the Creative Commons Attribution 4.0 License.
the Creative Commons Attribution 4.0 License.
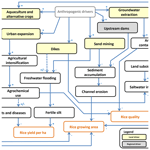
Interacting effects of land-use change and natural hazards on rice agriculture in the Mekong and Red River deltas in Vietnam
Tang Thi Hanh
Vu Duong Quynh
Adam D. Switzer
Paul Teng
Janice Ser Huay Lee
Vietnam is a major rice producer, and much of the rice grown is concentrated in the Red River Delta (RRD) and the Mekong River Delta (MRD). While the two deltas are highly productive regions, they are vulnerable to natural hazards and the effects of human-induced environmental change. To show that the processes and issues affecting food security are reinforcing, interdependent and operating at multiple scales, we used a systems-thinking approach to represent the major linkages between anthropogenic land-use and natural hazards and elaborate on how the drivers and environmental processes interact and influence rice growing area, rice yield and rice quality in the two deltas. On a local scale, demand for aquaculture and alternative crops, urban expansion, dike development, sand mining and groundwater extraction decrease rice production in the two deltas. Regionally, upstream dam construction impacts rice production in the two deltas despite being distally situated. Separately, the localized natural hazards that have adversely affected rice production include droughts, floods and typhoons. Outbreaks of pests and diseases are also common. Climate-change-induced sea level rise is a global phenomenon that will affect agricultural productivity. Notably, anthropogenic developments meant to improve agricultural productivity or increase economic growth can create many unwanted environmental consequences such as an increase in flooding, saltwater intrusion and land subsidence, which in turn decreases rice production and quality. In addition, natural hazards may amplify the problems created by human activities. Our meta-analysis highlights the ways in which a systems-thinking approach can yield more nuanced perspectives to tackle “wicked” and interrelated environmental challenges. Given that deltas worldwide are globally significant for food production and are highly stressed and degraded, a systems-thinking approach can be applied to provide a holistic and contextualized overview of the threats faced in each location.
- Article
(3983 KB) -
Supplement
(90 KB) - BibTeX
- EndNote
A delta is defined as a low-lying sedimentary landform located at the mouths of rivers. The mixing of fresh water and saltwater in these sediment-rich land–ocean coastal zones provides fertile land for agricultural activities to support a large number of people. Besides agriculture, resources in deltas have also been tapped for fisheries, navigation, trade, forestry, fossil energy production and manufacturing. Unfortunately, deltas are highly vulnerable to a range of environmental hazards such as typhoons, floods, storm surges, tsunamis, coastal erosion and seasonal inundations (Seto, 2011). In addition, local human activities, land subsidence, water stresses and global sea level rise have exacerbated their environmental vulnerability (Day et al., 2016; Syvitski and Saito, 2007; Tessler et al., 2015). The threats faced by deltas are considered to be “wicked problems” with no easy solutions to counter them (DeFries and Nagendra, 2017).
In this paper, we focus on the Red River Delta (RRD) and the Mekong River Delta (MRD) in Vietnam as these two deltas are highly populated hubs of agricultural production that are highly vulnerable to environmental hazards. We use a systems-thinking approach to illustrate some of the wicked problems present in the two deltas in Vietnam and the implications of these anthropogenic and natural-hazard drivers on rice agriculture. Although a variety of crops are cultivated in Vietnam, we focus on rice as it is a staple food for the Vietnamese (M. T. Nguyen et al., 2019; USDA, 2012), and it is also a key export crop. In 2019, Vietnam exported USD 1.4 billion of rice and was the fourth-largest rice exporter in the world, contributing 6.6 % of the world's total rice exports (Workman, 2020).
Many studies have investigated how Vietnam is affected by natural hazards or anthropogenic land-use change (cf. Howie, 2005; Minderhoud et al., 2018; K.-A. Nguyen et al., 2019; Vinh et al., 2014). Several studies go a step further to examine how changes in anthropogenic land use have affected rice productivity. For example, higher prices and rising demand for aquaculture and non-rice products have incentivized farmers to shift away from rice monoculture to embrace non-rice crops (Hai, 2021; Morton, 2020). In addition, environmental threats such as worsening saltwater intrusion have limited rice production areas and forced many farmers to convert their now-unusable rice fields into shrimp ponds (Kotera et al., 2005; Nguyen et al., 2017) or turn to growing salt-tolerant crops such as coconut, mango and sugar cane (Nguyen and Vo, 2017). Urban expansion is also another key factor that has reduced rice growing areas although agricultural intensification has kept rice yields high despite shrinking growing areas (Drebold, 2017; Morton, 2020).
Meanwhile, the construction of high dikes to mitigate flooding in the Mekong River Delta has facilitated triple-cropping of rice and increased yields. However, these high dikes reduce the availability of fertile silt and force farmers to rely on costly agrochemicals to maintain yields (Chapman and Darby, 2016; Tran and Weger, 2018). Though there is substantial research on sand mining and upstream dam construction, these non-related anthropogenic factors are often not linked to agricultural productivity even though reduced sediment availability and increased channel erosion would have adverse implications on agricultural productivity (Binh et al., 2020; Park et al., 2020; Jordan et al., 2019).
Besides land-use change, rice grown in the RRD and MRD is susceptible to damage from natural hazards such as typhoons, floods and droughts (Chan et al., 2012, 2015; Grosjean et al., 2016; Terry et al., 2012). Rice crops can be damaged by strong winds and flooding from heavy rain associated with a typhoon event. Rice damage is worse if the typhoon occurs during the vulnerable heading or harvesting periods (Masutomi et al., 2012). In addition, floods can also be caused by heavy monsoonal rains. Notably, moderate levels of freshwater flooding may be beneficial to agricultural production (Chapman et al., 2016). On the other hand, droughts, while uncommon, have caused millions in economic loss, particularly in the agriculture sector (Grosjean et al., 2016). The most recent 2015–2016 drought affected all the Mekong River Delta provinces and caused up to USD 360 million in damage, of which USD 300 million was agriculture- and aquaculture-related damage (Nguyen, 2017).
Arias et al. (2019) conceptually integrated local and regional drivers of change to illustrate the factors contributing to environmental change in the Mekong floodplains. Similarly, M. T. Nguyen et al. (2019) recognized that there are various drivers of change associated with adapting to widespread salinity intrusion in the Mekong and Red River deltas, and these drivers are constantly interacting with and providing feedback to each other. On a more technical level, Chapman and Darby (2016) used system dynamics modeling to simulate the delays, feedbacks and tipping points between farmers' socioeconomic status and the practice of double- or triple-cropping in An Giang Province in the MRD. Building on the framework provided by these studies, we use systems-thinking to present an overarching picture of how anthropogenic and natural-hazard drivers can interact to reinforce or diminish rice production. While many studies may have highlighted the links between the various drivers and rice production, we seek to integrate the different drivers of anthropogenic change and natural hazards to show their inter-related and interdependent nature and how the processes associated with each driver affect rice growing areas, rice production and rice quality in general.
The use of systems-thinking is appropriate as the wicked environmental problems present in the deltas of Vietnam are caused by a range of interdependent anthropogenic and natural hazards drivers operating at multiple scales with no easily identifiable, predefined solutions. While interventions may be made to ameliorate problems, these interventions may create feedbacks and unanticipated outcomes (Rittel and Webber, 1973; DeFries and Nagendra, 2017). In addition, systems-thinking can be applied in a range of contexts and at multiple scales. Geist and Lambin (2002) and Lim et al. (2017) applied a system-dynamics approach to understand drivers of deforestation and forest degradation at the national and global scales, while Ziegler et al. (2016) used a transdisciplinary learning approach to understand the role of environmental and cultural factors in driving the development of human diseases in northeastern Thailand at the landscape scale.
Our aim is to use a literature review to develop flow diagrams to represent the major linkages between anthropogenic land-use factors and natural hazards and elaborate on how they interact and influence rice productivity in these two deltas. Due to the importance of Vietnam as a major rice producer and exporter in Southeast Asia as well as the range of threats faced by the rice sector from natural hazards and anthropogenic land use, we hope to show how the processes and issues affecting food security are not one-dimensional and linear but in fact reinforcing and interdependent. Lastly, given that deltas worldwide are globally significant for food production and are highly stressed and degraded landscapes, we argue that a systems-thinking approach can be applied to provide a holistic and contextualized overview of the threats faced in each location.
2.1 Study sites
The Mekong River Delta (MRD) is the world's third-largest delta, with a physical area of 4 million ha, and it is the larger of the two deltas in Vietnam (Schneider and Asch, 2020; Fig. 1). In 2018, the planted area for spring, autumn and winter paddies was 1.6 million ha, 2.3 million ha and 197.2 thousand ha, respectively. In total, 4.1 million ha of rice was planted over the three planting seasons, with 24.5 million tonnes of rice produced. The delta is home to 17.8 million people, with many dependent on agriculture for their livelihoods. That 54 % of Vietnam's rice is grown in the MRD, and most of it is exported overseas, makes it strategically important for the Vietnamese economy and for global food security (Chapman et al., 2016; Cosslett and Cosslett, 2018; General Statistics Office of Vietnam, 2020). Up north, the Red River Delta (RRD) is the next largest, with a physical delta area of 1.5 million ha (Fig. 1; Schneider and Asch, 2020). In 2018, 1 million ha of planted rice produced 6.3 million tonnes of rice, the equivalent of 14 % of Vietnam's total rice production (524.3 and 516.4 thousand ha of rice was planted in the spring and winter seasons, respectively). Approximately 21.6 million people live in the RRD, with many also dependent on agriculture (General Statistics Office of Vietnam, 2020).
Soils in the MRD are highly variable, with alluvial, acid sulfate and saline soils dominant. Most of the rice grows in the highly fertile alluvial soils, which are found in only 30 % of the delta (GRSP, 2013). Conversely, soils in the RRD consist of Holocene delta sediments. These Holocene delta sediments are relatively fine-grained muds and sands, up to 30 m thick, that are the product of rapid progradation during the Holocene high sea level stand (Mathers and Zalasiewicz, 1999). The Holocene sequence overlies coarse-grained Pleistocene sediments dominated by braided river and alluvial fan deposits formed during the last glacial low sea level stand. The Quaternary sediments are underlain by a >400 m thick layer of Neogene sedimentary rocks that are made up of conglomerate sandstone, clay and siltstone (Berg et al., 2007).
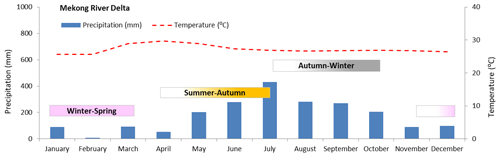
Figure 2Climograph for the Mekong River Delta annotated with the winter–spring, summer–autumn and autumn–winter growing seasons. The color gradient (from faded to dark) represents planting, growing and harvesting times for each crop. Precipitation and temperature data were taken from the Statistical Yearbook of Vietnam 2018 (General Statistics Office, 2018).
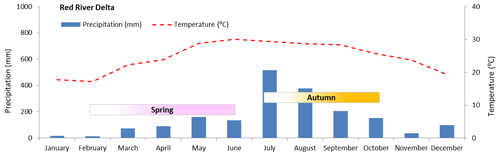
Figure 3Climograph for the Red River Delta annotated with the spring and autumn growing seasons. The color gradient represents planting, growing and harvesting times for each crop. Precipitation and temperature data were taken from the Statistical Yearbook of Vietnam 2018 (General Statistics Office, 2018).
Climatically, the MRD has a tropical monsoon climate with two distinct seasons, a dry season from December to April and a rainy season from May to November. It is generally warm year round, with average temperatures in 2018 ranging from 26 ∘C in December, January and February to 29 ∘C in May. The annual rainfall is between 2000 and 2400 mm (General Statistics Office, 2018; Kotera et al., 2008). Conversely, the RRD has a tropical monsoon climate with three seasons: (1) a hot and wet season from May to September, (2) a cool and dry season from October to January, and (3) a cool and humid season from February to April. The hot and wet season is characterized by high temperatures and high rainfall, the cool and dry season has moderate to low temperatures and low rainfall, and the cool and humid season has low to moderate temperatures and low rainfall (Huong et al., 2013; Li et al., 2006). In 2018, the monthly average temperatures in the RRD ranged from 17 ∘C in February to 30 ∘C in June. Average annual rainfall is between 1300 and 1800 mm (Li et al., 2006; General Statistics Office, 2018). Both deltas are low-lying, with elevations ranging from 0.7 to 1.2 m a.s.l. (above sea level) (Binh et al., 2017).
In the MRD, favorable environmental conditions with ample rainfall, tropical temperatures and fertile alluvial soils, coupled with an extensive dike and irrigation system, have facilitated the production of three rice crops annually: winter–spring, summer–autumn and autumn–winter (Table 1; Fig. 2). In 2018, the summer–autumn crop was the largest (12.8 million tonnes), and the winter–spring crop was the second-largest (10.8 million tonnes), followed by the autumn–winter crop (909.6 thousand tonnes) (General Statistics Office of Vietnam, 2020). Compared to the MRD, rice is planted biannually in the RRD, first from February to June (spring crop) and a second time from July to October (autumn crop) (Table 1; Fig. 3). The chilly winters preclude the cultivation of a third crop of rice. Approximately 3.5 million tonnes of rice was produced during the spring cropping season, while 2.8 million tonnes was produced during the autumn season in 2018 (General Statistics Office of Vietnam, 2020).
2.2 Literature review and causal loop diagrams
We conducted an online search on Scopus, Web of Science, Google, Google Scholar and individual journal databases to find articles related to the effects of anthropogenic land-use change and natural hazards on rice agricultural systems in the RRD and/or MRD. Articles related to the environmental impacts of these anthropogenic interventions and natural hazards were included as these tend to explain the environmental processes in detail. A range of literature sources including peer-reviewed journal articles, book chapters and scientific reports from non-governmental organizations were included. In addition, we reviewed the bibliographies of our articles to follow up with any other relevant literature that was not listed in our search. Since sea level rise would affect the viability of the two deltas as major rice producing regions (Mainuddin et al., 2011), we also included relevant articles on sea level rise.
We obtained 126 articles through our literature search (cf. Supplement). Every article was considered to be a single case study and was read in detail by the lead author. Thereafter, the natural or anthropogenic drivers and/or the environmental process that would lead to a change in rice productivity directly or indirectly were identified. Adopting a systems-thinking approach, we constructed flow diagrams to identify and visualize the interconnections among the drivers of rice productivity in both deltas.
We first developed causal links which describe how an anthropogenic driver would influence rice productivity either directly or through an environmental process. We also documented if each driver had an increasing or decreasing effect on an environmental process that could influence rice productivity by affecting rice growing area, rice yield or rice quality. This relationship is represented by an arrow, which indicates the direction of influence, from cause to effect. The polarity of the arrows (plus or minus) indicates whether the effect is increasing or decreasing (Lim et al., 2017). A plus sign indicates that a link has “positive polarity”, and a minus sign indicates “negative polarity.” The polarity of the causal link between A and B is said to be positive when an increase (decrease) in A causes B to increase (decrease). A causal link is negative when an increase (decrease) in A causes B to decrease (increase) (Newell and Watson, 2002). We constructed two flow diagrams; the first flow diagram describes how anthropogenic land-use drivers affect rice growing area, rice yield per hectare and rice quality in the MRD and RRD (Fig. 4), while the second causal flow diagram describes how natural hazards in the MRD and RRD affect rice growing area, rice yield per hectare and rice quality (Fig. 6). The references we used are found in the Supplement.
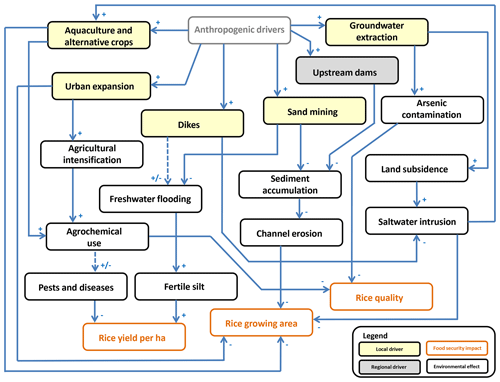
Figure 4Flow diagram showing the key anthropogenic drivers that affect rice production in the two mega-deltas of Vietnam. The drivers are classified as a local driver if it occurs in the two mega-deltas. Regional drivers are those that occur farther away from the two mega-deltas but within the Asian region. A plus (+) sign indicates that an increase (decrease) in A causes B to increase (decrease). A negative (−) sign indicates that an increase (decrease) in A causes B to decrease (increase). Dashed lines with “±” are used when outcomes are unclear. For example, dikes reduce flooding, but poorly maintained or planned dikes increase flooding instead. In addition, dikes may potentially cause flooding in unprotected areas. Agrochemical use may reduce the incidence of pests and diseases, but the overuse of chemicals can lead to pesticide resistance, which may increase outbreaks of pests and diseases.
3.1 Local anthropogenic drivers
3.1.1 Aquaculture and alternative crops
Both deltas face widespread salinity intrusion that threatens rice production. In the Mekong River Delta, salinity intrusion is a naturally occurring phenomenon during the dry season. Tides from the South China Sea and the Gulf of Thailand bring saltwater inland, and salinity intrudes up to 70–90 km inland as the length of sea dikes is limited. There are 1500 km of sea and estuary dikes in RRD versus 450 km of sea dikes in the MRD (Le et al., 2018; Preston et al., 2003; Pilarcyzk and Nguyen, 2005). Thus, salinity intrusion extends up to 20 km from the main river in the RRD (Ca et al., 1994). As rice plants are unable to thrive in soils with soil salinities exceeding 4 g L−1 (V. H. T. Pham et al., 2018), affected farmers have converted their paddy fields into aquaculture ponds to cultivate shrimp and fish instead. Other farmers have turned to planting salinity-tolerant crops such as coconut, mango and sugarcane. In some cases, farmers have opted for a rice-aquaculture system, whereby rice is planted in the wet season, and fish and shrimp are cultivated in the dry season, when soil salinities are high (Nguyen and Vo, 2017; Pham et al., 2017).
Besides environmental factors, the greater profitability of fruits, vegetables, fish and shrimp also incentivizes farmers to plant more non-rice crops. The income per hectare of rice was USD 146 compared to pomelo (USD 16 844) and coconut (USD 1484) (Hoang and Tran, 2019). Meanwhile, farmers who practice shrimp–rice rotational systems earned 50 % more than those who had two rice crops (Morton, 2020; Schneider and Asch, 2020). In addition, high demand for fruits and vegetables for export and from growing urban populations with greater affluence and knowledge about nutrition also encouraged farmers to diversify from rice monoculture, leading to possible declines in the overall rice growing area (Hai, 2021; Nguyen and Vo, 2017; Fig. 4).
Finally, government policies encouraging farmers to move away from growing rice also contributed to the planting of more non-rice crops on paddy land. As a result, there is increased use of paddy land for non-rice crops, orchards, and freshwater and brackish aquaculture (Van Kien et al., 2020). Using remote sensing to assess land-use and land-cover change in the Mekong River Delta, Liu et al. (2020) found that aquaculture had become the second-largest land-use type, following planted land. This was facilitated by government regulations, salt intrusion and higher profitability of aquaculture productions. In any case, even though farmers may have moved on from growing rice, many still continue to apply excessive quantities of pesticides on their crops (Normile, 2013; Fig. 4).
3.1.2 Urban expansion
Rapid urbanization is accelerating the loss of agricultural land in both the Red River Delta and Mekong River Delta. In Hanoi, a major city in the Red River Delta, 1420 ha of agricultural land was lost per year from 2000–2007, equivalent to a yearly loss of 3 %. By 2025, up to 450 000 ha of agricultural land is expected to be converted to urban land. Most of this land-use conversion occurs in peri-urban areas 5–15 km from the city center. The same peri-urban land is often used to grow food, flowers and livestock for the urban population in Hanoi. However, this land is considered by local authorities to be land reserve for urban planning instead of resources for food supply (Drebold, 2017; Pham et al., 2015). Most of this peri-urban agricultural land is often forcibly obtained, with minimal compensation given to farmers, and then sold to foreign developers (Drebold, 2017). Similar urban expansion is also occurring in Can Tho city in the Mekong River Delta, with corresponding losses of agricultural land (cf. Garschagen et al., 2011; Pham et al., 2010). A decline in agricultural land means a decline in rice growing areas as well (Fig. 4).
High land prices and a shrinking availability of arable land have forced farmers to practice agricultural intensification. In the RRD, rice production grew more than 25 % from 2000–2011 without corresponding increases in rice growing areas due to intensified cropping practices involving the use of new high yielding rice varieties, irrigation during the dry season and high inputs of agrochemicals (Drebold, 2017; Morton, 2020). The high pesticide use was reflected in a study in Nam Dinh Province in the RRD, where 8 out of 12 target pesticides were found in agricultural soils. In this study, frequently detected pesticides include isoprothiolane, chlorpyrifos and propiconazole, and besides polluting the environment, the presence of high concentrations of pesticide residues also lowers the quality of rice sold for consumption (Braun et al., 2018; Fig. 4).
In general, pests such as the brown planthopper are naturally occurring and are not a threat at low densities. However, intensive rice production with high seeding densities and the use of susceptible varieties create a constant supply of food, which allows their numbers to balloon. This is exacerbated by the asynchronous planting, which creates a continuous supply of rice plants throughout the year in the Mekong River Delta. In addition, the overuse of nitrogen fertilizers increases the pests' reproductive potential. Thirdly, the excessive use of pesticides also kills the natural enemies of pests such as spiders, ants, bees, beetles, dragonflies, frogs, ladybugs and wasps. Besides killing the natural predators of rice pests, the pests targeted by the pesticides may also become resistant to the pesticide. As a result, higher doses of the pesticide may be needed to kill them in the future. For example, killing planthoppers now requires a pesticide dose 500 times larger than was needed in the past (Normile, 2013). The overuse of pesticide is due to a combination of factors such as insufficient knowledge of its proper use as well as aggressive marketing by agrochemical companies (Bottrell and Schoenly, 2012; Normile, 2013; Sebesvari et al., 2011). The dashed lines in Fig. 4 show that pesticide use may not necessarily reduce the incidence of pests and diseases if excessive volumes were applied.
3.1.3 Dikes
Wet-season flooding is a naturally occurring phenomenon in the two deltas of Vietnam (Chan et al., 2012, 2015). To facilitate the planting of rice during the wet season, flood prevention dikes were constructed to keep floodwaters out (Fig. 5). The MRD has more than 13 000 km of flood prevention dikes, of which 8000 km are low dikes below 2 m tall. These low dikes were mostly constructed before 2000 to delay the entry of floodwaters at the start of the monsoon season to allow two rice crops to be grown. A severe flood in 2000 provided the impetus for river dikes to be heightened to 3.5 m to completely keep floodwaters out. These high dikes have facilitated triple-cropping in the MRD, particularly in Dong Thap and An Giang provinces (Chapman et al., 2016; Howie, 2005; Le et al., 2018; Triet et al., 2017). However, the presence of high dikes in the MRD has reduced the supply of fertile alluvium, increasing the need for artificial fertilizers and pesticides to maintain yields (Chapman et al., 2017; Fig. 4).
A study comparing sediment deposition in areas of high and low dikes in An Giang Province found that double-cropping farmers who cultivate their crops in areas with low dikes have an average of 2.5 cm of sediment deposition. This deposition by floodwaters improved their average annual input efficiency by 0.3 t of yield per tonne of fertilizer. Conversely, triple-cropping farmers had very little deposition, averaging 0.5 cm as the high dikes kept floodwaters out. Some deposition was found only if there had been a dike breach which also caused crop damage (Chapman et al., 2016). The value of flood deposits is reiterated by the study of Manh et al. (2015), which estimated that the annual deposition of sediment-bound nutrients can naturally supply over half of the fertilizers needed for a season of rice crop. The provision of “free” fertilizers by the encroaching floodwaters benefits the less economically endowed farmers who must purchase artificial fertilizers to maintain yields (Chapman et al., 2017; Kondolf et al., 2018; Fig. 4).
However, poorly planned and/or maintained dikes are not only functionally ineffective against floodwaters or coastal surges; they may also become an amplifier of destruction when their presence creates a false sense of security which results in intensive development of low-lying areas (Mai et al., 2009; Tran et al., 2018). In addition, areas unprotected by dikes may be more vulnerable to flooding as the excess water has to flow somewhere. Using a GIS-linked numerical model, Le et al. (2007) confirmed that engineering structures in the MRD increased water levels and flow velocities in rivers and canals. This in turn increased the risk of flooding in both non-protected areas and protected areas (due to dike failure). Dashed lines are used in Fig. 4 to show that dikes do not necessarily reduce flooding.
Likewise, the RRD is also heavily diked, with 3000 km of river dikes (Fig. 5), but unlike the MRD, high dikes are absent (Pilarcyzk and Nguyen, 2005). Besides river and flood control dikes, there are also sea dikes and salinization prevention dikes in both deltas to protect the area from salinity intrusion. There are 1500 km of sea and estuary dikes in the RRD. In the MRD, there are 1290 km of salinization prevention dikes and 450 km of sea dikes (Le et al., 2018; Pilarczyk and Nguyen, 2005; Fig. 4).
3.1.4 Sand mining
Sand mining is carried out on a large scale in the Mekong (Kondolf et al., 2018). Fueled by demand from reclamation, export and construction, 55.2 million tonnes of sediment was extracted from the Mekong main stem in Laos, Thailand, Cambodia and Vietnam from 2011 to 2012 (Bravard and Gaillot, 2013; Robert, 2017). A more recent analysis of bathymetric maps and the local refilling processes by Jordan et al. (2019) put the amount of sand extracted from the Mekong River Delta in 2018 at 17.77 Mm3.
Besides removing large quantities of riverbed sediments, sand mining operations have created numerous pits and pools. These pits and pools, which can be up to 45 m deep, then become sediment traps, trapping bed loads from upstream reaches and preventing them from traveling downstream and contributing to the continued presence and growth of the delta. In addition, bed incision also occurs as the water is sediment-starved. The down-cutting of riverbanks can propagate upstream and downstream from the extraction sites for many kilometers, in turn affecting river ecosystems over a large area (Kondolf et al., 2018). This bank incision results in land loss, which threatens rice growing areas (Fig. 4).
Aggressive sand mining also disrupts natural flooding. A recent study of the effects of riverine mining on flood frequency in the Long Xuyen Quadrangle (LXQ) in the Mekong River Delta found that flood frequency had dropped by 7.8 % from 2005–2015. Water levels at local gauge stations also showed an overall decreasing trend, indicating that the lowering of the riverbed had reduced the frequency of flooding. Disrupted flood regimes result in reduced volumes of water and sediments for agricultural production. In addition, floodwaters typically deposit fertile sediments while flushing the pesticides and fertilizers accumulated from intensive agricultural production. When the flood frequency decreases, the frequency at which farmlands benefit from this natural soil quality enhancement decreases. Consequently, soil fertility may decrease over time and lead to declines in rice yields unless artificial fertilizers are added (Park et al., 2020; Fig. 4).
While there are several studies on the diffuse, yet insidious nature of sand mining in the Mekong (cf. Bravard and Gaillot, 2013; Brunier et al., 2014; Jordan et al., 2019; Kondolf et al., 2018; Park et al., 2020; Robert, 2017; Schmitt et al., 2017), the extent of sand mining in the Red River Delta is unclear as there is almost no research on this issue. We did however come across an article in a Vietnamese newspaper about rampant sand mining in the Red River and how mining operations have caused erosion in nearby villages (Chinh, 2018). Similar to the situation in the MRD, the authorities have turned a blind eye to this illegal business (Bravard and Gaillot, 2013; Chinh, 2018).
3.1.5 Groundwater extraction
Another example of an anthropogenic development creating other interrelated problems is that of groundwater extraction. While groundwater extraction has increased the availability of water for human activities, it has exacerbated land subsidence, which has increased the severity and extent of saltwater intrusion and reduced the suitability of land for rice cultivation (Fig. 4). Minderhoud et al. (2017) developed a 3D numerical groundwater flow model of the MRD surface and found that subsidence rates from groundwater extraction were between 1.1 and 2.5 cm yr−1. The model also showed that 25 years of groundwater extraction since 1991 had resulted in a cumulative average of 18 cm of subsidence, with some hotspots recording over 30 cm of subsidence. Land subsidence from excessive groundwater extraction acts as a catalyst that increases vulnerability to saltwater intrusion and reduces the availability of land suitable for rice production.
Moreover, rice crops become contaminated with arsenic when arsenic-rich groundwater used for non-agricultural use is discharged into rivers, and the river water is used for rice irrigation (Lan and Giao, 2017; Minderhoud et al., 2018). High arsenic concentrations in groundwater seem to be of natural origin. In the Mekong River Delta, naturally occurring biochemical and hydrological processes cause As to be released from Fe oxides in rocks and sediments into groundwater reservoirs (Fendorf et al., 2010). In addition, deep groundwater extraction causes interbedded clays to compact and expel water containing dissolved As (Erban et al., 2013). Crop quality is reduced when the arsenic-enriched water is deposited on topsoils and absorbed by rice plants during growth (Rahman and Hasegawa, 2011; Fig. 4).
Similarly, prevalence of groundwater extraction is also high in the Red River Delta. Approximately 70 % of the population living in the RRD accesses water from Holocene and Pleistocene aquifers (Berg et al., 2007; Winkel et al., 2011). Groundwater in the Red River Delta is also contaminated with high levels of As due to reductive dissolution of As from iron oxyhydroxides in buried sediment (Berg et al., 2007; Luu, 2019). Berg et al. (2007) sampled 196 tube wells randomly over a 700 km2 area in the Red River Delta, and the concentrations of As in groundwater ranged from 1 to 3050 µg L−1, with an average of 159 µg L−1. Separately, Winkel et al. (2011) collected 512 water samples from private wells in the Red River floodplain and found As concentrations varying from <0.1 to 810 µg L−1, with 27 % of the samples exceeding the WHO guideline value of 10 µg L−1. The high concentrations of As reduce the quality of rice harvested in the RRD if As gets into soils and river waters indirectly through the usage of As-enriched groundwater (Fig. 4). In a study on As accumulation in white rice from the Red River region in Vietnam, Phuong et al. (1999) reported As values of between 0.03 and 0.47 µg g−1 d wt with the mean value at 0.21 µg g−1 d wt. The mean value was higher than the mean value reported for Thai rice (0.14 µg g−1 d wt; range: 0.01 to 0.39 µg g−1) (Meharg et al., 2009).
Lastly, although high As concentrations in groundwater are common in the RRD (cf. Berg et al., 2007; Luu, 2019; H. V. Pham et al., 2018; Winkel et al., 2011), there is no research on (groundwater induced) land subsidence in the Red River Delta. Thus, the magnitude of land subsidence in the delta is uncertain and should be an area for future research.
3.2 Regional anthropogenic drivers
3.2.1 Upstream dams
The Mekong River originates in the Tibetan Plateau and flows through China, Myanmar, Laos, Thailand, Cambodia and southern Vietnam. To meet growing demands for electricity, many small- and large-scale hydropower projects have been commissioned in each country to take advantage of this supposedly green and clean source of energy (Nhan and Cao, 2019; Manh et al., 2015). A total of 241 dams have been completed in the entire Mekong basin, with another 29 under construction. A further 91 are currently being planned. These 361 dams consist of 176 hydropower dams and 185 irrigation dams. Of the 364 dams in the Mekong, 20 are in Vietnamese territory (WLE Mekong CGIAR, 2020a). Conversely, the Red River originates in Yunnan Province in China and flows towards northern Vietnam. It is less heavily dammed, with a total of 105 dams in China and Vietnam. There are 25 hydropower dams, 3 multi-purpose dams and 9 irrigation dams in the Red River basin in Vietnam (Vinh et al., 2014; WLE Mekong CGIAR, 2020b). While there are no dams in the Mekong River Delta or the Red River Delta due to their relatively flat elevation, upstream dam development influences downstream regions in many ways, with the environmental impacts extending far beyond the dam itself (Kondolf et al., 2014).
Firstly, a substantial amount of coarse sand, gravel and suspended sediment is impounded in reservoirs behind the dams instead of being transported downstream. This diminished sediment load may aggravate erosion downstream from the dam (Nhan and Cao, 2019; Fig. 4). Using a network model, under a “definitive future” scenario of 38 new dams, the cumulative sediment reduction in the Mekong River Delta would be 51 %. Conversely, under full build-up of 133 new dams, only 4 % of the pre-dam sediment load will reach the delta (Kondolf et al., 2014). Manh et al. (2015) also reached similar conclusions with a quasi-2D hydrodynamic model of suspended sediment dynamics. Floodplain sedimentation would decrease by about 21 % to 96 %, while sediment load supplied to the sea at the river will diminish by 1 % to 95 %, with the extreme values representing full dam build-up. Even if dam construction was limited to the river tributaries instead of the main stem, the cumulative sediment trapped could be as high as 68 %, meaning that only about 32 % of the sediment load would reach the Mekong River Delta (Kondolf et al., 2014).
Indeed, Binh et al. (2020) found that the suspended sediment loads in the MRD had decreased by 74.1 % in 2012–2015 primarily due to six mainstream dams in the Lancang cascade in China. In particular, the Manwan and Dachaoshan dams contributed to 32 % of the reduction. In addition, from 2014–2017, the average incision rate of the Tien River in the MRD was 3 times higher than the previously recorded value. Sand mining was responsible for a maximum of 14.8 % of the annual riverbed incision, while the remainder was caused by hydropower dams upstream.
The deleterious impacts of dams on sediment loads can also be found in the Red River Delta in spite of the smaller number of dams. While the Hoa Binh dam is located on a tributary of the Red River in Vietnam, its large size has influenced suspended sediment distribution in the lower Red River basin. For example, an analysis of the suspended sediment concentration over a 50-year period from 1960 to 2010 showed that yearly suspended sediment flux had dropped by 61 % at Son Tay near Hanoi (Vinh et al., 2014). Similarly, Duc et al. (2012) calculated that the suspended sediment budget at Son Tay and Hanoi hydrological monitoring stations was reduced by 56 % after the Hoa Binh dam became operational in 1989. The reduction in sediment loads at the Red River Delta would likewise have a similar impact on delta size and rice growing areas.
Besides a change in sediment loads, dams also alter stream discharge and water levels, with concurrent effects on water supplies (not shown in Fig. 4). When water levels are high during the rainy season, dams can be used to impound the excess water in the reservoir behind. During the dry season, when water levels are lower, the dams can release water downstream. In doing so, dams increase dry-season discharge and decrease wet-season discharge. The modification of seasonal water flows is problematic as changes in natural flow patterns, such as higher flows in the dry season and lower flows in the wet season, would affect rice production as rice growing calendars are currently linked to the natural fluctuations in high and low flows (Robert, 2017). Hence, changes in water levels due to anthropogenic interventions may create unfavorable conditions for crop growth if planting calendars remain unchanged. In addition, lowered water levels during dry seasons due to upstream water impoundment can also lead to increased saltwater intrusion and create unfavorable growing conditions for rice farmers.
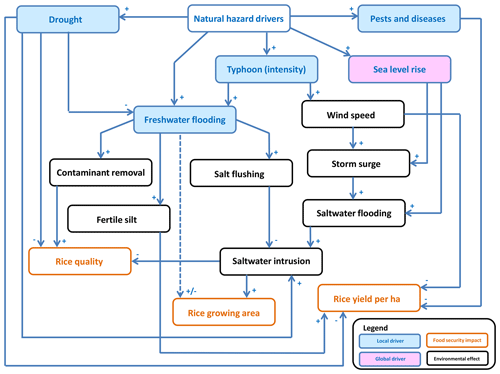
Figure 6Flow diagram showing the natural hazards that affect rice production in the two mega-deltas of Vietnam. Local drivers refer to natural hazards that occur within the two mega-deltas. Although sea level rise has implications on a local scale, it is considered a global driver as it occurs on a global scale. The effect of flooding on rice growing areas is variable as other factors that affect crop mortality include the type of rice grown, the stage of rice growth, and the depth and length of submergence in floodwaters.
3.3 Local natural-hazard drivers
3.3.1 Drought
Droughts do not result solely from a lack of rainfall; they can also result from changes in the arrival of rains and the length of the wet season (Adamson and Bird, 2010; Lassa et al., 2016). Vietnam was affected by droughts in 1997–1998, 2002–2003, 2009–2010 and most recently in 2015–2016. The 2015–2016 drought was the most severe in 90 years (Grosjean et al., 2016). All 13 provinces in the Mekong River Delta were affected by the 2015 drought. Besides a lack of water for irrigation, the drought caused saltwater to intrude up to 70 km inland. Cumulatively, the drought and accompanying saltwater intrusion damaged 400 000 ha of rice crops, including 50 000 ha of paddy in Kien Giang and Ca Mau provinces in the MRD (Grosjean et al., 2016; Nguyen, 2017). Although there is no research on how droughts and salinity intrusion have affected rice quality in Vietnam, research from elsewhere has shown that water shortages and salt stress induce physiochemical alterations which affect the rice grains produced (Pandey et al., 2014; Razzaq et al., 2019; Fig. 6).
Compared to the MRD, research and reports on droughts in the Red River Delta are scarce. The UNW-DPC (2014) reported that the RRD experienced droughts from the end of 1998 to April 1999, which affected 86 140 ha of rice. Another drought occurred from January to February 2004, with the water level of the Red River at the lowest in 40 years. Low water levels were also reported in 2010; however drought conditions and saltwater intrusion were more severe in the MRD (Overland, 2010). The effect of droughts on rice agriculture – reduced yields from a lack of water and salinity intrusion – would also be similar in the RRD.
3.3.2 Freshwater flooding
Ranked as the second most severe natural hazard after typhoons, freshwater floods are caused by overflowing rivers or heavy monsoonal rains or associated with heavy rain from typhoons (Chan et al., 2012, 2015; Hung et al., 2012; McElwee et al., 2017). Theoretically, flooding reduces rice growing areas, but it is simplistic to assume that flooded fields result in immediate loss of rice crops. A study by Kotera et al. (2005) in the RRD showed that the type of rice, stage of rice growth, and lengths and depths of submergence were factors that influence the survival rates of rice crops. For example, the local variety Moc Tuyen was less resilient to submergence than the two other genetically improved high-yielding varieties. In terms of growth stage, rice plants at the tillering stage are more likely to succumb to submergence than those at the vegetative stage. Plants fully submerged for short durations (2 d) also had lower chances of survival than those that were partially submerged in floodwaters for longer durations (up to 8 d). As such the effect of flooding on rice growing areas is variable as other factors that affect crop mortality include the type of rice grown, the stage of rice growth, and the depth and length of submergence in floodwaters (Fig. 6).
Although severe flooding can disrupt agricultural activities, moderate levels of freshwater flooding bring benefits to (rural) farmers (EEPSEA, 2011). Floodwaters from rivers improve agricultural productivity by depositing nutrient-rich flood sediments on agricultural soils (Chapman et al., 2016). In addition, floods wash away contaminants, purify and recharge aquifers, kill pests, and mitigate saltwater intrusion (EEPSEA, 2011; Hoang et al., 2018; Fig. 6). Aquatic resources such as fish, crabs and snails also come in with the floodwaters, which local farmers can collect to supplement their incomes. Besides growing rice, it is also possible to cultivate vegetables, fish, prawns and ducks in the flooded fields (Nguyen and James, 2013). Towards the end of the flooding season and the start of the rice cultivation season, floodwaters provide the water needed to start growing rice (Hoa et al., 2008).
While there are investments in flood prevention measures with the construction and upgrading of river dikes, the construction and dredging of reservoirs and drainage canals, and the raising of roads and embankments, recent floods have caused substantial agricultural losses as current measures have proven to be inadequate (Hoa et al., 2008; Pilarczyk and Nguyen, 2005). In 2018, 39 000 ha of rice in the RRD was inundated by heavy rains and floods triggered by Typhoon Son-Tinh (VNA, 2018a). Similarly, due to a lack of embankments and/or poor construction and maintenance of existing embankments, more than 2000 ha of rice was lost during the annual floods in 2018. An Giang Province was the worst-affected, losing 1270 ha of rice (VNA, 2018b).
3.3.3 Typhoons
Typhoons are the most severe natural hazard that affects Vietnam. When a typhoon occurs, affected areas are exposed to strong winds of up to 50 m/s and up to 300 mm of rainfall in a day. As the rainy season in Vietnam coincides with the typhoon season, widespread flooding can be expected from heavy rain and overflowing rivers (CCFSC, 2005; Mai et al., 2009; K.-A. Nguyen et al., 2019). Storm surges can also occur when high winds pushing on the ocean's surface are combined with the effect of low pressure in the center of a typhoon (Takagi et al., 2013). A study of typhoon disasters in Vietnam since 1950 showed that half of the 450 typhoons recorded during the study period were accompanied by a storm surge of over 1 m, and 11 % were over 2.5 m high (Imamura and Van To, 1997). In the Red River Delta, Quynh et al. (1998) found that the maximum storm surge is usually between 1 to 1.5 m a.m.s.l. (above mean sea level). In short, rice production will be adversely affected by strong winds and widespread flooding from heavy precipitation and storm surges in the event of a typhoon. Saltwater flooding may reduce rice growing areas as rice is not adapted to withstand prolonged submergence and/or saline conditions. Additionally, strong winds damage rice plants, with both effects contributing to a reduction in rice yields (Fig. 6).
Although a stronger typhoon usually brings higher wind speeds, more rainfall, larger waves and higher storm surges (Larson et al., 2014), the quantity of agricultural losses depends on factors such as landfall location(s) and whether the typhoon occurs during the vulnerable heading or harvesting periods (Masutomi et al., 2012). For example, Typhoon Mirinae (2016), which made landfall in Nam Dinh as a tropical storm, damaged or submerged 225 216 ha of rice. Meanwhile Typhoon Nesat (2011) made landfall in Hai Phong with a similar intensity and caused only 3500 ha of rice damage. Conversely, Typhoon Kalmai (2014) made landfall in Quang Ninh as a slightly stronger category 1 storm and caused 20 000 ha of rice damage (Nhân Dân, 2014; United Nations Vietnam, 2016; Viêt Nam News, 2011).
An average of five to six typhoons affect Vietnam between June and November every year (Larson et al., 2014; Nguyen et al., 2007). Typhoon activity shifts from the north to the south as the year progresses. Therefore, peak activity in the north and southern part of Vietnam is in August and November, respectively (Imamura and Van To, 1997). We reviewed the Digital Typhoon (2021) database and found 303 typhoons that came within 500 km of Vietnam's coastline from 1995 to 2018. A total of 29 cyclones made their initial landfall in the Red River Delta, while only 4 cyclones made landfall in the Mekong River Delta during the study period – one each in 1973, 1996, 1997 and 2006 (unpublished results). Although the MRD is less prone to typhoons, two of the most recent typhoons caused significant damage despite each being classified as a tropical storm upon landfall. Typhoon Linda (1997) caused some 349 232 ha of rice to be submerged, while Typhoon Durian (2006) damaged 6978 ha of agricultural land (International Federation of Red Cross and Red Crescent Society, 2006; UN Department of Humanitarian Affairs, 1997).
Lastly, typhoons may not necessarily be bad all the time. Darby et al. (2016) combined suspended sediment load data from the Mekong River with hydrological model simulations to examine the role of typhoons in transporting suspended sediments and found that one-third (32 %) of the suspended sediment reaching the delta is delivered by runoff generated by rainfall associated with typhoons. When a typhoon affects areas upstream, the land receives higher-than-usual levels of rainfall, which may trigger landslides. This sediment can be transferred into rivers and delivered downstream. While the role of tropical typhoons in sediment mobilization is unclear given the lack of research in this area, such findings have important implications for the MRD as sand mining and upstream dams have caused sharp declines in fluvial sediment loads, with corresponding impacts on channel incision and flood frequencies (Brunier et al., 2014; Rubin et al., 2015; Park et al., 2020).
3.3.4 Pests and diseases
Examples of pests that occur in rice fields of Vietnam include the brown planthopper (BPH; Nilaparvata lugens Stål), whitebacked planthopper (WBPH; Sogatella furcifera Horvath) and small brown planthopper (SBPH; Laodelphax striatellus Fallen). These planthoppers not only damage plants by ingesting its sap; they also transmit pathogenic viruses that kill the plants. The BPH is a vector for the rice grassy stunt virus (RGSV) and the rice ragged stunt virus (RRSV); the WBPH transmits the southern rice black streaked dwarf virus (RBSDV), while the SBPH vectors the rice stripe virus (RSV) and the RBSDV (Bottrell and Schoenly, 2012; Matsukawa-Nakata et al., 2019). Other pests that affect rice include parasitic worms called nematodes. Root nematodes that affect deepwater and irrigated rice fields in the MRD include Hirschmanniella oryzae (rice root nematode), Hirschmanniella mucronata and Meloidogyne graminicola (rice root knot nematode). Stem nematodes like the Ditylenchus angustus infect floating, deepwater and rainfed lowland rice in the MRD (Nguyen and Prot, 1995). Other significant pests of rice in Vietnam include rice leaf folders, rice thrips and stem borers (Sebesvari et al., 2011). Meanwhile, common rice diseases include bacterial leaf blight, bakanae, black rot of grain, brown spot, leaf yellowing disease, neck blast, rice blast disease, root rot, sheath blight, sheath rot and stem rot (Pinnschmid et al., 1995; Sebesvari et al., 2011; Trung et al., 1995; Kim et al., 1995).
Between 2005 and 2008, rice production in the Mekong River Delta was severely reduced by outbreaks of brown planthopper and the associated virulent diseases. The problem was particularly severe in An Giang, Dong Thap and Tien Giang provinces, with more than 50 % of the cultivated areas affected (Berg and Tam, 2012). In 2009, the southern rice black streaked dwarf virus affected 19 provinces in northern Vietnam, including those in the Red River Delta. More than 80 % of the rice fields in Nam Dinh, Nghe An, Quang Ninh and Thai Binh provinces were infested, and yield was non-existent (Hoang et al., 2011). In short, an increase in pest and/or disease outbreaks will likely cause a reduction in rice yield (Fig. 6).
3.4 Global natural-hazard driver
Sea level rise (SLR)
Besides creating new environmental challenges, pre-existing threats to rice production and food security will be exacerbated by climate change. One of the effects of climate change is rising sea levels. Globally, the Intergovernmental Panel on Climate Change (IPCC) has projected sea levels to rise from a rate of 3.2 mm yr−1 from 1993 to 2010 to as much as 10 mm yr−1 or more by 2010 (Church et al., 2013). This may result in a 0.98 m increase in sea level by 2100 (Lassa et al., 2016). To quantify sea level rise locally, observations at tide gauges across Vietnam have recorded an average yearly increase of 3.3 mm from 1993–2014 (Hens et al., 2018). SLR may also increase the risk of storm surges (Hanh and Furukawa, 2007). In the Red River Delta, Neumann et al. (2015) found that sea level rise through 2050 could reduce the recurrence interval of the current 100-year storm surge with a 5 m height to once every 49 years. Inadequately constructed and poorly maintained dikes and embankments may be breached, resulting in saltwater flooding, which will damage rice growing areas and other properties (Hanh and Furukawa, 2007; Fig. 6).
Rising sea levels coupled with accelerated coastal subsidence caused by excessive groundwater extraction will cause large portions of the low-lying RRD and MRD to be inundated and flooded (Allison et al., 2017). This facilitates the infiltration of saltwater into groundwater aquifers, and this may increase salinity gradients in the MRD and RRD. In particular, salinity intrusion will worsen during the dry season. Approximately 1.8 million ha in the MRD is already affected by dry-season salinity, of which 1.3 million ha is affected by salinity levels above 5 g L−1 (Lassa et al., 2016). This area is predicted to increase to 2.2 million ha with rising sea levels. Meanwhile, in the northeastern part of the RRD, the 1 % salinity contour has migrated landwards by 4 to 10 km (Hanh and Furukawa, 2007). Increased soil salinization leads to a loss of land available for rice production (Fig. 6). Though there are already sea dikes and saline water intrusion sluices in both the MRD and RRD to reduce incursions of seawater (Braun et al., 2018; Tuong et al., 2003), they may be inadequate if they are not well maintained and upgraded.
4.1 Untangling complexity
Relevant information on the different drivers and environmental processes affecting rice production in Vietnam is fragmented in a range of academic and non-academic sources (Bosch et al., 2007), making it difficult for policymakers and managers to have a good overview of the reinforcing and interdependent processes and issues affecting food security in Vietnam. Using a systems-thinking approach, we amalgamated the various drivers and created flow diagrams to consider how rice productivity can be positively or negatively impacted by the various drivers and environmental processes (Figs. 4 and 6). Rice growing areas are negatively affected by the expansion of aquaculture and alternative crops and urban expansion. But with agricultural intensification facilitated by high agrochemical inputs, it was possible to maximize rice yields in spite of a smaller growing area. However excessive agrochemical use affects rice quality and may have an opposite effect on the prevalence of pests and diseases. Next, anthropogenic developments meant to improve agricultural productivity or increase economic growth can create many unwanted environmental consequences. Dikes keep floodwaters and salt intrusion out, but there is a reduction in fertile silt deposits. Sand mining increases channel erosion and reduces the frequency of natural freshwater flooding. Similar to sand mining, upstream dams affect sediment accumulation as sediments are trapped in reservoirs upstream, but the impacts of upstream dams extend over a larger geographical area. Lastly, groundwater extraction causes land subsidence, saltwater intrusion and arsenic contamination, with negative feedbacks on rice growing areas and the quality of rice produced.
Natural hazards not only affect rice quality and quantity but may also amplify some of the problems created by human activities; for instance, typhoons and sea level rise may induce saltwater flooding and aggravate salinity intrusion. Conversely, droughts also worsen the extent of salinity intrusion but due to a lack of fresh water. Overall, a substantial reduction in sediment from sand mining and upstream dams, coupled with the process of land subsidence from groundwater extraction and rising sea levels, will potentially reduce rice growing areas in the future. Besides sea level rise, climate change may also exacerbate the effects of natural hazards by increasing the frequency and severity of natural disasters (cf. Hausfather et al., 2017; Grosjean et al., 2016; Terry et al., 2012). As such, the problems such as excessive saltwater flooding and saltwater intrusion may intensify.
The use of flow diagrams provides a visual overview of the key anthropogenic drivers and natural hazards that affect rice production, but we caution that the Red River Delta and the Mekong River Delta are vast and diverse regions, and there are differences in the ways each delta is affected by natural hazards and anthropogenic drivers. For example, high dikes and the associated problem of sediment exclusion are a problem unique to the Mekong River Delta (Chapman et al., 2017). Next, compared to the Mekong, the Red River has substantially fewer dams (361 vs. 105). In addition, typhoons are less common in the Mekong River Delta, and droughts occur less frequently in the Red River Delta.
Within each delta, typhoons tend to affect coastal provinces more than those farther inland. Similarly, arsenic contamination and saltwater intrusion are not an issue everywhere across the two deltas. A comparison study of arsenic pollution in the Mekong and Red River deltas showed that groundwater arsenic concentrations ranged from 1–845 µg L−1 in the MRD and from 1–3050 µg L−1 in the RRD. Hotspots with high arsenic concentrations were likely due to local geogenic conditions (Berg et al., 2007). For salinity intrusion, Kotera et al. (2005) measured salinity concentrations in river and canal water across four Mekong River Delta provinces and showed that the salinity levels ranged from 0.6 to 14.4 g L−1, while a localized study in the Nam Dinh Province in the RRD showed that salt concentration in river water was higher at the river mouth than in upstream locations. Hence, given the possibility of spatial variations within a large landscape, it is important for local conditions to be taken into consideration.
One limitation of our study is that it was not possible to include all the problems that can potentially affect rice cultivation in our flow diagrams. We acknowledge issues related to industrial pollution, which may reduce rice quality and rice productivity (Khai and Yabe, 2012, 2021; Huong et al., 2008). In spite of this, our study presents the major issues that are common in both deltas and describes how the issues and processes affecting rice production are interrelated and may operate at different scales. Additionally, a systems-thinking approach has allowed the multitude of drivers and environmental processes affecting rice production to be visualized and mapped in a manner that is easy to understand. As ameliorating problems require policymakers and managers to have a good grasp of the different factors and processes present, a method that considers all the different drivers and possible unintended consequences from the outset can avoid oversimplifying a problem and assuming a straightforward solution can be found (DeFries and Nagendra, 2017). For example, to solve the problem of a shrinking delta, the effects of (high) dikes, sand mining, upstream dams and groundwater extraction have to be considered. While typhoons may provide some fluvial sediment to offset a shrinking delta (Darby et al., 2016), the sediment load provided may not be sufficient to offset sediment loss from sand mining and upstream dams.
4.2 Adaptation and soft solutions
Recognizing the environmental challenges limiting agricultural production, farmers in both deltas have adapted and improvised. Instead of accepting their fate, farmers overcome high soil salinities by implementing measures such as replacing rice with salinity-tolerant crops; transiting to shrimp aquaculture; or turning to rice–shrimp farming, whereby rice is grown in the wet season, and shrimp is cultivated in the dry season. For those unable to switch from rice monoculture, farmers have sought to grow rice on higher ground, shift crop calendars, or dig additional ditches to drain saltwater and store fresh water. In addition, to prevent water storage ponds from becoming contaminated with saltwater, canvas sheets are placed on the soil surface to create a protective barrier (Tran et al., 2019; Nguyen et al., 2012). Similarly, farmers in peri-urban areas who are faced with shrinking agricultural lands have turned to practicing agricultural intensification and/or switched to planting high-value crops such as fruits and vegetables (Morton, 2020; van den Berg et al., 2003). In short, farmers are constantly experimenting, learning and sharing knowledge and experiences with other farmers to come up with solutions for overcoming environmental limitations (Tran et al., 2019; Nguyen et al., 2012).
Unfortunately, adaptations are not successful all the time. For example, the widespread conversion of paddy fields to shrimp ponds will increase soil salinity and reduce the availability of fresh water. Over time, neighbors who have not switched to aquaculture may be unable to plant rice and would have to seek alternative livelihoods (Nguyen et al., 2012). In addition, rice–shrimp systems are not problem-free as well. Leigh et al. (2017) found that environmental conditions in rice–shrimp systems were suboptimal and contributed to low yields and survival. Water temperature and salinity tended to be too high in the dry season and dissolved oxygen too low, causing most shrimps to die. For rice, the high soil salinity caused by having the aquaculture pond was a major limitation; in their study, only 3 out of 18 ponds produced a harvestable rice crop.
Apart from farmer-led initiatives related to crop and land-use change, integrated pest management (IPM) should also be adopted to reduce the use of pesticides to get rid of pests. Farmers who practice IPM use a combination of pest-resistant cultivars, fertilizer management and agronomic practices to increase the effects of predators and other naturally occurring biological control agents. For example, farmers can grow flowers, okra and beans along their paddy fields to attract bees and wasps that infest planthopper pests' eggs. With more natural predators around, pesticides are only used when necessary (Bottrell and Schoenly, 2012; Normile, 2013). Alternatively, rice–fish farming and duck–rice systems can also be implemented to provide a more economically and ecologically sustainable alternative to intensive rice monoculture (Berg and Tam, 2012; Men et al., 2002).
In rice–fish farming, farmers use minimal pesticide as it kills the fish and the natural predators of rice pests. Instead, fish help to control pests, and fish droppings keep the soil fertile. Upon maturity, the fish can be sold to increase the farmer's income by up to 30 % (Berg et al., 2017; Bosma et al., 2012). Ducks can also be reared in immature rice fields. Besides providing food, the ducks serve as biological controls for insects and weeds. Their droppings fertilize the soils, and their movement aerates the water to benefit the rice plants (Men et al., 2002, 2021). Men et al. (2002) showed that a duck–rice system in Can Tho Province in the Mekong eliminated the use of pesticides and halved the use of fertilizers, and the additional income from the sale of ducks increased farmers' incomes by 50 % to 150 %. Overall, the higher incomes and ecosystem services provided by the fish or ducks, coupled with reduced agrochemical use, benefit farmers.
Increasingly, there are calls to move away from three to two rice crops a year in the MRD. Instead of planting a third crop, floodwaters are allowed to enter the fields to replenish soil nutrients, wash away contaminants, kill pests and mitigate salinity intrusion. Fish, crabs and snails that arrive with the floodwaters can be collected for additional income. Triple-cropping of rice provides only a single ecosystem service, which is marketable rice. On the other hand, the integration of rice cropping with natural flooding creates a series of positive feedback mechanisms and ecosystem services that include providing natural pest control and facilitating nutrient cycling (Nikula, 2008; Tong, 2017). However, a study by Tran and Weger (2018) in An Giang Province revealed that despite official encouragement to move away from triple-cropping, most farmers have largely ignored the directive as they preferred to earn money from the additional rice crop. In addition, many of them felt that the benefits of flooding the land were minimal as upstream dams have drastically reduced fertile sediment and fish. Evidently, farmers are willing to make changes to their farming practices only if it benefits them.
To mitigate “wicked” environmental challenges, there is a need for holistic land-use planning and soft measures (e.g., implementing crop and land-use change) on top of hard engineering structures. Previously, management options to increase agricultural productivity and mitigate the threats posed by natural hazards were largely characterized by hard options such as the construction of dikes, sea walls and sluice gates. These were typically top-down projects spearheaded by the local government (Neumann et al., 2015; Smajgl et al., 2015). While highly visible engineering structures are easily constructed and generally effective, unwanted side effects may be created. For example, flooding and sediment exclusion were some problems that were inadvertently created due to the presence of high dikes. In the long term, (costly) maintenance is needed to maintain the functionality of engineered structures (Hoang et al., 2018; Neumann et al., 2015). In addition, during the pre-construction phase, natural vegetation may be cleared (Geist and Lambin, 2002). Adopting a systems-thinking approach would allow policymakers and managers to situate the range of mitigation measures within broader environmental processes. In doing so, a clearer view of the possibilities and challenges present in an era of widespread anthropogenic development and changing climates is provided.
The focus of this paper is on the impacts of land-use patterns and natural hazards on rice agriculture in the Mekong and Red River deltas in Vietnam. While we focused on rice agriculture, these two deltas, like many other deltas worldwide, are also major production hubs for fruits and vegetables (Day et al., 2016; Nhan and Cao, 2019). Hence, the natural hazards and anthropogenic factors listed will have an effect on other agricultural produce as well.
A key finding is that demand for aquaculture and alternative crops and urban expansion has diminished rice growing areas. The problem of shrinking agricultural land is ameliorated by agricultural intensification, which has increased land efficiency. However, widespread agrochemical use causes land and water pollution and reduces crop quality. In addition, anthropogenic developments such as dike construction can improve agricultural productivity but also create unintended environmental problems. Even human activities that are unrelated to agriculture such as sand mining, groundwater extraction and dam construction can reduce rice productivity. In addition, natural hazards not only affect rice quality and quantity but may also amplify some of the problems created by human activities; for instance, typhoons and sea level rise may induce saltwater flooding and worsen salinity intrusion. In the future, climate change may exacerbate the effects of natural hazards by increasing the frequency and severity of natural disasters. Therefore, the problems associated with some of the natural hazards such as excessive saltwater flooding and saltwater intrusion may increase in frequency and severity. In sum, the processes and issues affecting food security are multidimensional and interdependent, and we used a systems-thinking approach to develop a visual representation of the ways in which anthropogenic land-use factors and natural hazards can affect rice quantity and quality in the MRD and the RRD in Vietnam. We have also sought to define whether the anthropogenic or natural-hazard driver was a local, regional or global driver to highlight the scale at which each driver operates.
Our review focuses on food security in Vietnam's two deltas but can be applied to other contexts. The problems present in the two deltas in Vietnam are hardly unique. Across the world, deltas are global food production hubs that support large populations. Nearly half a billion people live in deltaic regions. Similar to the Mekong and Red River deltas, large tracts of deltaic wetlands in other countries have been reclaimed for agriculture, aquaculture, and urban and industrial land use. Resultantly, many deltas suffer from flooding, retreating shorelines due to upstream dams, pollution problems, and increasing land subsidence due to groundwater and mineral extraction. With climate change, rising sea levels will further threaten the viability of the deltaic landform (Chan et al., 2012, 2015; Day et al., 2016; Giosan et al., 2014; Syvitski et al., 2009).
Given that river deltas worldwide are highly stressed and degraded, a systems-thinking approach can provide a holistic overview of the “wicked problems” faced in each location and how the various environmental processes interact with each other. Although our study has focused on rice agriculture in the two deltas in Vietnam, the application of a systems-thinking approach to evaluate other pertinent phenomena in deltas elsewhere is a useful tool for understanding how human activities, natural hazards and climate change have compromised deltaic sustainability.
The papers used in this study are listed in the Supplement.
The supplement related to this article is available online at: https://doi.org/10.5194/nhess-21-1473-2021-supplement.
KW and JSH came up with the idea for this project. KW reviewed papers and wrote the manuscript with discussions and improvements from all co-authors. JSH, AD, PT, TTH and VDQ provided feedback on the analysis of data and helped in revisions of the manuscript. JSH provided financial support for this paper.
The authors declare that they have no conflict of interest.
We thank Khoi Dang Kim, Jose Ma Luis Pangalangan Montesclaros and Nghiem Thi Phuong Le for their advice. We greatly appreciate the constructive comments and detailed feedback by our reviewers.
This research has been supported by the Earth Observatory of Singapore (grant no. M4430263.B50.706022) via its funding from the National Research Foundation Singapore and the Singapore Ministry of Education under the Research Centres of Excellence initiative. This work comprises EOS contribution number 360.
This paper was edited by Animesh Gain and reviewed by James Terry and one anonymous referee.
Adamson, P. and Bird, J.: The Mekong: A drought-prone tropical environment?, Int. J. Water Resour. Dev., 26, 579–594, https://doi.org/10.1080/07900627.2010.519632, 2010.
Allison, M. A., Nittrouer, C. A., Ogston, A. S., Mullarney, J. C., and Nguyen, T. T.: Sedimentation and survival of the Mekong Delta. A case study of decreased sediment supply and accelerating rates of relative sea level rise, Oceanography, 30, 98–109, 2017.
Arias, M. E., Holtgrieve, G. W., Ngor, P. B., Dang, T. D., and Piman, T.: Maintaining perspective of ongoing environmental change in the Mekong floodplains, Curr. Opin. Environ. Sustainabil., 37, 1–7, https://doi.org/10.1016/j.cosust.2019.01.002, 2019.
Berg, H. and Tam, N. T.: Use of pesticides and attitude to pest management strategies among rice and rice-fish farmers in the Mekong Delta, Vietnam, Int. J. Pest Manage., 58, 153–164, https://doi.org/10.1080/09670874.2012.672776, 2012.
Berg, H., Ekman Söderholm, A., Söderström, A.-S., and Tam, N. T.: Recognizing wetland ecosystem services for sustainable rice farming in the Mekong Delta, Vietnam, Sustainabil. Sci., 12, 137–154, https://doi.org/10.1007/s11625-016-0409-x, 2017.
Berg, M., Stengel, C., Trang, P. T. K., Hung Viet, P., Sampson, M. L., Leng, M., Samreth, S., and Fredericks, D.: Magnitude of arsenic pollution in the Mekong and Red River Deltas – Cambodia and Vietnam, Sci. Total Environ., 372, 413–425, https://doi.org/10.1016/j.scitotenv.2006.09.010, 2007.
Binh, D. V., Kantoush, S., and Sumi, T.: Changes to long-term discharge and sediment loads in the Vietnamese Mekong Delta caused by upstream dams, Geomorphology, 353, 107011, https://doi.org/10.1016/j.geomorph.2019.107011, 2020.
Binh, D. V., Kantoush, S., Sumi, T., Mai, N. T. P., and Trung, L. V.: La Vinh: Study on the impacts of river-damming and climate change on the Mekong Delta of Vietnam, DPRI Annuals, 60B, 804–826, 2017.
Bosch, O. J. H., King, C. A., Herbohn, J. L., Russell, I. W., and Smith, C. S.: Getting the big picture in natural resource management – systems thinking as `method' for scientists, https://doi.org/10.1002/sres.818, 2007.
Bosma, R. H., Nhan, D. K., Udo, H. M. J., and Kaymak, U.: Factors affecting farmers' adoption of integrated rice–fish farming systems in the Mekong delta, Vietnam, Rev. Aquacult., 4, 178–190, https://doi.org/10.1111/j.1753-5131.2012.01069.x, 2012.
Bottrell, D. G. and Schoenly, K. G.: Resurrecting the ghost of green revolutions past: The brown planthopper as a recurring threat to high-yielding rice production in tropical Asia, J. Asia-Pacif. Entomol., 15, 122–140, https://doi.org/10.1016/j.aspen.2011.09.004, 2012.
Braun, G., Sebesvari, Z., Braun, M., Kruse, J., Amelung, W., An, N. T., and Renaud, F. G.: Does sea-dyke construction affect the spatial distribution of pesticides in agricultural soils? – A case study from the Red River Delta, Vietnam, Environ. Pollut., 243, 890–899, https://doi.org/10.1016/j.envpol.2018.09.050, 2018.
Bravard, J.-P. and Gaillot, M. G. E. S.: Geography of sand and gravel mining in the Lower Mekong River, EchoGéo, 26, 1–20, https://doi.org/10.4000/echogeo.13659, 2013.
Brunier, G., Anthony, E. J., Goichot, M., Provansal, M., and Dussouillez, P.: Recent morphological changes in the Mekong and Bassac river channels, Mekong delta: The marked impact of river-bed mining and implications for delta destabilisation, Geomorphology, 224, 177–191, https://doi.org/10.1016/j.geomorph.2014.07.009, 2014.
Ca, V. T., Vongvisessomjai, S., and Asaeda, T.: Study on Salinity Intrusion in the Red River Delta, Environ. Syst. Res., 22, 213–218, https://doi.org/10.2208/proer1988.22.213, 1994.
CCFSC: National report on disasters in Vietnam, Vietnam Central Committee for flood and storm control, in: World Conference on disaster reduction, 18–22 January 2005, Kobe-Hyogo, 2005.
Chan, F. K. S., Mitchell, G., Adekola, O., and McDonald, A.: Flood risk in Asia's urban mega-deltas: Drivers, impacts and response, Environ. Urbaniz. Asia, 3, 41–61, https://doi.org/10.1177/097542531200300103, 2012.
Chan, F. K. S., Friess, D. A., Terry, J. P., and Mitchell, G.: Climate change & flood risk: challenges for the coastal regions of East Asia, in: Routledge Handbook of East Asia and the Environment, edited by: Harris, P. G. and Land, G., Routledge, London, 367–384, 2015.
Chapman, A. and Darby, S.: Evaluating sustainable adaptation strategies for vulnerable mega-deltas using system dynamics modelling: Rice agriculture in the Mekong Delta's An Giang Province, Vietnam, Sci. Total Environ., 559, 326–338, https://doi.org/10.1016/j.scitotenv.2016.02.162, 2016.
Chapman, A., Darby, S., Tompkins, E., Hackney, C., Leyland, J., Van, P. D. T., Pham, T. V., Parsons, D., Aalto, R., and Nicholas, A.: Sustainable rice cultivation in the deep flooded zones of the Vietnamese Mekong Delta, Vietnam J. Sci. Techno. Eng., 59, 34–38, https://doi.org/10.31276/VJSTE.59(2).34, 2017.
Chapman, A. D., Darby, S. E., Hőng, H. M., Tompkins, E. L., and Van, T. P. D.: Adaptation and development trade-offs: fluvial sediment deposition and the sustainability of rice-cropping in An Giang Province, Mekong Delta, Climatic Change, 137, 593–608, https://doi.org/10.1007/s10584-016-1684-3, 2016.
Chinh, G.: Illegal sand mining rampant in Vietnam's Red River, authorities do nothing, available at: https://e.vnexpress.net/news/news/illegal-sand-mining-rampant-in-vietnam-s-red-river-authorities, last access: 19 November 2018.
Church, J. A., Clark, P. U., Cazenave, A., Gregory, J. M., Jevrejeva, S., Levermann, A., Merrifield, M. A., Milne, G. A., Nerem, R. S., Nunn, P. D., Payne, A. J., Pfeffer, W. T., Stammer, D., and Unnikrishnan, A. S.: Sea level change, in: Climate change 2013: The physical science basis, Contribution of Working Group I to the Fifth Assessment Report of the Intergovernmental Panel on Climate Change, edited by: Stocker, T. F., Qin, D., Plattner, G.-K., Tignor, M., Allen, S. K., Boschung, J., Nauels, A., Xia, Y., Bex, V., and Midgley, P. M., Cambridge University Press, Cambridge, UK and New York, USA, 1137–1216, 2013.
Cosslett, T. L. and Cosslett, P. D.: Rice cultivation, production, and consumption in Mainland Southeast Asian Countries: Cambodia, Laos, Thailand, and Vietnam, in: Sustainable development of rice and water resources in Mainland Southeast Asia and Mekong River Basin, Springer, Singapore, 29–53, 2018.
Darby, S. E., Hackney, C. R., Leyland, J., Kummu, M., Lauri, H., Parsons, D. R., Best, J. L., Nicholas, A. P., and Aalto, R.: Fluvial sediment supply to a mega-delta reduced by shifting tropical-cyclone activity, Nature, 539, 276–279, https://doi.org/10.1038/nature19809, 2016.
Day, J. W., Agboola, J., Chen, Z., D'Elia, C., Forbes, D. L., Giosan, L., Kemp, P., Kuenzer, C., Lane, R. R., Ramachandran, R., Syvitski, J., and Yañez-Arancibia, A.: Approaches to defining deltaic sustainability in the 21st century, Estuar. Coast. Shelf Sci., 183, 275–291, https://doi.org/10.1016/j.ecss.2016.06.018, 2016.
DeFries, R. and Nagendra, H.: Ecosystem management as a wicked problem, Science, 356, 265–270, https://doi.org/10.1126/science.aal1950, 2017.
Digital Typhoon: Typhoon images and information, available at: http://agora.ex.nii.ac.jp/digital-typhoon/index.html.en, last access: 6 April 2021.
Drebold, H.: Food security and rapid urbanization. A case study of urban agriculture in Hanoi, Institute of Natural Science, Environmental Studies and Technology, Södertörns University, Stockholm, 2017.
Duc, D. M., Nhuan, M. T., and Ngoi, C. V.: An analysis of coastal erosion in the tropical rapid accretion delta of the Red River, Vietnam, J. Asian Earth Sci., 43, 98–109, https://doi.org/10.1016/j.jseaes.2011.08.014, 2012.
EEPSEA: The winners and losers of the floods in the Mekong Delta – A study from Vietnam, EEPSEA Policy Brief No. 2011-PB10, EEPSEA, Singapore, 2011.
Erban, L. E., Gorelick, S. M., Zebker, H. A., and Fendorf, S.: Release of arsenic to deep groundwater in the Mekong Delta, Vietnam, linked to pumping-induced land subsidence, P. Natl. Acad. Sci. USA, 110, 13751–13756, https://doi.org/10.1073/pnas.1300503110, 2013.
Fendorf, S., Michael, H. A., and van Geen, A.: Spatial and temporal variations of groundwater arsenic in South and Southeast Asia, Science, 328, 1123–1127, https://doi.org/10.1126/science.1172974, 2010.
Garschagen, M., Renaud, F. G., and Birkmann, J.: Dynamic Resilience of Peri-Urban Agriculturalists in the Mekong Delta Under Pressures of Socio-Economic Transformation and Climate Change, in: Environmental Change and Agricultural Sustainability in the Mekong Delta, edited by: Stewart, M. A., and Coclanis, P. A., Springer Netherlands, Dordrecht, 141–163, 2011.
Geist, H. J. and Lambin, E. F.: Proximate causes and underlying driving forces of tropical deforestation: Tropical forests are disappearing as the result of many pressures, both local and regional, acting in various combinations in different geographical locations, BioScience, 52, 143–150, https://doi.org/10.1641/0006-3568(2002)052[0143:PCAUDF]2.0.CO;2, 2002.
General Statistics Office: Statistical Yearbook of Vietnam 2018, Statistical Publishing House, Hanoi, 2018.
General Statistics Office of Vietnam: Agriculture, forestry and fishery, available at: https://www.gso.gov.vn/en/agriculture-forestry-and-fishery/, last access: 19 November 2020.
Giosan, L., Syvitski, J., Constantinescu, S., and Day, J.: Climate change: protect the world's deltas, Nat. News, 516, 31–33, https://doi.org/10.1038/516031a, 2014.
Grosjean, G., Monteils, F., Hamilton, S. D., Blaustein-Rejto, D., Gatto, M., Talsma, T., Bourgoin, C., Sebastian, L. S., Catacutan, D., Mulia, R., Bui, Y., Tran, D. N., Nguyen, K. G., Pham, M. T., Lan, L. N., and Läderach, P.: Increasing resilience to droughts in Vietnam; The role of forests, agroforests and climate smart agriculture, CCAFS-CIAT-UN-REDD Position Paper n. 1, CCAFS-CIAT-UN-REDD, Hanoi, 2016.
GRSP – Global Rice Science Partnership: Rice almanac, 4th Edn., International Rice Research Institute, Los Baños, 2013.
Hai, T. T. B.: Fruit production and business in the Mekong Delta, available at: http://jabes.ueh.edu.vn/Content/ArticleFiles/c23bab76-b2ea-c582-7df8-bf4eea1add2d/JED_2002_226.pdf, last access: 6 May 2021.
Hanh, P. T. T. and Furukawa, M.: Impact of sea level rise on coastal zone of Vietnam, Bull. Facult. Sci. Univers. Ryukyu, 84, 45–49, 2007.
Hausfather, Z., Cowtan, K., Clarke, D. C., Jacobs, P., Richardson, M., and Rohde, R.: Assessing recent warming using instrumentally homogeneous sea surface temperature records, Sci. Adv., 3, 1–13, https://doi.org/10.1126/sciadv.1601207, 2017.
Hens, L., Thinh, N. A., Hanh, T. H., Cuong, N. S., Lan, T. D., Thanh, N. V., and Le, D. T.: Sea-level rise and resilience in Vietnam and the Asia-Pacific: A synthesis, Vietnam J. Earth Sci., 40, 126–152, https://doi.org/10.15625/0866-7187/40/2/11107, 2018.
Hoa, L. T. V., Shigeko, H., Nhan, N. H., and Cong, T. T.: Infrastructure effects on floods in the Mekong River Delta in Vietnam, Hydrol. Process., 22, 1359–1372, https://doi.org/10.1002/hyp.6945, 2008.
Hoang, A. T., Zhang, H.-M., Yang, J., Chen, J.-P., Hébrard, E., Zhou, G.-H., Vinh, V. N., and Cheng, J.-A.: Identification, characterization, and distribution of southern rice black-streaked dwarf virus in Vietnam, Plant Disease, 95, 1063–1069, https://doi.org/10.1094/pdis-07-10-0535, 2011.
Hoang, L. P., Biesbroek, R., Tri, V. P. D., Kummu, M., van Vliet, M. T. H., Leemans, R., Kabat, P., and Ludwig, F.: Managing flood risks in the Mekong Delta: How to address emerging challenges under climate change and socioeconomic developments, Ambio, 47, 635–649, https://doi.org/10.1007/s13280-017-1009-4, 2018.
Hoang, V. and Tran, K. T.: Comparative advantages of alternative crops: A comparison study in Ben Tre, Mekong Delta, Vietnam, AGRIS on-line Papers in Economics and Informatics, 11, 35–47, 2019.
Howie, C.: High dykes in the Mekong Delta in Vietnam bring social gains and environmental pains, Aquacult. News, 32, 15–17, 2005.
Hung, N. N., Delgado, J. M., Tri, V. K., Hung, L. M., Merz, B., Bárdossy, A., and Apel, H.: Floodplain hydrology of the Mekong Delta, Vietnam, Hydrol. Process., 26, 674–686, https://doi.org/10.1002/hyp.8183, 2012.
Huong, N. T. L., Ohtsubo, M., Li, L., Higashi, T., Kanayama, M., and Nakano, A.: Heavy metal contamination of soil and rice in a wastewater-irrigated paddy field in a suburban area of Hanoi, Vietnam, Clay Sci., 13, 205–215, https://doi.org/10.11362/jcssjclayscience1960.13.205, 2008.
Huong, P. T. T., Everaarts, A. P., Neeteson, J. J., and Struik, P. C.: Vegetable production in the Red River Delta of Vietnam. I. Opportunities and constraints, Wageningen J. Life Sci., 67, 27–36, https://doi.org/10.1016/j.njas.2013.09.002, 2013.
Imamura, F. and Van To, D.: Flood and typhoon disasters in Viet Nam in the half Century since 1950, Nat. Hazards, 15, 71–87, https://doi.org/10.1023/A:1007923910887, 1997.
International Federation of the Red Cross and Red Crescent Society: Viet Nam: Typhoons revised appeal no. MDRVN001 – 22 December 2006, available at: https://reliefweb.int/report/viet-nam/viet-nam-typhoons-revised-appeal-no-mdrvn001-22-dec-2006 (last access: 20 November 2018), 2006.
Jordan, C., Tiede, J., Lojek, O., Visscher, J., Apel, H., Nguyen, H. Q., Quang, C. N. X., and Schlurmann, T.: Sand mining in the Mekong Delta revisited – current scales of local sediment deficits, Scient. Rep., 9, 17823, https://doi.org/10.1038/s41598-019-53804-z, 2019.
Khai, H. V. and Yabe, M.: Rice Yield Loss Due to Industrial Water Pollution in Vietnam, J. US-China Publ. Admin., 9, 248–256, 2012.
Khai, H. V. and Yabe, M.: Impact of industrial water pollution on rice production in Vietnam, available at: https://www.intechopen.com/books/international-perspectives-on-water-quality-management-and-pollutant-control/impact-of-industrial-water-pollution -on-rice-production-in-vietnam, last access: 6 May 2021.
Kim, P. V., Cu, R. M., Teng, P. S., Cassman, K. G., Mew, T. W., Nga, T. T., Ve, N. B., and Bien, P. V.: Relationships between rice intensification, rice plant nutrition, and leaf-yellowing disease in the Mekong River Delta. Vietnam and IRRI: A partnership in rice research, in: Proceedings of a conference, 4–7 May 1994, Hanoi, Vietnam, 211–216, 1995.
Kondolf, G. M., Rubin, Z. K., and Minear, J. T.: Dams on the Mekong: Cumulative sediment starvation, Water Resour. Res., 50, 5158–5169, https://doi.org/10.1002/2013WR014651, 2014.
Kondolf, G. M., Schmitt, R. J. P., Carling, P., Darby, S., Arias, M., Bizzi, S., Castelletti, A., Cochrane, T. A., Gibson, S., Kummu, M., Oeurng, C., Rubin, Z., and Wild, T.: Changing sediment budget of the Mekong: Cumulative threats and management strategies for a large river basin, Sci. Total Environ., 625, 114–134, https://doi.org/10.1016/j.scitotenv.2017.11.361, 2018.
Kotera, A., Nawata, E., Thao, L. V., Vuong, N. V., and Sakuratani, T.: Effect of submergence on rice yield in the Red River Delta, Vietnam, Japan. J. Trop. Agricult., 49, 197–206, https://doi.org/10.11248/jsta1957.49.197, 2005.
Kotera, A., Sakamoto, T., Nguyen, D. K., and Yokozawa, M.: Regional consequences of seawater intrusion on rice productivity and land use in coastal area of the Mekong River Delta, Japan Agricult. Res. Quart., 42, 267–274, https://doi.org/10.6090/jarq.42.267, 2008.
Lan, N. X. and Giao, N. T.: Arsenic dynamics within rice production systems in the Mekong Delta, Viet Nam, Imper. J. Interdisciplin. Res., 3, 412–420, 2017.
Larson, M., Hung, N. M., Hanson, H., Sundström, A., and Södervall, E.: 2 – Impacts of typhoons on the Vietnamese coastline: A case study of Hai Hau Beach and Ly Hoa Beach, in: Coastal Disasters and Climate Change in Vietnam, edited by: Thao, N. D., Takagi, H., and Esteban, M., Elsevier, Oxford, 17–42, 2014.
Lassa, J. A., Lai, A. Y.-H., and Goh, T.: Climate extremes: an observation and projection of its impacts on food production in ASEAN, Nat. Hazards, 84, 19–33, https://doi.org/10.1007/s11069-015-2081-3, 2016.
Le, T. N., Bregt, A. K., van Halsema, G. E., Hellegers, P. J. G. J., and Nguyen, L.-D.: Interplay between land-use dynamics and changes in hydrological regime in the Vietnamese Mekong Delta, Land Use Policy, 73, 269–280, https://doi.org/10.1016/j.landusepol.2018.01.030, 2018.
Le, T. V. H., Nguyen, H. N., Wolanski, E., Tran, T. C., and Haruyama, S.: The combined impact on the flooding in Vietnam's Mekong River delta of local man-made structures, sea level rise, and dams upstream in the river catchment, Estuar. Coast. Shelf Sci., 71, 110–116, https://doi.org/10.1016/j.ecss.2006.08.021, 2007.
Leigh, C., Hiep, L. H., Stewart-Koster, B., Vien, D. M., Condon, J., Sang, N. V., Sammut, J., and Burford, M. A.: Concurrent rice-shrimp-crab farming systems in the Mekong Delta: Are conditions (sub) optimal for crop production and survival?, Aquacult. Res., 48, 5251–5262, https://doi.org/10.1111/are.13338, 2017.
Li, Z., Saito, Y., Matsumoto, E., Wang, Y., Tanabe, S., and Lan Vu, Q.: Climate change and human impact on the Song Hong (Red River) Delta, Vietnam, during the Holocene, Quatern. Int., 144, 4–28, https://doi.org/10.1016/j.quaint.2005.05.008, 2006.
Lim, C. L., Prescott, G. W., De Alban, J. D. T., Ziegler, A. D., and Webb, E. L.: Untangling the proximate causes and underlying drivers of deforestation and forest degradation in Myanmar, Conserv. Biol., 31, 1362–1372, https://doi.org/10.1111/cobi.12984, 2017.
Liu, S. a., Li, X., Chen, D., Duan, Y., Ji, H., Zhang, L., Chai, Q., and Hu, X.: Understanding land use/land cover dynamics and impacts of human activities in the Mekong Delta over the last 40 years, Global Ecol. Conserv., 22, e00991, https://doi.org/10.1016/j.gecco.2020.e00991, 2020.
Luu, T. L.: Remarks on the current quality of groundwater in Vietnam, Environ. Sci. Pollut. Res., 26, 1163–1169, https://doi.org/10.1007/s11356-017-9631-z, 2019.
Mai, C. V., Stive, M. J. F., and Van Gelder, P. H. A. J. M.: Coastal protection strategies for the Red River Delta, J. Coast. Res., 25, 105–116, https://doi.org/10.2112/07-0888.1, 2009.
Mainuddin, M., Kirby, M., and Hoanh, C. T.: Adaptation to climate change for food security in the lower Mekong Basin, Food Secur., 3, 433–450, https://doi.org/10.1007/s12571-011-0154-z, 2011.
Manh, N. V., Dung, N. V., Hung, N. N., Kummu, M., Merz, B., and Apel, H.: Future sediment dynamics in the Mekong Delta floodplains: Impacts of hydropower development, climate change and sea level rise, Global Planet. Change, 127, 22–33, https://doi.org/10.1016/j.gloplacha.2015.01.001, 2015.
Masutomi, Y., Iizumi, T., Takahashi, K., and Yokozawa, M.: Estimation of the damage area due to tropical cyclones using fragility curves for paddy rice in Japan, Environ. Res. Lett., 7, 014020, https://doi.org/10.1088/1748-9326/7/1/014020, 2012.
Mathers, S. and Zalasiewicz, J.: Holocene sedimentary architecture of the Red River Delta, Vietnam, J. Coast. Res., 15, 314–325, 1999.
Matsukawa-Nakata, M., Nguyen, H. C., and Kobori, Y.: Insecticide application and its effect on the density of rice planthoppers, Nilaparvata lugens and Sogatella furcifera, in paddy fields in the Red River Delta, Vietnam, J. Pesticide Sci., 44, 129–135, https://doi.org/10.1584/jpestics.D18-080, 2019.
McElwee, P., Nghiem, T., Le, H., and Vu, H.: Flood vulnerability among rural households in the Red River Delta of Vietnam: implications for future climate change risk and adaptation, Nat. Hazards, 86, 465–492, https://doi.org/10.1007/s11069-016-2701-6, 2017.
Meharg, A. A., Williams, P. N., Adomako, E., Lawgali, Y. Y., Deacon, C., Villada, A., Cambell, R. C. J., Sun, G., Zhu, Y.-G., Feldmann, J., Raab, A., Zhao, F.-J., Islam, R., Hossain, S., and Yanai, J.: Geographical variation in total and inorganic arsenic content of polished (white) rice, Environ. Sci. Technol., 43, 1612–1617, https://doi.org/10.1021/es802612a, 2009.
Men, B. X., Tinh, T. K., Preston, T. R., Ogle, R. B., and Lindberg, J. E.: Use of local ducklings to control insect pests and weeds in the growing rice field, available at: http://www.fao.org/ag/AGA/agap/FRG/FEEDback/lrrd/lrrd11/2/men112.htm, last access: 6 May 2021.
Men, B. X., Ogle, R. B., and Lindberg, J. E.: Studies on integrated duck-rice systems in the Mekong Delta of Vietnam, J. Sustain. Agricult., 20, 27–40, https://doi.org/10.1300/J064v20n01_05, 2002.
Minderhoud, P. S. J., Erkens, G., Pham, V. H., Bui, V. T., Erban, L., Kooi, H., and Stouthamer, E.: Impacts of 25 years of groundwater extraction on subsidence in the Mekong delta, Vietnam, Environ. Res. Lett., 12, 064006, https://doi.org/10.1088/1748-9326/aa7146, 2017.
Minderhoud, P. S. J., Coumou, L., Erban, L. E., Middelkoop, H., Stouthamer, E., and Addink, E. A.: The relation between land use and subsidence in the Vietnamese Mekong delta, Sci. Total Environ.t, 634, 715–726, https://doi.org/10.1016/j.scitotenv.2018.03.372, 2018.
Morton, L. W.: Working toward sustainable agricultural intensification in the Red River Delta of Vietnam, J. Soil Water Conserv., 75, 109A–116A, https://doi.org/10.2489/jswc.2020.0304A, 2020.
Nelson, A. and Gumma, M. K.: A map of lowland rice extent in the major rice growing countries of Asia, available at: http://irri.org/our-work/research/policy-and-markets/mapping (last access: 19 November 2018), 2015.
Neumann, J. E., Emanuel, K. A., Ravela, S., Ludwig, L. C., and Verly, C.: Risks of coastal storm surge and the effect of sea level rise in the Red River Delta, Vietnam, Sustainability, 7, 6553–6572, 2015.
Newell, B. and Wasson, R.: Social system vs solar system: Why policy makers need history. Conflict and cooperation related to international water resources: Historical perspectives, in: Selected papers of the International Water History Association's Conference on The Role of Water in History and Development, A contribution to IHP-VI, Theme 4 “Water and Society” and the UNESCO/Green Cross International Initiative from Potential Conflict to Co-operation Potential: Water for Peace, a sub-component of the World Water Assessment Programme, 10–12 August 2001, Bergen, Norway, 2002.
Nguyen, H. N. V., Trung, K., and Nguyen, X. N.: Flooding the Mekong River Delta, Viet Nam, Human Development Report 2007/2008, Fighting climate change: Human solidarity in a divided world, Human Development Report Office Occassional Paper, available at: https://citeseerx.ist.psu.edu/viewdoc/download?doi=10.1.1.422.5648&rep=rep1&type=pdf (last access: 6 May 2021), 2007.
Nguyen, K.-A., Liou, Y.-A., and Terry, J. P.: Vulnerability of Vietnam to typhoons: A spatial assessment based on hazards, exposure and adaptive capacity, Sci. Total Environ., 682, 31–46, https://doi.org/10.1016/j.scitotenv.2019.04.069, 2019.
Nguyen, K. V. and James, H.: Measuring household resilience to floods: A case study in the Vietnamese Mekong River Delta, Ecol. Soc., 18, https://doi.org/10.5751/ES-05427-180313, 2013.
Nguyen, M. T., Renaud, F. G., and Sebesvari, Z.: Drivers of change and adaptation pathways of agricultural systems facing increased salinity intrusion in coastal areas of the Mekong and Red River deltas in Vietnam, Environ. Sci. Policy, 92, 331–348, https://doi.org/10.1016/j.envsci.2018.10.016, 2019.
Nguyen, N. A.: Historic drought and salinity intrusion in the Mekong Delta in 2016: Lessons learned and response solutions, Vietnam J. Sci. Technol. Eng., 60, 93–96, 2017.
Nguyen, Q. H. and Vo, H. T.: Paper 3: Ensuring a sustainable tropical fruit industry in the midst of climate change: The Vietnam story, in: 2017 International Symposium on Tropical Fruits, 23–25 October 2017, Nadi, Fiji, 2017.
Nguyen, T. T. C. and Prot, J. C.: Nematode parasites of deepwater and irrigated rice in the Mekong River Delta. Vietnam and IRRI: A partnership in rice research, in: Proceedings of a conference, 4–7 May 1994, Hanoi, Vietnam, 251–260, 1995.
Nguyen, V. K., Tran, A. T., Tran, V. H., Vo, D. T., Le, T., Tran, X., and Nguyen, V.: Climate change: Local perception, impacts and adaptation of agrarian communities in the coastal provinces of the Mekong River Delta, Vietnam, Water and Climate: Policy Implementation Challenges, in: Proceedings of the 2nd Practical Responses to Climate Change Conference, Canberra, available at: https://www.researchgate.net/publication/327357956_Climate_Change_Local_Perception_Impacts_and_Adaptation_of_Agrarian_Communities_in_the_Coastal_Provinces_of_the_Mekong_River_Delta_Vietnam (last access: 6 May 2021), 2012.
Nguyen, Y. T. B., Kamoshita, A., Dinh, V. T. H., Matsuda, H., and Kurokura, H.: Salinity intrusion and rice production in Red River Delta under changing climate conditions, Paddy Water Environ., 15, 37–48, https://doi.org/10.1007/s10333-016-0526-2, 2017.
Nhan, N. H., and Cao, N. B.: Chapter 19 – Damming the Mekong: Impacts in Vietnam and solutions, in: Coasts and Estuaries, edited by: Wolanski, E., Day, J. W., Elliott, M., and Ramachandran, R., Elsevier, 321–340, 2019.
Nhân Dân: Typhoon Kalmaegi claimed 12 lives in northern region, available at: http://en.nhandan.com.vn/society/item/2802302-typhoon-kalmaegi-claimed-12-lives-in-northern-region.html (last access: 20 November 2018), 2014.
Nikula, J.: Is harm and destruction all that floods bring?, Modern myths of the Mekong – a critical review of water and development concepts, principles and policies, Water & Development Publications, Helsinki University of Technology, Helsinki, Finland, 27–38, 2008.
Normile, D.: Vietnam turns back a `tsunami of pesticides', Science, 341, 737–738, https://doi.org/10.1126/science.341.6147.737, 2013.
Overland, M. A.: Vietnam feels the heat of a 100 year drought, available at: http://content.time.com/time/world/article/0,8599,1969630,00.html (last access: 20 November 2018), 2010.
Pandey, A., Kumar, A., Pandey, D., and Thongbam, P.: Rice quality under water stress, Indian J. Adv. Plant Res., 1, 23–26, 2014.
Park, E., Ho, H. L., Tran, D. D., Yang, X., Alcantara, E., Merino, E., and Son, V. H.: Dramatic decrease of flood frequency in the Mekong Delta due to river-bed mining and dyke construction, Sci. Total Environ., 723, 138066, https://doi.org/10.1016/j.scitotenv.2020.138066, 2020.
Pham, H. V., Pham, T. K. T., and Dao, V. N.: Arsenic contamination in groundwater in the Red river delta, Vietnam – a review, Vietnam J. Sci. Technol. Eng., 60, 23–27, 2018.
Pham, T. M. T., Raghavan, V., and Pawar, N. J.: Urban expansion of Can Tho city, Vietnam: A study based on multi-temporal satellite images, Geoinformatics, 21, 147–160, https://doi.org/10.6010/geoinformatics.21.147, 2010.
Pham, V. C., Pham, T.-T.-H., Tong, T. H. A., Nguyen, T. T. H., and Pham, N. H.: The conversion of agricultural land in the peri-urban areas of Hanoi (Vietnam): patterns in space and time, J. Land Use Sci., 10, 224–242, https://doi.org/10.1080/1747423X.2014.884643, 2015.
Pham, V. H. T., Febriamansyah, R., Afrizal, A., and Anh Tran, T.: Impact of saline intrusion on social and economic livelihoods of farmers in the Vietnam Mekong Delta, Int. J. Agricult. Sci., 1, 75–84, 2017.
Pham, V. H. T., Febriamansyah, R., and Thong, T. A.: Government intervention and farmers' adaptation to saline intrusion: A case study in the Vietnamese Mekong Delta, Int. J. Adv. Sci. Eng. Inform. Technol., 8, 2142–2148, https://doi.org/10.18517/ijaseit.8.5.7090, 2018.
Phuong, T. D., Chuong, P. V., Khiem, D. T., and Kokot, S.: Elemental content of Vietnamese rice Part 1. Sampling, analysis and comparison with previous studies, Analyst, 124, 553–560, https://doi.org/10.1039/A808796B, 1999.
Pilarczyk, K. W. and Nguyen, S. N.: Experience and practices on flood control in Vietnam, Water Int., 30, 114–122, https://doi.org/10.1080/02508060508691843, 2005.
Pinnschmidt, H. O., Long, N. D., Viet, T. T., Don, L. D., and Teng, P. S.: Characterization of pests, pest losses, and production patterns in a rainfed lowland rice of the Mekong River Delta. Vietnam and IRRI: A partnership in rice research, in: Proceedings of a conference, 4–7 May 1994, Hanoi, Vietnam, 223–240, 1995.
Preston, N., Brennan, D., and Clayton, H.: An overview of the project research, in: Rice–shrimp farming in the Mekong Delta: Biophysical and socioeconomic issues, ACIAR Technical Reports No. 52e, edited by: Preston, N. and Clayton, H., ACIAR, Canberra, 2003.
Quynh, D. N., Ninh, P. V., Manh, D. V., and Lien, N. T. V.: The typhoon surges in Vietnam: the regime characteristics and prediction, in: Proceedings of the Fifth Asian Science and Technology Week, Hanoi, 1998.
Rahman, M. A. and Hasegawa, H.: High levels of inorganic arsenic in rice in areas where arsenic-contaminated water is used for irrigation and cooking, Sci. Total Environ., 409, 4645–4655, https://doi.org/10.1016/j.scitotenv.2011.07.068, 2011.
Razzaq, A., Ali, A., Safdar, L. B., Zafar, M. M., Rui, Y., Shakeel, A., Shaukat, A., Ashraf, M., Gong, W., and Yuan, Y.: Salt stress induces physiochemical alterations in rice grain composition and quality, J. Food Sci., 85, 14–20, https://doi.org/10.1111/1750-3841.14983, 2020.
Rittel, H. W. J. and Webber, M. M.: Dilemmas in a general theory of planning, Policy Sci., 4, 155–169, https://doi.org/10.1007/BF01405730, 1973.
Robert, A.: A river in peril: Human activities and environmental impacts on the Lower Mekong River and its Delta, Environment: Sci. Policy Sustain. Dev., 59, 30–40, https://doi.org/10.1080/00139157.2017.1374794, 2017.
Rubin, Z. K., Kondolf, G. M., and Carling, P. A.: Anticipated geomorphic impacts from Mekong basin dam construction, Int. J. River Basin Manage., 13, 105–121, https://doi.org/10.1080/15715124.2014.981193, 2015.
Schmitt, R. J. P., Rubin, Z., and Kondolf, G. M.: Losing ground – scenarios of land loss as consequence of shifting sediment budgets in the Mekong Delta, Geomorphology, 294, 58–69, https://doi.org/10.1016/j.geomorph.2017.04.029, 2017.
Schneider, P., and Asch, F.: Rice production and food security in Asian Mega deltas – A review on characteristics, vulnerabilities and agricultural adaptation options to cope with climate change, J. Agron. Crop Sci., 206, 491–503, https://doi.org/10.1111/jac.12415, 2020.
Sebesvari, Z., Le, T. T. H., and Renaud, F. G.: Climate change adaptation and agrichemicals in the Mekong Delta, Vietnam, in: Environmental change and agricultural sustainability in the Mekong Delta, edited by: Stewart, M. A. and Coclanis, P. A., Springer Netherlands, Dordrecht, 219–239, 2011.
Seto, K. C.: Exploring the dynamics of migration to mega-delta cities in Asia and Africa: Contemporary drivers and future scenarios, Global Environ. Change, 21, S94–S107, https://doi.org/10.1016/j.gloenvcha.2011.08.005, 2011.
Smajgl, A., Toan, T. Q., Nhan, D. K., Ward, J., Trung, N. H., Tri, L. Q., Tri, V. P. D., and Vu, P. T.: Responding to rising sea levels in the Mekong Delta, Nat. Clim. Change, 5, 167–174, https://doi.org/10.1038/nclimate2469, 2015.
Syvitski, J. P. M. and Saito, Y.: Morphodynamics of deltas under the influence of humans, Global Planet. Change, 57, 261–282, https://doi.org/10.1016/j.gloplacha.2006.12.001, 2007.
Syvitski, J. P. M., Kettner, A. J., Overeem, I., Hutton, E. W. H., Hannon, M. T., Brakenridge, G. R., Day, J., Vörösmarty, C., Saito, Y., Giosan, L., and Nicholls, R. J.: Sinking deltas due to human activities, Nat. Geosci., 2, 681–686, https://doi.org/10.1038/ngeo629, 2009.
Takagi, H., Nguyen, D. T., Esteban, M., Tran, T. T., Knaepen, H. L., Mikami, T., and Yamamoto, L.: Coastal disaster risk in Southern Vietnam – the problems of coastal development and the need for better coastal planning, Background paper, Global Assessment Report on Disaster Risk Reduction 2013, The United Nations Office for Disaster Risk Reduction, Geneva, 2013.
Terry, J. P., Winspear, N., and Cuong, T. Q.: The `terrific Tongking typhoon' of October 1881 – implications for the Red River Delta (northern Vietnam) in modern times, Weather, 67, 72–75, https://doi.org/10.1002/wea.882, 2012.
Tessler, Z. D., Vörösmarty, C. J., Grossberg, M., Gladkova, I., Aizenman, H., Syvitski, J. P. M., and Foufoula-Georgiou, E.: Profiling risk and sustainability in coastal deltas of the world, Science, 349, 638–643, https://doi.org/10.1126/science.aab3574, 2015.
Tong, Y. D.: Rice intensive cropping and balanced cropping in the Mekong Delta, Vietnam – economic and ecological considerations, Ecol. Econ., 132, 205–212, https://doi.org/10.1016/j.ecolecon.2016.10.013, 2017.
Tran, D. D. and Weger, J.: Barriers to implementing irrigation and drainage policies in An Giang province, Mekong Delta, Vietnam, Irrig. Drain., 67, 81–95, https://doi.org/10.1002/ird.2172, 2018.
Tran, D. D., van Halsema, G., Hellegers, P. J. G. J., Phi Hoang, L., Quang Tran, T., Kummu, M., and Ludwig, F.: Assessing impacts of dike construction on the flood dynamics of the Mekong Delta, Hydrol. Earth Syst. Sci., 22, 1875–1896, https://doi.org/10.5194/hess-22-1875-2018, 2018.
Tran, T. A., Nguyen, T. H., and Vo, T. T.: Adaptation to flood and salinity environments in the Vietnamese Mekong Delta: Empirical analysis of farmer-led innovations, Agr. Water Manage., 216, 89–97, https://doi.org/10.1016/j.agwat.2019.01.020, 2019.
Triet, N. V. K., Dung, N. V., Fujii, H., Kummu, M., Merz, B., and Apel, H.: Has dyke development in the Vietnamese Mekong Delta shifted flood hazard downstream?, Hydrol. Earth Syst. Sci., 21, 3991–4010, https://doi.org/10.5194/hess-21-3991-2017, 2017.
Trung, H. M., Vien, N. V., Thanh, D. T., Thuy, N. T., Thanh, H. M., Cassman, K. G., Mew, T. W., Teng, P. S., and Cu, R. M.: Relationships between rice intensification, plant nutrition, and diseases in the Red River Delta. Vietnam and IRRI: A partnership in rice research, in: Proceedings of a conference, 4–7 May 1994, Hanoi, Vietnam, 200–210, 1995.
Tuong, T. P., Kam, S. P., Hoanh, C. T., Dung, L. C., Khiem, N. T., Barr, J., and Ben, D. C.: Impact of seawater intrusion control on the environment, land use and household incomes in a coastal area, Paddy Water Environ., 1, 65–73, https://doi.org/10.1007/s10333-003-0015-2, 2003.
UN Department of Humanitarian Affairs: Vietnam typhoon situation report no. 4, available at: https://reliefweb.int/report/viet-nam/vietnam-typhoon-linda-situation-report-no4 (last access: 20 November 2018), 1997.
United Nations Vietnam: Viet Nam: Tropical storm Mirinae situation update no. 1 (as of 2 August 2016), available at: http://www.un.org.vn/en/publications/doc_details/516-viet-nam-tropical-storm-mirinae-situation-update-no-1-as (last access: 20 November 2018), 2016.
UNW-DPC: Drought situation and management in Vietnam, available at: http://www.droughtmanagement.info/literature/UNW DPC_NDMP_Country_Report_Vietnam_2014.pdf (last access: 20 November 2018), 2014.
USDA: Vietnam: Record rice production forecast on surge in planting in Mekong Delta, available at: https://ipad.fas.usda.gov/highlights/2012/12/Vietnam/ (last access: 16 November 2018), 2012.
van den Berg, L. M., van Wijk, M. S., and van Hoi, P.: The transformation of agriculture and rural life downstream of Hanoi, Environ. Urbaniz., 15, 35–52, https://doi.org/10.1177/095624780301500122, 2003.
Van Kien, N., Han, N. H., and Cramb, R.: Trends in rice-based farming systems in the Mekong Delta, in: White gold: The commercialisation of rice farming in the Lower Mekong Basin, Palgrave Macmillan, Singapore, 347–373, 2020.
Viêt Nam News: Typhoon Nesat wrecks havoc in coastal areas, available at: https://vietnamnews.vn/Miscellany/216044/Typhoon-Nesat-wreaks-havoc-in-coastal-areas.html#LxRMtk4clvvxbUTz.97 (last access: 20 November 2018), 2011.
Vinh, V. D., Ouillon, S., Thanh, T. D., and Chu, L. V.: Impact of the Hoa Binh dam (Vietnam) on water and sediment budgets in the Red River basin and delta, Hydrol. Earth Syst. Sci., 18, 3987–4005, https://doi.org/10.5194/hess-18-3987-2014, 2014.
VNA: Downpours, flooding wreak havoc in northern localities, available at: https://en.vietnamplus.vn/downpours-flooding-wreak-havoc-in-northern-localities/135075.vnp (last access: 20 November 2018), 2018a.
VNA: Floods damage 2,000 ha of rice in Mekong Delta, available at: https://en.vietnamplus.vn/floods-damage-2000ha-of-rice-in-mekong-delta/140921.vnp (last access: 20 November 2018), 2018b.
Winkel, L. H. E., Trang, P. T. K., Lan, V. M., Stengel, C., Amini, M., Ha, N. T., Viet, P. H., and Berg, M.: Arsenic pollution of groundwater in Vietnam exacerbated by deep aquifer exploitation for more than a century, P. Natl. Acad. Sci. USA, 108, 1246–1251, https://doi.org/10.1073/pnas.1011915108, 2011.
WLE Mekong CGIAR: Mapping the dams of the Mekong, available at: https://wle-mekong.cgiar.org/wp-content/uploads/unnamed-11.jpg (last access: 6 May 2021), 2020a.
WLE Mekong CGIAR: Red River basin, available at: https://wle-mekong.cgiar.org/wp-content/uploads/unnamed-11.jpg (last access: 9 January 2021), 2020b.
Workman, D.: Rice exports by country, available at: http://www.worldstopexports.com/rice-exports-country/, last access: 19 November 2020.
Ziegler, A. D., Echaubard, P., Lee, Y. T., Chuah, C. J., Wilcox, B. A., Grundy-Warr, C., Sithithaworn, P., Petney, T. N., Laithevewat, L., Ong, X., Andrews, R. H., Ismail, T., Sripa, B., Khuntikeo, N., Poonpon, K., Tungtang, P., and Tuamsuk, K.: Untangling the complexity of liver fluke infection and cholangiocarcinoma in NE Thailand through transdisciplinary learning, EcoHealth, 13, 316–327, https://doi.org/10.1007/s10393-015-1087-3, 2016.