Montes and Beira Baixa, with the latter, Baixo Alentejo and Alto Alentejo, having a higher percentage of population affected.
Finally, the results obtained for respiratory mortality show that Algarve, Lezíria do Tejo and Alentejo Litoral are the NUTS III regions which top the ranking in the summer months, whereas Alto Alentejo is the region with most population affected. Alentejo and Algarve suffer from high temperatures in the summer, which may also be an indicator that contributes to a higher mortality in general (Basu and Samet, 2002) but also due to cardiovascular and respiratory diseases (Pinheiro et al., 2014). In addition, the aforementioned regions suffer from a considerable afflux of tourists that increase their population in the same period, particularly in Algarve. In Alentejo, the combination of high temperatures with an aged population and less health care resources available may be the reason why more of the population is affected by mortality (Chen et al., 2019). In fact, this is a tendency that has become stronger since the beginning of the 21st century, as the percentage of the population over 65 years of age changed in Alentejo from 22.5 % in 2001 to 25.4 % in 2018, higher than the percentages in the whole of Portugal (from 16.4 % in 2001 to 21.7 % in 2018) (as derived from Pordata, https://www.pordata.pt, last access: 31 March 2020).
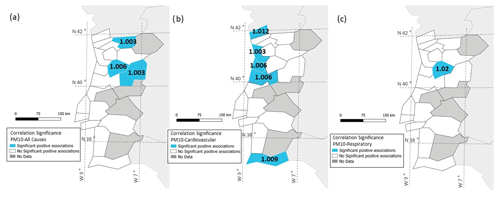
Figure 6Relative risks (RRs, numbers) obtained from Poisson regression for PM10 and (a) all-cause mortality, (b) cardiovascular mortality and (c) respiratory mortality from June to September in the period 2001–2016 (light blue NUTS III regions indicate a significant result, p<0.05; white NUTS III regions present no significant results for the variables studied; grey NUTS III regions indicate that no data were available for correlations). Only significant RRs are shown.
3.2.2 Associations between mortality and PM10
Wildfires are an important source of particulate matter, and the associations between mortality, PM10 and the occurrence of large wildfires are assessed in this section. As shown in Fig. 6a, three NUTS III regions (Alto Tâmega, Beiras e Serra da Estrela and Viseu Dão-Lafões) present associations between PM10 and all-cause mortality during the studied period. None showed a direct significant association with the occurrence of large fires likely due to the fact that their contribution to the total burned area in each year from 2001 to 2016 (Table 1) was highly variable (from 12.1 % in 2011 to 79.5 % in 2003). However, the wildfire origin of PM10 is corroborated by the positive significant correlations obtained for these three NUTS regions between PM10 and burned area (Fig. 5), with Viseu Dão-Lafões displaying the highest correlations.
Beiras e Serra da Estrela is the NUTS region most affected by large wildfires during the studied period, both in number (50) and respective burned area (> 100 000 ha), and Viseu Dão-Lafões is the third in occurrences (28) corresponding to over 58 000 ha burned. This involves high levels of PM10 in a short period of time, which might result in damage to human health, particularly in an aged population (e.g. for Beiras e Serra da Estrela, 23.8 % and 28.7 % over-65-year-olds in 2001 and 2018, respectively; PORDATA, https://www.pordata.pt, last access: 31 March 2020).
In terms of types of diseases, for cardiovascular mortality five NUTS regions presented associations with PM10: Alto Minho, A.M. Porto, Região de Aveiro, Região de Coimbra and Algarve (Fig. 6b). Again, no direct significant associations were obtained with the occurrence of large fires. From these five NUTS, only Região de Aveiro showed a significant correlation between PM10 and burned area, revealing the impact of wildfires in the origin of the PM10. For respiratory mortality, only Viseu Dão-Lafões presents associations with PM10, for which a strong correlation between PM10 and burned area was found, suggesting again the impact of wildfires on the presence of PM10 (Fig. 6c).
During the studied period (2001–2016), large fires were responsible for 46 % of the more than 2 million ha of forest burned in mainland Portugal. The areas most affected by number and size of wildfires are the north, centre and inland of the country. Wildfires do not follow a pattern in number of the occurrences or size during the years studied. This evidence was found despite the difficulties that the uneven scattering of the air quality monitoring stations analysing PM10 in Portugal posed. In fact, the areas where wildfires are usually more frequent (inland) are far from the urban centres (mainly along the coast) and thus not abundant in available air quality data due to the shortage (or even lack in some NUTS III regions) of monitoring stations. These regions also have an aged population, poorer economy and less health care resources, which can lead to an increase in the mortality rates in general. The socio-economic status of the population affected and the health care facilities and measures existing in the communities have to be taken into account (Oliveira et al., 2017), adding to the countless parameters that may affect these estimations which contribute to considerable gaps identified in this type of studies (Black et al., 2017). Unfortunately, the scarce data available and the lack of accuracy in the existing data prevented us from estimating and/or including a correction regarding their influence.
Nevertheless, it was possible to find relationships between very relevant parameters. The significant positive correlation between PM10 and burned area found for 7 of the 13 NUTS III regions with available data is a good indication of where the influence of wildfires on the emission of PM10 is likely to be stronger. Although the location of the air quality monitoring stations may influence these correlations, especially when they are scarcer, for these NUTS regions it reveals the influence of wildfires on the local levels of PM10.
Large wildfires tend to be active for several days, releasing high amounts of pollutants to the atmosphere. In Portugal, such as in other Mediterranean countries, most of the wildfires are potentiated by strong winds which may spread the fire smoke over large distances (Turco et al., 2019; Augusto et al., 2020). Thus, air quality monitoring stations located far from the ignition sites can still detect increases in the concentrations of, for instance, PM10. Augusto et al. (2020), while studying the impact of the uncontrolled wildfires of October 2017 in Portugal on mortality, found that the PM10 and PM2.5 emitted reached the United Kingdom, as well as other northern European countries. Likewise, in Finland, where ambient PM levels are relatively low compared to other countries in Europe, most of the strongest PM pollution episodes are typically related to emissions from wildfires in eastern European countries (Russia, Belarus, Ukraine, Estonia, Latvia and Lithuania) at a distance of hundreds to thousands of kilometres from southern Finland (Niemi et al., 2009; Kollanus et al., 2016).
The negative impact particulate matter can bring to human health is well established and can be translated into several types of diseases (Kim et al., 2015). There is evidence that it can enter the human body, arrive in the bloodstream, and damage some organs or even cause death due to cardiovascular afflictions like stroke or heart attack, among others, representing a clear hazard to public health (Brook et al., 2010; Hamanaka and Mutlu, 2018). Particulate matter also can damage the human respiratory system. The risk depends on the size of the particle, which if very small can even reach the alveolus (Neuberger et al., 2004; Jo et al., 2017).
In our study, the NUTS III regions where PM10 concentrations were found to be correlated with the burned area from large fires (Cávado, Ave, Tâmega e Sousa, Região de Aveiro, Viseu Dão-Lafões, Alto Tâmega and Beiras e Serra da Estrela) are indeed the ones where it would be expected to find the strongest influence of the wildfire-originated PM10 on population mortality. Although not for all, indeed associations between all-cause mortality and PM10 were found for three of these NUTS regions (Alto Tâmega, Viseu Dão-Lafões and Beiras e Serra da Estrela), with RRs varying from 1.003 to 1.006; between cardiovascular mortality and PM10 for one (Região de Aveiro) with an RR of 1.006, and between respiratory mortality and PM10 also for one (Viseu Dão-Lafões) with an RR of 1.020. Associations between cardiovascular mortality and PM10 were found for four NUTS regions (Alto Minho, A.M. Porto, Coimbra and Algarve) where there was no significant correlation of PM10 with burned area. All these are located on the coast, where the population density in Portugal is clearly predominant (particularly in A.M. Porto), as well as considerable industrial presence. In these regions, cardiovascular disease may have many other sources, some of them derived from a more sedentary and stressed lifestyle.
The mortality increase associated with PM10 is consistent with the estimates reported in other European studies, such as APHEA2 (Katsouyanni et al., 2001), APHENA (Samoli et al., 2008), EpiAir (Faustini et al., 2011) and MED-PARTICLES (Faustini et al., 2015), which also reported higher PM10 effects on all-cause, cardiovascular and respiratory mortalities.
However, some studies present uneven conclusions. Johnston et al. (2011) reported the highest effects on cardiovascular mortality, but Morgan et al. (2010) did not find any consistent effect with cardiovascular deaths in Australia, and Analitis et al. (2012) registered the highest effects on respiratory mortality in Greece. This high variability may be related to several factors, notably (i) different PM composition or varying gaseous emissions (CO, VOCs, NOx or SO2) from wildfires, which may have different degrees of toxicity on cardiovascular and respiratory systems; or (ii) increasing temperature during wildfires, which is known to enhance the effects of PM on more susceptible individuals (e.g. cardiac patients) (Qian et al., 2008). Therefore, the effects we found on all-cause, cardiovascular and respiratory mortalities during the wildfire seasons may be due to different PM compositions or increasing temperature.
Region-specific associations between PM10 concentrations and mortality were also observed. These may have been influenced by the factors described above (different PM composition and increasing temperature) but also by the magnitude and duration of the exposure to PM from a given fire, the underlying health status of the population and the size of the population. The age of the exposed individuals can also be important. In some studies, larger effect estimates in groups of those 65 years and older have been reported. Analitis et al. (2012) mentioned that the effect of respiratory mortality in Greece was higher in adults of ages 75 and above during large fires, whereas Haikerwal et al. (2015) observed an increase in risk of cardiac arrest, especially in older adults in Australia, although not all resulted in death. In Brazil, Nunes et al. (2013) reported that older adults had the strongest association between exposure to biomass burning and circulatory disease mortality. In Portugal, the regions traditionally impacted by wildfires coincide with a larger percentage of an aged population, which can help explain the obtained associations.
In our analysis, to study the relationship between the burned area and PM10 concentrations, averaged monthly data were used as the minimum temporal scale available for the burned area was 1 month. Other studies relating wildfire-originated PM and mortality are usually based on daily PM concentrations and daily death counts since they do not account for the burned area as a measure of the wildfire size. Therefore, the monthly approach obviously reduced the amount of data available and the possibility of finding more significant correlations, which may have diluted the effects of some wildfires on the population mortality. Moreover, some health effects may not have been detected because wildfires are episodic and local events. Nevertheless, the results provide an overall context, highlighting the strongest associations between wildfire-generated PM10 and all-cause, cardiovascular and respiratory mortalities. Being able to achieve them with this uneven distribution of available data is an indication that the approach can be very useful to at least uncover tendencies and, in regions with stronger monitoring capabilities and coverage, a way to find stronger and more accurate correlations. This will help legislators and other government bodies to propose ways to protect the population chronically exposed to wildfires or more susceptible to acute reactions to wildfire smoke.
Portugal is a country that suffers constantly from serious wildfire incidents, which are bound to pose a risk not only to chronically affected populations but also from acute impacts of the pollutants released in such events. In this work, analysing the summer months (June to September) on a lengthy time frame (2001–2016), it was possible to find relevant associations between PM10 (associated with large wildfires) and mortality in some NUTS III regions of mainland Portugal (mainly inland and in the north), as well as a significant correlation between burned area and PM10.
In particular, it was found that large fires (in this study considered above 1000 ha of burned area) have an impact on the health of the population in some areas due to the emission of particulate matter. The lack of data or possible confounding factors likely prevented a higher number of NUTS III regions with significant correlations. Moreover, in such severe events, the population exposed to a high concentration of pollutants in a short period of time should be considered as a risk modifier of the impacts of air pollution exposure (Desikan, 2017; Rappold et al., 2017).
These episodes occurred during the summer months (June–July–August–September) when high temperatures and long episodes of drought increase the probabilities of one of these extreme events. In a future ruled by climate changes, the high temperatures and long periods of drought that usually fuel big fires are expected to increase, thus paving the way for more extreme and intense events to occur even outside the typically affected regions. Thus, more population will be exposed more frequently to high pollutant levels, affecting their general health and increasing chronic diseases and mortality. Hence, restrictive policies and protocols to improve the effectiveness of preventive and mitigation actions must be enforced to face this environmental and societal issue.
Data are publicly available through the websites mentioned in the text:
-
EFFIS (European Forest Fire Information System), Data and Services, 2019. Available at https://effis.jrc.ec.europa.eu/applications/data-and-services/ (last access: 11 October 2019).
-
ICNF (Instituto da Conservação da Natureza e das Florestas), 2019. Available at https://www.icnf.pt/ (last accessed: 1 September 2019).
-
INE (Instituto Nacional de Estatística), Statistics Portugal – Web Portal, 2019. Available at https://www.ine.pt/xportal/xmain?xpid=INE&xpgid=ine_indicadores&contecto=pi&indOcorrCod=0008273&selTab=tab0 (last access: 16 October 2019).
-
GWF (Global Forest Watch), 2020. Available at https://www.globalforestwatch.org/map/?gfwfires=true (last access: 10 October 2020).
-
PORDATA (Base de Dados Portugal Contemporâneo), População residente: total e por grandes grupos etários (in Portuguese), 2019. Available at https://www.pordata.pt (last access: 31 March 2020).
All the compiled data are available upon contacting the corresponding author (pedro.jimenezguerrero@um.es).
The supplement related to this article is available online at: https://doi.org/10.5194/nhess-21-2867-2021-supplement.
PTC wrote the manuscript, with contributions from SA and NR. The manuscript was finally revised by PJG. PTC and SA designed the experiments and led the statistical analysis, with the support of LPP, NR and PJG.
The authors declare that they have no conflict of interest.
Publisher's note: Copernicus Publications remains neutral with regard to jurisdictional claims in published maps and institutional affiliations.
This article is part of the special issue “The role of fire in the Earth system: understanding interactions with the land, atmosphere, and society (ESD/ACP/BG/GMD/NHESS inter-journal SI)”. It is a result of the EGU General Assembly 2020, 3–8 May 2020.
The authors are thankful to the G-MAR research group at the University of Murcia for the fruitful scientific discussions.
This work was financially supported by the European Regional Development Fund–Fondo Europeo de Desarrollo Regional (ERDF-FEDER), Spanish Ministry of Economy and Competitiveness/Agencia Estatal de Investigación (grant no. CGL2017-87921-R (ACEX project)) and Project UIDB/00511/2020 of LEPABE (Portuguese national funds through FCT/MCTES PIDDAC). Sofia Augusto was supported by the Portuguese Foundation for Science and Technology (grant no. SFRH/BPD/109382/2015).
This paper was edited by Sander Veraverbeke and reviewed by two anonymous referees.
Analitis, A., Georgiadis, I., and Katsouyanni, K.: Forest fires are associated with elevated mortality in a dense urban setting, Occup. Environ. Med., 69, 158–162, https://doi.org/10.1136/oem.2010.064238, 2012. a, b, c
Anderson, J. O., Thundiyil, J. G., and Stolbach, A.: Clearing the Air: A Review of the Effects of Particulate Matter Air Pollution on Human Health, Journal of Medical Toxicology, 8, 166–175, https://doi.org/10.1007/s13181-011-0203-1, 2012. a
Augusto, S., Ratola, N., Tarín-Carrasco, P., Jiménez-Guerrero, P., Turco, M., Schuhmacher, M., Costa, S., Teixeira, J., and Costa, C.: Population exposure to particulate-matter and related mortality due to the Portuguese wildfires in October 2017 driven by storm Ophelia, Environ. Int., 144, 106056, https://doi.org/10.1016/j.envint.2020.106056, 2020. a, b, c
Basu, R. and Samet, J. M.: Relation between Elevated Ambient Temperature and Mortality: A Review of the Epidemiologic Evidence, Epidemiol. Rev., 24, 190–202, https://doi.org/10.1093/epirev/mxf007, 2002. a
Black, C., Tesfaigzi, Y., Bassein, J. A., and Miller, L. A.: Wildfire smoke exposure and human health: Significant gaps in research for a growing public health issue, Environ. Toxicol. Phar., 55, 186–195, https://doi.org/10.1016/j.etap.2017.08.022, 2017. a
Bowman, D. M. J. S., Williamson, G. J., Abatzoglou, J. T., Kolden, C. A., Cochrane, M. A., and Smith, A. M. S.: Human exposure and sensitivity to globally extreme wildfire events, Nature Ecology & Evolution, 1, 58, https://doi.org/10.1038/s41559-016-0058, 2017. a
Brook, R. D., Rajagopalan, S., Pope, C. A., Brook, J. R., Bhatnagar, A., Diez-Roux, A. V., Holguin, F., Hong, Y., Luepker, R. V., Mittleman, M. A., Peters, A., Siscovick, D., Smith, S. C., Whitsel, L., and Kaufman, J. D.: Particulate Matter Air Pollution and Cardiovascular Disease: an update to the scientific statement from the American Heart Association, Circulation, 121, 2331–2378, https://doi.org/10.1161/CIR.0b013e3181dbece1, 2010. a
Cascio, W. E.: Wildland fire smoke and human health, Sci. Total Environ., 624, 586–595, https://doi.org/10.1016/j.scitotenv.2017.12.086, 2018. a
Chen, C.-H., Wu, C.-D., Chiang, H.-C., Chu, D., Lee, K.-Y., Lin, W.-Y., Yeh, J.-I., Tsai, K.-W., and Guo, Y.-L. L.: The effects of fine and coarse particulate matter on lung function among the elderly, Sci. Rep.-UK, 9, 14790, https://doi.org/10.1038/s41598-019-51307-5, 2019. a
Ciscar, J. C., Feyen, L., Soria, A., Lavalle, C., Raes, F., Perry, M., Nemry, F., Demirel, H., Rózsai, M., Dosio, A., Donatelli, M., Srivastava, A., Fumagalli, D., Niemeyer, S., Shrestha, S., Ciaian, P., Himics, M., Van Doorslaer, B., Barrios, S., Ibáñez, J., Forzieri, G., Rojas, R., Bianchi, A., Dowling, P., Camia, A., Liberta, G., San-Miguel-Ayanz, J., De Rigo, D., Caudullo, G., Barredo, J., Paci, D., Pycroft, J., Saveyn, B., Van Regemorter, D., Revesz, T., Vandyck, T., Vrontisi, Z., Baranzelli, C., Vandecasteele, I., Batista, F., and Ibarreta, D.: Climate impacts in Europe-The JRC PESETA II project, in: JRC Scientific and Policy Reports EUR 26586EN, vol. 26586, p. 155, Joint Research Center, Publications Office of the European Union, Luxembourg, 2014. a
Desikan, A.: Outdoor air pollution as a possible modifiable risk factor to reduce mortality in post-stroke population, Neural Regen. Res., 12, 351–353, https://doi.org/10.4103/1673-5374.202917, 2017. a
Eurostat: NUTS 2016, version 14/03/2019, 1:60 million scale, available at: https://ec.europa.eu/eurostat/web/gisco/geodata/reference-data/administrative-units-statistical-units/nuts (last access: 19 June 2021), 2019. a
Faustini, A., Stafoggia, M., Berti, G., Bisanti, L., Chiusolo, M., Cernigliaro, A., Mallone, S., Primerano, R., Scarnato, C., Simonato, L., Vigotti, M., and Forastiere, F.: The relationship between ambient particulate matter and respiratory mortality: a multi-city study in Italy, Eur. Respir. J., 38, 538–547, https://doi.org/10.1183/09031936.00093710, 2011. a
Faustini, A., Alessandrini, E. R., Pey, J., Perez, N., Samoli, E., Querol, X., Cadum, E., Perrino, C., Ostro, B., Ranzi, A., Sunyer, J., Stafoggia, M., and Forastiere, F.: Short-term effects of particulate matter on mortality during forest fires in Southern Europe: results of the MED-PARTICLES Project, Occup. Environ. Med., 72, 323–329, https://doi.org/10.1136/oemed-2014-102459, 2015. a, b, c
Fuller, G. W., Tremper, A. H., Baker, T. D., Yttri, K. E., and Butterfield, D.: Contribution of wood burning to PM10 in London, Atmos. Environ., 87, 87–94, https://doi.org/10.1016/j.atmosenv.2013.12.037, 2014. a
Gillett, N. P., Weaver, A. J., Zwiers, F. W., and Flannigan, M. D.: Detecting the effect of climate change on Canadian forest fires, Geophys. Res. Lett., 31, L18211, https://doi.org/10.1029/2004GL020876, 2004. a
Haikerwal, A., Akram, M., Monaco, A. D., Smith, K., Sim, M. R., Meyer, M., Tonkin, A. M., Abramson, M. J., and Dennekamp, M.: Impact of Fine Particulate Matter (PM2.5) Exposure During Wildfires on Cardiovascular Health Outcomes, J. Am. Heart Assoc., 4, e001653, https://doi.org/10.1161/JAHA.114.001653, 2015. a
Hamanaka, R. B. and Mutlu, G. M.: Particulate Matter Air Pollution: Effects on the Cardiovascular System, Front. Endocrinol., 9, 680, https://doi.org/10.3389/fendo.2018.00680, 2018. a
Hoek, G., Krishnan, R. M., Beelen, R., Peters, A., Ostro, B., Brunekreef, B., and Kaufman, J. D.: Long-term air pollution exposure and cardio-respiratory mortality: A review, Environ. Health-Glob., 12, 43, https://doi.org/10.1186/1476-069X-12-43, 2013. a
Im, U., Brandt, J., Geels, C., Hansen, K. M., Christensen, J. H., Andersen, M. S., Solazzo, E., Kioutsioukis, I., Alyuz, U., Balzarini, A., Baro, R., Bellasio, R., Bianconi, R., Bieser, J., Colette, A., Curci, G., Farrow, A., Flemming, J., Fraser, A., Jimenez-Guerrero, P., Kitwiroon, N., Liang, C.-K., Nopmongcol, U., Pirovano, G., Pozzoli, L., Prank, M., Rose, R., Sokhi, R., Tuccella, P., Unal, A., Vivanco, M. G., West, J., Yarwood, G., Hogrefe, C., and Galmarini, S.: Assessment and economic valuation of air pollution impacts on human health over Europe and the United States as calculated by a multi-model ensemble in the framework of AQMEII3, Atmos. Chem. Phys., 18, 5967–5989, https://doi.org/10.5194/acp-18-5967-2018, 2018. a
Islam, M. A. and Chowdhury, R. I.: A generalized right truncated bivariate Poisson regression model with applications to health data, PLOS One, 12, 1–13, https://doi.org/10.1371/journal.pone.0178153, 2017. a
Jo, E.-J., Lee, W.-S., Jo, H.-Y., Kim, C.-H., Eom, J.-S., Mok, J.-H., Kim, M.-H., Lee, K., Kim, K.-U., Lee, M.-K., and Park, H.-K.: Effects of particulate matter on respiratory disease and the impact of meteorological factors in Busan, Korea, Resp. Med., 124, 79–87, https://doi.org/10.1016/j.rmed.2017.02.010, 2017. a
Johnston, F., Hanigan, I., Henderson, S., Morgan, G., and Bowman, D.: Extreme air pollution events from bushfires and dust storms and their association with mortality in Sydney, Australia 1994–2007, Environ. Res., 111, 811–816, https://doi.org/10.1016/j.envres.2011.05.007, 2011. a
Johnston, F. H., Henderson, S. B., Chen, Y., Randerson, J. T., Marlier, M., DeFries, R. S., Kinney, P., Bowman, D. M., and Brauer, M.: Estimated Global Mortality Attributable to Smoke from Landscape Fires, Environ. Health Persp., 120, 695–701, https://doi.org/10.1289/ehp.1104422, 2012. a
Katsouyanni, K., Touloumi, G., Samoli, E., Gryparis, A., Tertre, A. L., Monopolis, Y., Rossi, G., Zmirou, D., Ballester, F., Boumghar, A., Anderson, H. R., Wojtyniak, B., Paldy, A., Braunstein, R., Pekkanen, J., Schindler, C., and Schwartz, J.: Confounding and Effect Modification in the Short-Term Effects of Ambient Particles on Total Mortality: Results from 29 European Cities within the APHEA2 Project, Epidemiology, 12, 521–531, 2001. a
Kim, K.-H., Kabir, E., and Kabir, S.: A review on the human health impact of airborne particulate matter, Environ. Int., 74, 136–143, https://doi.org/10.1016/j.envint.2014.10.005, 2015. a
Knorr, W., Dentener, F., Hantson, S., Jiang, L., Klimont, Z., and Arneth, A.: Air quality impacts of European wildfire emissions in a changing climate, Atmos. Chem. Phys., 16, 5685–5703, https://doi.org/10.5194/acp-16-5685-2016, 2016. a
Kollanus, V., Tiittanen, P., Niemi, J. V., and Lanki, T.: Effects of long-range transported air pollution from vegetation fires on daily mortality and hospital admissions in the Helsinki metropolitan area, Finland, Environ. Res., 151, 351–358, https://doi.org/10.1016/j.envres.2016.08.003, 2016. a, b, c
Lazaridis, M., Latos, M., Aleksandropoulou, V., Hov, Ø., Papayannis, A., and Tørseth, K.: Contribution of forest fire emissions to atmospheric pollution in Greece, Air Qual. Atmos. Hlth., 1, 143–158, https://doi.org/10.1007/s11869-008-0020-0, 2008. a, b
Liang, C.-K., West, J. J., Silva, R. A., Bian, H., Chin, M., Davila, Y., Dentener, F. J., Emmons, L., Flemming, J., Folberth, G., Henze, D., Im, U., Jonson, J. E., Keating, T. J., Kucsera, T., Lenzen, A., Lin, M., Lund, M. T., Pan, X., Park, R. J., Pierce, R. B., Sekiya, T., Sudo, K., and Takemura, T.: HTAP2 multi-model estimates of premature human mortality due to intercontinental transport of air pollution and emission sectors, Atmos. Chem. Phys., 18, 10497–10520, https://doi.org/10.5194/acp-18-10497-2018, 2018. a, b
Lidskog, R., Johansson, J., and Sjödin, D.: Wildfires, responsibility and trust: public understanding of Sweden's largest wildfire, Scand. J. Forest Res., 34, 319–328, https://doi.org/10.1080/02827581.2019.1598483, 2019. a
Lin, M., Fiore, A. M., Horowitz, L. W., Cooper, O. R., Naik, V., Holloway, J., Johnson, B. J., Middlebrook, A. M., Oltmans, S. J., Pollack, I. B., Ryerson, T. B., Warner, J. X., Wiedinmyer, C., Wilson, J., and Wyman, B.: Transport of Asian ozone pollution into surface air over the western United States in spring, J. Geophys. Res.-Atmos., 117, D00V07, https://doi.org/10.1029/2011JD016961, 2012. a
Liu, J. C., Pereira, G., Uhl, S. A., Bravo, M. A., and Bell, M. L.: A systematic review of the physical health impacts from non-occupational exposure to wildfire smoke, Environ. Res., 136, 120–132, https://doi.org/10.1016/j.envres.2014.10.015, 2015. a, b
Maia, P., Keizer, J., Vasques, A., Abrantes, N., Roxo, L., Fernandes, P., Ferreira, A., and Moreira, F.: Post-fire plant diversity and abundance in pine and eucalypt stands in Portugal: Effects of biogeography, topography, forest type and post-fire management, Forest Ecol. Manag., 334, 154–162, https://doi.org/10.1016/j.foreco.2014.08.030, 2014. a
Moreira, F., Viedma, O., Arianoutsou, M., Curt, T., Koutsias, N., Rigolot, E., Barbati, A., Corona, P., Vaz, P., Xanthopoulos, G., Mouillot, F., and Bilgili, E.: Landscape – wildfire interactions in southern Europe: Implications for landscape management, J. Environ. Manage., 92, 2389–2402, https://doi.org/10.1016/j.jenvman.2011.06.028, 2011. a
Morgan, G., Sheppeard, V., Khalaj, B., Ayyar, A., Lincoln, D., Jalaludin, B., Beard, J., Corbett, S., and Lumley, T.: Effects of Bushfire Smoke on Daily Mortality and Hospital Admissions in Sydney, Australia, Epidemiology, 21, 47–55, https://doi.org/10.1097/EDE.0b013e3181c15d5a, 2010. a
Münzel, T., Gori, T., Al-Kindi, S., Deanfield, J., Lelieveld, J., Daiber, A., and Rajagopalan, S.: Effects of gaseous and solid constituents of air pollution on endothelial function, Eur. Heart J., 39, 3543–3550, https://doi.org/10.1093/eurheartj/ehy481, 2018. a
Neuberger, M., Schimek, M. G., Horak, F., Moshammer, H., Kundi, M., Frischer, T., Gomiscek, B., Puxbaum, H., and Hauck, H.: Acute effects of particulate matter on respiratory diseases, symptoms and functions: epidemiological results of the Austrian Project on Health Effects of Particulate Matter (AUPHEP), Atmos. Environ., 38, 3971–3981, https://doi.org/10.1016/j.atmosenv.2003.12.044, 2004. a
Niemi, J. V., Saarikoski, S., Aurela, M., Tervahattu, H., Hillamo, R., Westphal, D. L., Aarnio, P., Koskentalo, T., Makkonen, U., Vehkamäki, H., and Kulmala, M.: Long-range transport episodes of fine particles in southern Finland during 1999–2007, Atmos. Environ., 43, 1255–1264, https://doi.org/10.1016/j.atmosenv.2008.11.022, 2009. a
Nunes, K. V. R., Ignotti, E., and Hacon, S. d. S.: Circulatory disease mortality rates in the elderly and exposure to PM2.5 generated by biomass burning in the Brazilian Amazon in 2005, Cad. Saude Publica, 29, 589–598, https://doi.org/10.1590/S0102-311X2013000300016, 2013. a
Nunes, L. J., Meireles, C. I., Gomes, C. J., and Ribeiro, N. M.: Historical development of the Portuguese forest: The introduction of invasive species, Forests, 10, 881–888, https://doi.org/10.3390/f10110974, 2019. a, b
Oliveira, S., Zêzere, J. L., Queirós, M., and Pereira, J. M.: Assessing the social context of wildfire-affected areas. The case of mainland Portugal, Appl. Geogr., 88, 104–117, https://doi.org/10.1016/j.apgeog.2017.09.004, 2017. a, b
Pallarés, S., Gómez, E., Martínez, A., and Jordán, M. M.: The relationship between indoor and outdoor levels of PM10 and its chemical composition at schools in a coastal region in Spain, Heliyon, 5, e02270, https://doi.org/10.1016/j.heliyon.2019.e02270, 2019. a
Parente, J., Pereira, M., Amraoui, M., and Tedim, F.: Negligent and intentional fires in Portugal: Spatial distribution characterization, Sci. Total Environ., 624, 424–437, https://doi.org/10.1016/j.scitotenv.2017.12.013, 2018. a
Pearson, K. and Galton, F.: VII. Note on regression and inheritance in the case of two parents, P. R. Soc. London, 58, 240–242, https://doi.org/10.1098/rspl.1895.0041, 1895. a
Perrino, C., Tofful, L., Torre, S. D., Sargolini, T., and Canepari, S.: Biomass burning contribution to PM10 concentration in Rome (Italy): Seasonal, daily and two-hourly variations, Chemosphere, 222, 839–848, https://doi.org/10.1016/j.chemosphere.2019.02.019, 2019. a
Pinheiro, S. L. L. A., Saldiva, P. H. N., Schwartz, J., and Zanobetti, A.: Isolated and synergistic effects of PM10 and average temperature on cardiovascular and respiratory mortality, Rev. Saude Publ., 48, 881–888, https://doi.org/10.1590/S0034-8910.2014048005218, 2014. a
Qian, Z., He, Q., Lin, H.-M., Kong, L., Bentley, C. M., Liu, W., and Zhou, D.: High Temperatures Enhanced Acute Mortality Effects of Ambient Particle Pollution in the “Oven” City of Wuhan, China, Environ. Health Persp., 116, 1172–1178, https://doi.org/10.1289/ehp.10847, 2008. a
Rahman, A., Luo, C., Khan, M. H. R., Ke, J., Thilakanayaka, V., and Kumar, S.: Influence of atmospheric , PM10, O3, CO, NO2, SO2, and meteorological factors on the concentration of airborne pollen in Guangzhou, China, Atmos. Environ., 212, 290–304, https://doi.org/10.1016/j.atmosenv.2019.05.049, 2019. a
Rappold, A. G., Cascio, W. E., Kilaru, V. J., Stone, S. L., Neas, L. M., Devlin, R. B., and Diaz-Sanchez, D.: Cardio-respiratory outcomes associated with exposure to wildfire smoke are modified by measures of community health, Environ. Health-Glob., 11, 1–9, https://doi.org/10.1186/1476-069X-11-71, 2012. a
Rappold, A. G., Reyes, J., Pouliot, G., Cascio, W. E., and Diaz-Sanchez, D.: Community Vulnerability to Health Impacts of Wildland Fire Smoke Exposure, Environ. Sci. Technol., 51, 6674–6682, https://doi.org/10.1021/acs.est.6b06200, 2017. a
Reid, C. E., Brauer, M., Johnston, F. H., Jerrett, M., Balmes, J. R., and Elliott, C. T.: Critical review of health impacts of wildfire smoke exposure, Environ. Health Persp., 124, 1334–1343, https://doi.org/10.1289/ehp.1409277, 2016. a, b
Rovira, J., Domingo, J. L., and Schuhmacher, M.: Air quality, health impacts and burden of disease due to air pollution (PM10, PM2.5, NO2 and O3): Application of AirQ+ model to the Camp de Tarragona County (Catalonia, Spain), Sci. Total Environ., 703, 135 538, https://doi.org/10.1016/j.scitotenv.2019.135538, 2020. a
Samoli, E., Peng, R., Ramsay, T., Pipikou, M., Touloumi, G., Dominici, F., Burnett, R., Cohen, A., Krewski, D., Samet, J., and Katsouyanni, K.: Acute Effects of Ambient Particulate Matter on Mortality in Europe and North America: Results from the APHENA Study, Environ. Health Persp., 116, 1480–1486, https://doi.org/10.1289/ehp.11345, 2008. a
San-Miguel-Ayanz, J., Durrant, T., Boca, R., Libertà, G., Branco, A., De Rigo, D., Ferrari, D., Maianti, P., Artés Vivancos, T., Costa, H., Schulte, E., and Loffler, P.: Forest Fires in Europe, Middle East and North Africa 2017, EUR 28707 EN, Publications Office of the European Union, Luxembourg, https://doi.org/10.2760/663443, 2017. a
Schär, C., Vidale, P. L., Lüthi, D., Frei, C., Häberli, C., Liniger, M. A., and Appenzeller, C.: The role of increasing temperature variability in European summer heatwaves, Nature, 427, 332–336, https://doi.org/10.1038/nature02300, 2004. a
Settele, J., Scholes, R., Betts, R., Bunn, S., Leadley, P., Nepstad, D., Overpeck, J. T., and Taboada, M. A.: Terrestrial and inland water systems. In: Climate Change 2014: Impacts, Adaptation, and Vulnerability. Part A: Global and Sectoral Aspects, Contribution of Working Group II to the Fifth Assessment Report of the Intergovernmental Panel on Climate Change, edited by: Field, C. B., Barros, V. R., Dokken, D. J., Mach, K. J., Mastrandrea, M. D., Bilir, T. E., Chatterjee, M., Ebi, K. L., Estrada, Y. O., Genova, R. C., Girma, B., Kissel, E. S., Levy, A. N., MacCracken, S., Mastrandrea, P. R., and White, L. L., Cambridge University Press, Cambridge, United Kingdom and New York, NY, USA, 271–359, 2014. a
Tarín-Carrasco, P., Morales-Suárez-Varela, M., Im, U., Brandt, J., Palacios-Peña, L., and Jiménez-Guerrero, P.: Isolating the climate change impacts on air-pollution-related-pathologies over central and southern Europe – a modelling approach on cases and costs, Atmos. Chem. Phys., 19, 9385–9398, https://doi.org/10.5194/acp-19-9385-2019, 2019. a, b
Trentmann, J., Yokelson, R. J., Hobbs, P. V., Winterrath, T., Christian, T. J., Andreae, M. O., and Mason, S. A.: An analysis of the chemical processes in the smoke plume from a savanna fire, J. Geophys. Res.-Atmos., 110, D12301, https://doi.org/10.1029/2004JD005628, 2005. a
Turco, M., Jerez, S., Augusto, S., Tarín-Carrasco, P., Ratola, N., Jiménez-Guerrero, P., and Trigo, R. M.: Climate drivers of the 2017 devastating fires in Portugal, Sci. Rep.-UK, 9, 13 886, https://doi.org/10.1038/s41598-019-50281-2, 2019. a, b, c
World Health Organization: Wildfires and heat-wave in the Russian Federation, in: Public health advice, WHO Regional Office for Europe, Copenhagen, Denmark, p. 15, 2010. a