the Creative Commons Attribution 4.0 License.
the Creative Commons Attribution 4.0 License.
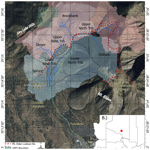
Post-wildfire sediment source and transport modeling, empirical observations, and applied mitigation: an Arizona, USA, case study
Edward R. Schenk
Alex Wood
Allen Haden
Gabriel Baca
Jake Fleishman
Joe Loverich
Post-wildfire floods are receiving greater attention as wildland–urban interfaces become more common and catastrophic wildfires have increased in frequency. Sediment sourcing, transport, and deposition in the post-wildfire environment receive attention due to the severity of risk caused by debris flows and concentrated sediment flood flows. This study compares sediment model predictions based on the Modified Universal Soil Loss Equation (MUSLE), the Watershed Assessment of River Stability and Sediment Analysis (WARSSS) suite of models, and the free internet-based WEPPcloud post-wildfire sediment model for the 2019 Museum Fire (809 ha of steep slope Pinus ponderosa forest on a series of basaltic domes). Empirical evidence from four floods in 2021 indicated 9900 Mg of sediment yield to city of Flagstaff neighborhoods, WEPPcloud estimated 3870 Mg yr−1, MUSLE predicted 4860 Mg yr−1 (based on the four events), and the WARSSS suite of models predicted 4630 Mg yr−1. Both the Watershed Erosion Prediction Project (WEPP) and WARSSS estimated more sediment yield from channels than hillslope (51 %/49 % and 60 %/40 %, respectively) though the spatial patterns differ between the models. The utility of sediment forecasting to inform the application of sediment mitigation structures to help reduce downstream impacts of post-wildfire water and sediment flows is discussed. Continued revisions of sediment forecasts, based on case studies such as this one, can provide researchers, managers, and policymakers with tools for ecological and human risk mitigation and emergency management.
- Article
(14520 KB) - Full-text XML
- BibTeX
- EndNote
Post-wildfire flooding at the wildland–urban interface (WUI) is an increasingly important issue for the health and safety of millions of humans living in or adjacent to semi-arid forests (e.g., Ebel et al., 2023; Kinoshita et al., 2016; Sankey et al., 2017). The development of neighborhoods directly adjacent to forest lands under severe drought conditions creates hazards not just to widespread burning but flooding in the aftermath of those fires (Kinoshita et al., 2016; Sankey et al., 2024). While the changes in hydrologic properties of watersheds after severe wildfires are relatively well known, there is now a need to rapidly assess and mitigate post-wildfire sediment transport and floods to prevent or lessen impacts to safety and property damage. A key portion of this process is understanding the potential for damaging debris flows and sediment sourcing, transport, and aggradation (Moody et al., 2013; Smith et al., 2011). The sediment component of the post-wildfire flood paradigm is perhaps the most damaging due to physical impacts (e.g., damage to infrastructure), bulking factors to flood flow volume, and long-term damage to soil profiles and stream channels that hamper watershed ecosystem recovery (e.g., Moody et al., 2013; Neary et al., 2012; Shakesby, 2011).
In the past 2 decades there has been an emphasis on predicting and remediating post-wildfire sediment sourcing and transport (Shakesby et al., 2016). Most studies have been focused on hillslope and channel processes and determining accurate or precise estimates of sediment fluxes (East et al., 2021; Rengers et al., 2021; Wu et al., 2021). While many studies rely on empirical measurements, there have been recent improvements and updates to post-wildfire sediment modeling. These improvements allow for rapid, relatively inexpensive assessments post-wildfire but potentially at the cost of precision or accuracy (Lopes et al., 2021).
This study explains sediment prediction methods utilized for a relatively small wildfire in Flagstaff, Arizona, USA, to predict sediment quantities as well as flow paths and sedimentation areas for the Museum Fire (2019). These methods have successfully guided mitigation efforts for the nearby Schultz Fire (2010; Neary et al., 2012) and were also used in another nearby fire in 2022 (Pipeline Fire). Three models were compared to empirical observations to provide an estimate of model precision and accuracy: the Watershed Assessment of River Stability and Sediment Analysis (WARSSS), the Modified Universal Soil Loss Equation (MUSLE), and the Watershed Erosion Prediction Project (WEPP). All three were then compared to field observations immediately downstream of the modeling domain in the urban environment.
Additionally, sediment mitigation structures (alluvial fan restoration areas) are discussed at the end of this study and are described as “work areas”. These work areas include alluvial fan restorations that spread flow, allowing for a drop in stream power. The loss in stream power allows for sediment accretion upstream of urban neighborhoods (Grover, 2021; Rosgen and Rosgen, 2015). The work areas were informed and designed by the 2022 modeling effort and were built in late 2022 and early 2023 after the 2021 floods.
The objective of this study is to provide an estimate of the precision and accuracy of three sediment modeling techniques (WARSSS, MUSLE, and WEPP) and determine where future modeling improvements should be focused. All three modeling techniques are available internationally and with low barriers to entry. They are either extremely user-friendly (WEPP in the WEPPcloud user interface) or have large applied technical user bases (MUSLE and WARSSS). A secondary objective is to display the utility of post-wildfire sediment modeling for determining the location and type of sediment mitigation structures. The intent of both objectives is to help researchers and land managers rapidly and accurately predict post-wildfire sediment risk to both ecological and human environments so that sediment mitigation strategies can be developed and implemented to reduce risk to life and the ecosystem.
Flagstaff, Arizona, lies at the edge of the dormant San Francisco Volcanic Field, including the San Francisco Peaks, Dry Lake Hills, and Mount Elden. The local watersheds are generally hydrologically complacent, unless disturbed, with extremely low rainfall–runoff ratios due to local geology (weathered dacite, cinders, and karstic fractured limestone), vegetation (dense Pinus ponderosa forest), and relatively deep soil organic layers (Quisenberry, 2009; Youberg et al., 2019; Schenk et al., 2021). The Spruce Wash watershed is an ephemeral tributary to the Rio de Flag, another ephemeral watershed that drains the southern portions of the San Francisco Volcanic Field. The Spruce Wash watershed drains the six dacite intrusive hills that make up the Dry Lake Hills feature (2695 m) as well as the western portion of Mount Elden (2835 m), a larger protuberance of the same orogeny (Holm, 2019; Schenk et al., 2021). A previous USGS study observed a peak flow of 0.14 m3 s−1 in the Spruce Wash watershed over a period of 11 years (Hill et al., 1988) despite a watershed contributing area of greater than 1450 ha.
The Museum Fire occurred in July 2019 over 800 ha on the steep, mountainous slopes of Dry Lake Hills and Mount Elden, both of which are immediately uphill of the established residential areas of Coconino County (CC) and the city of Flagstaff (CoF; Fig. 1). Mount Elden Estates (MEE; 2160 m) is a rural residential area and is the uppermost residential area within the Spruce Wash watershed. Approximately 1.5 km downstream and separated by open US Forest Service (USFS) land are the urban residential areas of Paradise and Sunnyside (2120 m), which are within the CoF city limits. MEE is located on flatter slopes near the base of Dry Lake Hills on the leading and lower edge of a previously inactive alluvial fan (activated post-wildfire, previously complacent; Fulé et al., 2023). Paradise and Sunnyside are on the toe of inactive alluvial fans and adjacent to the broad, ephemeral, and formerly unchannelized Spruce Wash. Prior to the Museum Fire, the Paradise and Sunnyside neighborhoods had one defined channel–pipe system, and surface water flow seldom occurred within these existing channels. Upgradient on USFS land, ephemeral surface flows were spread over wide alluvial fans (areas of sediment deposition) and were easily absorbed into the unconsolidated sediment. Consequently, pre-wildfire surface water flows within the channels were primarily from stormwater runoff during normal precipitation events from local CoF streets (Schenk et al., 2021; Schiefer and Schenk, 2024).
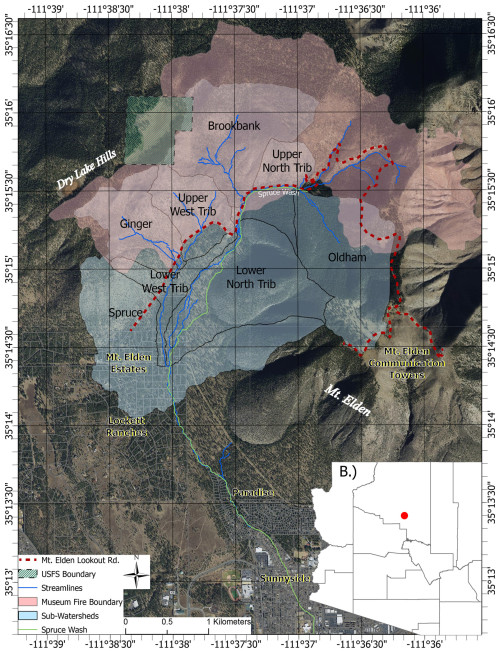
Figure 1Overview map of the 2019 Museum Fire watershed (Spruce Wash), subtributary names, and locations of impacted neighborhoods. The city of Flagstaff is located in north-central Arizona on the edge of the Colorado Plateau and shown on the lower portion of this figure. Background aerial imagery is public domain (Coconino County, 2015).
2.1 Flood events and study timeline
The Flagstaff region saw record-low summer monsoonal rain in 2019 and 2020 with no substantial post-wildfire impacts. Initial post-wildfire-induced flooding occurred during the above-average summer monsoon season of 2021, resulting in several debris flows high within the Spruce Wash watershed and four significant floods that entered the downstream city (Porter et al., 2021; Porter et al., 2023; Schenk et al., 2023; Sankey et al., 2024). Post-wildfire flooding resulted in vast amounts of sedimentation in downstream residential areas as existing drainage features and channels were overwhelmed with sediment and debris (e.g., https://www.weather.gov/fgz/FlagstaffJuly2021). These 2021 flood events allowed for empirical observation of sediment discharge and eventual comparison to the modeled predictions of sediment discharge. The models (WARSSS, MUSLE, and WEPP) were run in late 2021 and early 2022 after the flood events. Model results were directly comparable to the 2021 flood observations and were also used to inform the location and design of the alluvial fan restoration sediment mitigation structures, which were built in late 2022 and early 2023.
3.1 Flood flow modeling
Post-wildfire flood modeling was completed in August 2019 and was based on a 2-D hydrologic–hydraulic numerical model created in FLO-2D (Fuller, 2019, 2022). Initial flood modeling was completed at a 20 ft (6 m) grid scale using 2015 lidar elevation data. Subsequent modeling was completed at a 5 ft (1.8 m) grid scale using a fall 2019 lidar elevation dataset. Both datasets are available on the United States Geological Survey National Map portal (https://www.usgs.gov/programs/, last access: 1 July 2024). All modeling indicates an approximate 10 to 100 times (1–2 orders of magnitude) increase in surface water peak discharge depending on rain event; more information on hydrologic conditions is provided in a conference proceedings paper (Schenk et al., 2023).
3.2 Sediment modeling (WARSSS and MUSLE)
Sediment modeling focused on quantifying relative sediment sources relating to channel and hillslope erosional processes. The Spruce Wash watershed within the Museum Fire burn scar was divided into subwatersheds, based on tributaries in the USGS National Hydrography Dataset, to identify high-sediment-yield areas (USGS, 2019). Low-gradient areas downstream of high-sediment-yield areas were identified as work areas for applied sediment control practices that have the greatest impact on limiting downstream sediment transport (colloquially described as “alluvial fan restorations” elsewhere; https://www.coconino.az.gov/2407/Alluvial-Fan-Stabilization-Project, last access: 1 December 2024; Rosgen and Rosgen, 2015).
The WARSSS model (Rosgen, 2009) was the first modeling suite used for this fire (in 2021 post-flooding) due to its successful sediment transport predictions after the nearby 2010 Schultz Fire (NCD, 2012, Neary et al., 2012). WARSSS is designed to identify the location, nature, extent, and consequences of land use impacts on sediment and to understand the cause of watershed impairment. This approach was developed for application on large watersheds and is practical for the Museum Fire because it uses previously proven rapid-screening field observations that integrate hillslope, hydrologic, and channel processes. The analysis focuses on average annual yield of sediment rather than event-based analyses. The average annual yields do not ignore sediment delivery from large flood events but take into account the overall frequency of these types of flows, based on a 30-year climate average. An annual average sediment yield is used due to the highly heterogenous precipitation distribution in the American Southwest during monsoon storms. This annual average sediment yield, therefore, is appropriate for understanding watershed function and developing watershed restoration practices post-disturbance.
The WARSSS method relies on estimating bank erosion using the Bank Assessment of Non-Point Source Consequences of Sediment (BANCS) model and can quantify bank erosion rates and sediment supply for years with normal discharge patterns (Rosgen, 2009). Average annual hillslope erosion is estimated using the Erosion Risk Management Tool (ERMiT; Robichaud et al., 2014). The Modified Universal Soil Loss Equation (MUSLE; Williams and Berndt, 1977) is utilized to estimate sediment supply from hillslopes during specific precipitation events. Discharges for these events were estimated by a 2-D numerical model with U.S. Natural Resource Conservation Service (NRCS) curve number inputs as part of the post-wildfire flood modeling efforts (Fuller, 2019; Schenk et al., 2023). The MUSLE estimates are provided here as a reference point for larger events. Direct comparison of the different methods is difficult. While post-wildfire hillslope erosion will diminish over time with natural recovery, sediment bank contributions are expected to continue at high rates for many years due to post-wildfire channel evolution processes, from initial incision immediately post-wildfire (Santi et al., 2008) to subsequent widening of channels over time (Benda et al., 2003; Hupp and Simon 1991).
Sediment transport estimates were used to look at how supplied sediment can transport through the channel system. Sediment transport modeling used the FLOWSED–POWERSED platform in the RIVERMorph software (v. 5.1) and provided estimates of average annual sediment transport through a specific cross section of channel given an annual flow scenario (Rosgen, 2009; Hall and Bledsoe, 2023). Estimates of sediment supply into a reach can be compared within the reach to aggradation or degradation for both existing and proposed designs. This analysis is sensitive to several data inputs, including annual flow duration curves (based on watershed size), bankfull discharge, suspended sediment and bedload sediment rating curves, channel configuration, and slope (Rosgen, 2006; Hall and Bledsoe, 2023). These data are difficult to obtain for ungauged ephemeral systems; we used sediment rating curves and dimensionless flow duration curves developed during the 2010 Schultz Fire sediment analysis, which were derived from regional data and research from the U.S. National Forest Service Beaver Creek Experimental Watershed project (Natural Channel Design, 2012).
Once high-sediment-yield areas are identified, sediment transport analyses are conducted at typical channel cross sections that typify the range of channel conditions from upstream to downstream, proposed work areas in the Spruce Wash watershed. In addition to providing an analysis of sediment transport across channels in their current state (fall 2021), an analysis of sediment transport across a conceptualized design channel (a hypothetical 2 % slope post-restoration) was used to understand the feasibility of altering the downstream sediment delivery and was based on the upstream sediment supply.
3.3 Assessing the geomorphic channel condition
3.3.1 BEHI data collection
Bank erosion hazard index (BEHI) surveys were used to qualitatively evaluate all eroding channels within the Spruce Wash watershed (Rosgen, 2009; Fig. 2). Collected data consisted of channel bank height, channel and bank material, length of channel, vegetation and root density, bank slope angle, valley and stream type classification (Rosgen, 1996), and near-bank stress (NBS).
3.3.2 Channel surveys
Channel cross-sectional surveys were completed proximal to proposed work areas (i.e., flood mitigation capital improvements) to accurately model sediment transport through channels and assess channel characteristics. Twenty-seven (27) cross-sectional surveys (Fig. 3), longitudinal channel profiles, and pebble counts were completed to evaluate the channel slope and characteristics of specific channel reaches.
3.4 Estimating sediment yield
3.4.1 Channel sediment yield
The Bank Assessment for Non-point source Consequences of Sediment (BANCS) model was used to estimate annual sediment yield (Rosgen, 2009). The BANCS model utilizes BEHI and NBS survey data to estimate sediment supply from channel bank sources and yields a sediment supply in mass per year. The BANCS model provides reliable estimates of bank erosion but can underestimate bank erosion rates resulting from higher-than-normal flooding and overestimate rates from years with very low peak flows (Rosgen, 1996). Channel sediment supply was converted to Mg yr−1 per longitudinal meter for all evaluated reaches.
3.4.2 Hillslope sediment yield
The Erosion Risk Management Tool (ERMiT) and Modified Universal Soil Loss Equation (MUSLE) models were used to estimate hillslope sediment yield. The ERMiT model predicts sediment yield annually, while the MUSLE model is based on precipitation events (storm-based).
The ERMiT model uses soil burn severity, vegetation type, rock content, hillslope gradient, soil type, hillslope length, and annual precipitation to model sediment yield (Mg yr−1) up to 5 years post-fire (Robichaud et al., 2007). For the scope of this analysis, 2021 was used as the second-year post-wildfire. Therefore, only years 3 (2022), 4 (2023), and 5 (2024) of sediment yield were modeled. To capture the variability in hillslope impacts, the Spruce Wash watershed was subdivided into subcatchments using the watershed delineation in ESRI ArcMap 10.8 (ESRI, 2020). Each catchment was evaluated individually for its sediment yield.
The MUSLE is based on the Universal Soil Loss Equation (USLE) but utilizes transport efficiency and soil erodibility (Igwe et al., 2017). For the post-wildfire watersheds, the MUSLE model is useful for modeling post-wildfire sediment yield because soil erodibility increases due to hydrophobic ash-laden soils, and transport efficiency increases due to increased runoff from decreased infiltration and retention. The MUSLE model input for post-wildfire situations requires instantaneous peak discharge and total volume of 1, 2, and 3 in. (2.5, 5, and 7.5 cm, respectively) precipitation in 1 h events in addition to watershed area, slope, and soil erodibility. Unlike the ERMiT model, the MUSLE model predicts event-based sediment yield in mass per event. Soil erodibility (K values) were estimated for low, medium, and high erodibility at 0.29, 0.545, and 0.8, respectively, based on field conditions. The crop factor (C value) was estimated at 0.003 for forested area, and the slope type (P factor) was inputted as 1 to indicate steep slope. Since the P factor does not provide a measure of the slope, the LS coefficient (slope length) was set at 0.5 to account for steep slopes.
3.5 Observed sediment transport and aggradation
Observed sediment transport and aggradation were collected from CoF staff during 2021 flood events (three in July and one in August). Sediment was assessed qualitatively using photographs of known cross sections as well as quantitatively through landfill tipping fees for sediment removed from the channel and streets post-event. Landfill tipping fees were used as a surrogate for sediment deposition mass, as the landfill calculates fees based on precision scale measurements of truck loads. Each truck load of flood-related sediment was measured for potential federal and state disaster reimbursement, providing a relatively accurate empirical measure of sediment flux to the ultimate outfall (city of Flagstaff neighborhoods).
3.6 Evaluating sediment transport and retention
FLOWSED–POWERSED, a part of the proprietary RIVERMorph software package (Rosgen, 2006), was used to model sediment transport through channels in their current condition (2021) and through conceptual redesigned channels for mitigating sediment transport. FLOWSED–POWERSED predicts average annual sediment transport (Mg yr−1) at a stream reach scale based on flow duration curves for the reach, sediment rating curves for discharge, and the stream power at each stage as determined by channel morphology. Modeling is based on a typical channel cross section at a riffle within each reach. Stream power is calculated at stage intervals depending on cross section shape. Flow duration at each stage and sediment transport rates are utilized to estimate total sediment load at each stream stage and then summed for a total average annual sediment capacity at each reach.
For this study, dimensionless flow duration curves were derived from data collected at nearby watershed studies in Beaver Creek watersheds (Baker, 1982). The dimensionless curves were adjusted using the new estimated “bankfull” discharge, which was derived from runoff models developed for the Museum Fire area (Schenk et al., 2023). The 1-year return interval precipitation event was used for bankfull discharge and approximates the post-wildfire channel-forming discharge. Dimensionless sediment rating curves for the project area were derived from sediment rating curves for nonequilibrium-condition watersheds in the Beaver Creek watershed studies (suspended sediment) and for nonequilibrium-condition post-wildfire watersheds in Colorado, USA (Rosgen, 2010; Rosgen and Rosgen, 2015).
Based on preliminary sediment yield analyses, FLOWSED–POWERSED was modeled at eight proposed work areas. Each analysis consisted of an existing upstream sediment source cross section and a proposed alluvial fan restoration cross section. Upstream sediment source geometries were obtained from previously completed geomorphic surveys. Each analysis was iterated using the same upstream sediment source cross section and a conceptual (proposed) design cross section. The design cross section informed the final work area cross section and was drawn in RIVERMorph to incorporate a design that promotes the greatest amount of sediment retention.
For each model run, FLOWSED and POWERSED required the following inputs: bankfull cross-sectional area, Manning's n value, bankfull discharge, slope, suspended sediment (mg L−1), measured bankfull bedload (lb s−1), a flow duration curve, and a sediment rating curve comparison (data available in NCD, 2022 under Appendix B). FLOWSED models the total annual sediment yield, both suspended and bedload, using flow–duration curves and their corresponding sediment yields. The dimensionless flow–duration curve is developed from representative watersheds in the region using USGS stream gauge data. The POWERSED model compares sediment transport in various configurations of channel geometry.
The FLOWSED–POWERSED model was used to estimate the effect of rebuilding alluvial fans to increase sediment retention upstream of the city. A conceptual design cross section was used at each work area and evaluated for its efficiency in sediment transport. Design cross sections consist of a restored fan feature with the eroded, defined flow paths graded flat and stabilized with lateral rock sills. This added sediment retention was accomplished by widening and repairing the existing channel into a designed alluvial fan channel to fill the valley bottom. This reduces the ability of the channel to transport sediment by lowering shear stress and stream power. The slope of the channel remains the same, but the depth is lowered by allowing for a wider flow path.
3.7 Internet-based sediment source and transport modeling (WEPPcloud)
WEPP (Watershed Erosion Prediction Project) model runs were completed using the WEPPcloud online toolkit (http://wepp.cloud/weppcloud/, last access: 1 November 2024) in 2022 to compare with the WARSSS suite of models presented above. WEPP is a standard post-wildfire sediment tool for the US Forest Service and has been expanded in the last decade to include an online modeling tool based on available topography, soils, and climate data for three continents (Lew et al., 2022). The modeling domain is largely based on the Soil and Water Assessment Toolkit (SWAT) methodology with adjustments based on empirical relations since the initial SWAT development (Dobre et al., 2022). The post-wildfire “disturbed” WEPP model was populated using the USFS BAER team soil burn severity georeferenced raster file for the Museum Fire (available through the USFS InciWeb portal; https://inciweb.nwcg.gov/, last access: 1 February 2025), and model runs were completed using the Cligen precipitation toolbox with a PRISM-modified climate application (see Dobre et al., 2022, and Lew et al., 2022, for more information). The model outlet downstream condition was selected at the Spruce Wash entry into the CoF neighborhoods (Linda Vista Avenue; 35 13′22.74′′, 111 37′31.03′′).
4.1 Channel conditions
Approximately 20 % of the channels in the Museum Fire watershed are incised channels with high sediment contribution from channel and bank processes (G-type channels in the Rosgen classification). G channels were found primarily in the burned, steep, upper reaches of the watershed; however, some were found in reactivated alluvial fans (Fig. 4). Bank erosion from this type of channel can be sediment contribution an order of magnitude higher from bank and channel processes than other nonincised steep-slope channels (Rosgen, 2009). Aggrading, often braided D-type channels or valleys that can support aggrading alluvial fans or riparian floodplains are roughly 15 % of the watershed. While these channels have the potential to store large amounts of sediment, many are gullied post-wildfire and now function as sediment sources rather than sediment sinks. The Rosgen channel type was determined visually during the BEHI surveys, and the results are shown in Fig. 4.
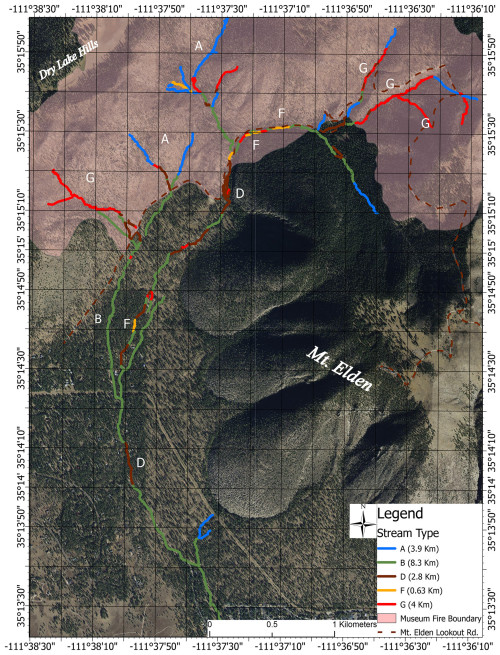
Figure 4Spruce Wash channel types based on the Rosgen classification system. A and B types are generally stable with low sediment contribution. F and G channel types are generally unstable and are sediment sources; D channel types tend to be aggradational (Rosgen 2009). Background aerial imagery is public domain (Coconino County, 2015).
4.2 Sediment yield
4.2.1 Channel and hillslope (ERMiT) sediment yield
The BANCS model estimates a total sediment yield of 9408 Mg yr−1 from stream-bank erosion, while the ERMiT model estimates that hillslope erosion would yield 6300 Mg of sediment in 2022. Combining both methods, sediment yield resulted in a cumulative 15 720 Mg yr−1 of predicted sediment yield from channels and hillslopes in their current conditions for the year 2022 (3 years post-wildfire; Table 1 and Fig. 5). However, these channels do not have the capacity to transport the entire sediment source to the city. The POWERSED and FLOWSED models (Figs. 5 and 6) take transport capacity into account and indicate a transport rate of 4630 Mg yr−1 on average. Empirical observations by CoF staff showed 9900 Mg of sediment delivered to the downstream end of the study site in 2021 from four flood events; the majority of the sediment transported to the city was during the first flood event, despite the magnitude of the flood event being less than some subsequent floods (Schenk et al., 2023).
Table 1BANCS, ERMiT, and total predicted sediment yield for Spruce Wash subwatersheds. BANCS-modeled bank erosion is a result of a channel survey of the current condition, while hillslope erosion is determined as a year-3 post-wildfire ERMiT-modeled sediment yield. Bold numbers indicate subwatersheds where hillslope erosion is predicted to be larger than bank erosion. Values are provided as shown in the model output; precision is likely to the hundredths place. Total predicted sediment transport (as modeled by POWERSED–FLOWSED) is shown at the bottom as well as empirical field observations from 2021 flood events.
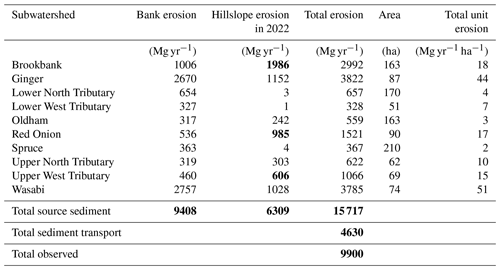
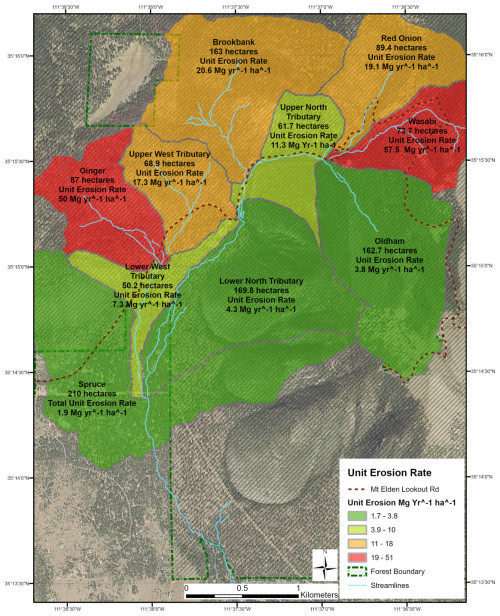
Figure 5Unit erosion rates for each subwatershed based on the ERMiT model. Background aerial imagery is public domain (Coconino County, 2015).
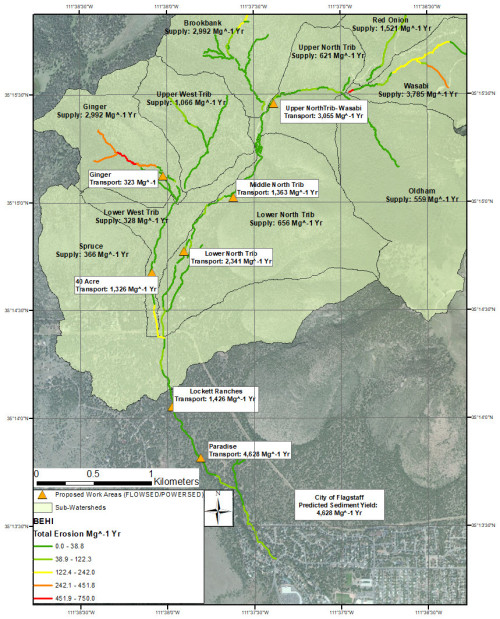
Figure 6Sediment transport capacity for all work areas (constructed in 2022 and 2023) from the POWERSED–FLOWSED model. Background aerial imagery is public domain (Coconino County, 2015).
The BANCS model also estimates the unit bank erosion rate, which is the erosion rate per longitudinal length of channel (0.3 m in this case). Figure 7 presents the unit bank erosion rate for channels in the Spruce Wash watershed, indicating the channels with the highest expected erosion rates. The Ginger and Wasabi subwatersheds, which are two steep watersheds in the burn area, have the highest unit bank erosion rates. The results of the ERMiT model showing the predicted hillslope erosion rates are presented in Fig. 8. The model generally demonstrates the higher hillslope erosion rates in the steeper, burned areas of the watershed.
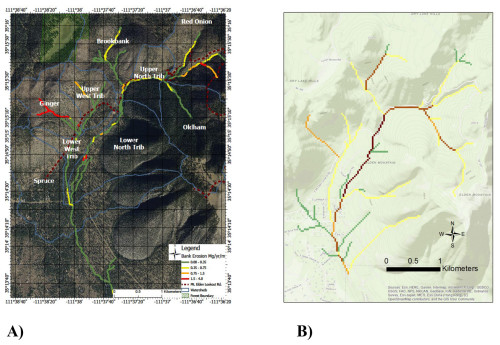
Figure 7(a) BANCS-modeled bank erosion rates for tributaries within the Spruce Wash watershed and (b) the WEPP-modeled bank erosion. There is a similar spatial pattern for Ginger, Brookbank, and the unnamed tributary south of Wasabi; differences exist for the main-stem channel erosion prediction. Background aerial imagery is public domain (Coconino County, 2015) for Fig. 7a; background imagery for Fig. 7b is public domain USGS NLCD data (Homer et al., 2012). © OpenStreetMap contributors 2023. Distributed under the Open Data Commons Open Database License (ODbL) v1.0.
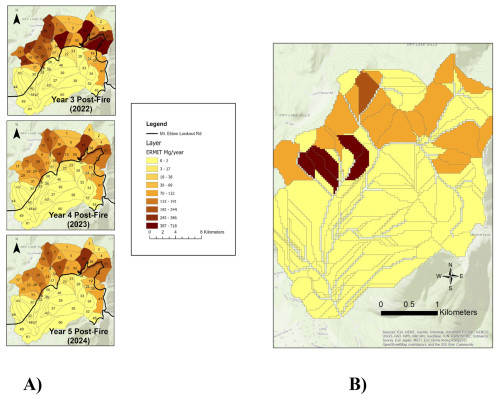
Figure 8Modeled hillslope erosion rates for 2022, 2023, and 2024 for the ERMiT model (a) and 100-year forecasted annual hillslope annual yield for the WEPP model (b). The WEPP model shading is to scale with the ERMiT model. Background imagery is USGS NLCD data in the public domain (Homer et al., 2012). © OpenStreetMap contributors 2023. Distributed under the Open Data Commons Open Database License (ODbL) v1.0.
4.2.2 Hillslope (MUSLE) sediment yield
The MUSLE model also estimates high rates of hillslope erosion for the four modeled precipitation events. The subtributaries utilized for the analysis are the same as those utilized for the average annual sediment transport estimates from FLOWSED–POWERSED analysis. The results vary widely depending on the precipitation event utilized and the erodibility factor (K) of the soils. Based on field observations, the medium K value (0.545) likely represents the best estimate of aggregate soil conditions in the various watersheds within the burn area (Table 2).
Table 2MUSLE model results for soil losses for three different soil erodibility factors (K) for three different rain events for 1, 2, and 3 in. (2.5, 5, and 7.5 cm) in 1 h. The medium K value is the most likely approximator for the 2019 Museum Fire. K values included 0.29, 0.545, and 0.80 for low, medium, and high based on soil conditions. See Fig. 4 for locations of subwatersheds.
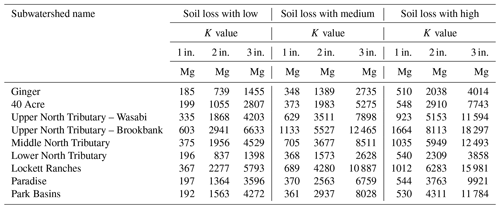
A simplification of the observed 2021 rain events would provide a MUSLE sediment yield estimate of 4860 Mg, with three 1 in. (2.54 cm) rain events in July and one 2 in. (5.08 cm) rain event in August; all have medium K values. No other large rain events occurred in 2021 over the Museum Fire burn scar.
4.3 Sediment transport and retention
Empirical results from in-city sediment removal, as measured at the Cinder Hills Landfill, are provided in Fig. 9. The majority of sediment removed from the urban environment occurred after the first storms post-wildfire, during July (6260 Mg) with an additional 3760 Mg removed after the larger August flood event. All in-city sediment removed is downstream of the modeling regimes and acts as an end point for the study system.
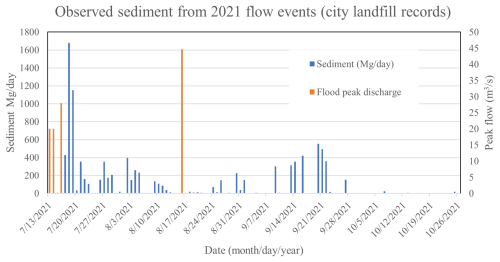
Figure 9Sediment and debris removed from channels and streets. Flood events occurred on 13 July, 14 July, 16 July, and 17 August. Flood flows were predicted at the upstream entry to the CoF as 20, 20, 28, and 44.7 m3 s−1, respectively (Schenk et al., 2023).
The upstream FLOWSED–POWERSED sediment transport and on-forest retention modeling determined that five of the seven work area channel cross sections currently transport more sediment than is supplied to them, potentially leading to upgradient headcutting and continued erosion (highlighted in bold in Table 3). These five proposed work area cross sections transport sediment more efficiently than the upstream sediment source cross section due to channel geometry, generally due to a headcut working into a D channel and converting it into a G channel. Once this process has begun, it exacerbates headcutting and fan degradation, channel migration, and bank erosion, and it provides little to no sediment aggradation (retention or deposition) on the now disconnected alluvial fan. Without direct intervention, these fans and channels will continue to efficiently transport sediment downstream towards the residential areas.
Sediment transport modeling results (FLOWSED–POWERSED) indicate that design cross sections retain an average of 70 % of incoming sediment in proposed work areas compared to the degraded alluvial fans and channels in their current (fall 2021) condition (Table 3). It should be noted that large, single events are not modeled by this analysis and could potentially deliver more sediment. Flow events in 2022 were muted in Spruce Wash due to small rain events; the alluvial fan sites that were constructed prior to monsoon season did appear to function well in terms of sediment aggradation and attenuation (Fig. 10a, b). However, there were no flow events that overtopped the channel within the city to provide empirical comparisons. Field observations of similarly designed sediment retention structures on the nearby 2022 Pipeline Fire burn scar showed consistent sedimentation in the 70 % to 80 % range, based on repeat surveys and sediment haul-off from events in 2022 and 2023 (Tiffany Construction LLC and Coconino County Flood Control District, personal communications, 2023; Beers et al., 2023).
Table 3FLOWSED–POWERSED model results indicating potential sediment retention for proposed sediment basins. Values in bold are net erosional alluvial fans in the current (2021) condition; values in italic indicate net aggradation (sediment storage). The annual sediment transport rate to the city neighborhoods is shown at the bottom in bold italics (4628 Mg yr−1).
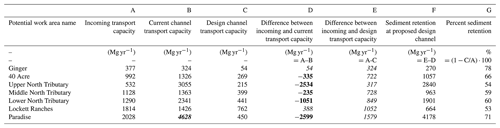
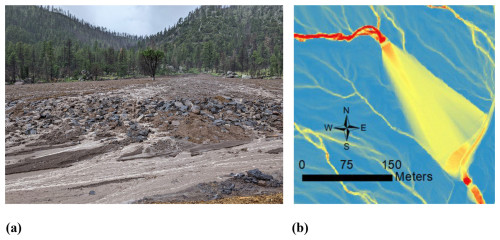
Figure 10(a) Ginger alluvial fan work site (35°15′10′′, −111°38′0′′, looking upstream) during a July 2022 flow event. Note the spread of flow and subsequent drop in water velocity, allowing sediment aggradation. (b) Ginger alluvial fan work site including predicted velocities during a 25-year annual exceedance probability rain event. The dark red areas are approximately 3 m s−1; the blue areas denote no flow (0 m s−1). The photograph in Fig. 10a was taken at the southeast corner of the fan looking northwest (upstream).
The commonly used WEPP model demonstrated lower annual sediment yields (3870 Mg yr−1) ) than the WARSSS model (4630 Mg yr−1) and empirical results (9900 Mg yr−1 in 2021) for the Museum Fire burn scar and Spruce Wash watershed. The event-based MUSLE model (4860 Mg yr−1) was comparable with the WARSSS results but lower than the empirical results.
The 2019 Museum Fire and the subsequent nearby 2022 Pipeline Fire demonstrated that previous hydrologic forecasts for watershed disturbance in the northern Arizona region were largely correct. Two studies of local ponderosa pine (Pinus ponderosa) forests indicated that current conditions exhibited complacent watersheds but that the threat of wildfire would enhance runoff by 1–2 orders of magnitude (Leao and Tecle, 2005; Quisenberry 2009), similar to observations in this burned watershed (Schenk et al., 2023; Sankey et al., 2024). Recent sediment risk predictions were also prescient, indicating sediment transport post-disturbance orders of magnitude higher (e.g., Neary et al., 2012; Natural Channel Design, 2012).
The four 2021 flooding events demonstrated a high sediment supply from the burn area with an observed rate of greater than 9900 Mg into the neighborhoods from the four flood events alone. The WEPP and WARSSS models appear to underestimate sediment delivery by roughly 50 % based on empirical observations of the above-average monsoon season of 2021. A portion of the underestimation of both modeling regimes is the lack of ability to anticipate hillslope gully incision. The reasons for the relatively large contribution from hillslope gully and rill erosion are not completely known at this time but are likely partly due to the long period of watershed complacency in the San Francisco Volcanic Field (estimated at several thousand years; Stempniewicz, 2014; Fulé et al., 2023), leading to abnormally large amounts of stored hillslope and channel sediment at risk of transport after drought-fueled catastrophic wildfires (Neary et al., 2012; Vanmaercke et al., 2021). The large antecedent sediment storage volume is not accounted for in WEPP or MUSLE and only partly accounted for in WARSSS through the empirical measurements used to inform BANCS. Other factors likely include uncertainty in the empirical estimates (both overestimations due to water volume in the sediment/debris loads and underestimation due to floodplain areas not addressed by flood cleanup efforts) and WARSSS and WEPP model limitations for rill and gully erosion processes (hillslope incision). Hillslope gullying is one of the most prevalent forms of erosion in Arizona post-wildfire environments, making the estimation of their sediment yield vitally important (Neary et al., 2012). Other case studies have also shown that WEPP underestimates post-wildfire erosion, as does MUSLE (e.g., Fernández and Vega, 2018; East et al., 2021). There are still very few case studies of WARSSS for post-wildfire sediment modeling. The advantage of WEPP over WARSSS is its ease of use, free availability, and rapid learning curve, allowing for rapid spatial determination of high-risk locations (Lew et al., 2022; East et al., 2024). However, WEPPcloud does not incorporate ground-truthed data, as is evident when comparing spatial “hot spots” of sediment yield in this case study. The WARSSS bank and hillslope predictions were informed by field measurements that largely corresponded with qualitative observations of spatial sediment yield.
All three modeling domains – MUSLE, WEPP, and WARSSS – showed drastic increases in channel and hillslope sediment yields post-wildfire in this case study. Both WEPP and WARSSS predict slightly more sediment yield from existing channels than from hillslope processes. The similarity between model results, as well as the comparison of less than an order of magnitude with empirical results, indicates that both WEPPcloud and WARSSS are useful for sediment predictions. There has been some controversy about the use of “natural channel design” versus “analytical channel design” for applied geomorphology projects (e.g., Lave, 2009; Kasprak et al., 2016). This case study suggests that both trains of thought have validity in the post-wildfire environment. Continued updates to post-wildfire sediment modeling has been called for by disparate studies at a global level (e.g., Lopes et al., 2021; Partington et al., 2022; Ebel et al., 2023), and the hope is this case study provides support for future improvements in the post-wildfire sediment monitoring, modeling, and applied mitigation arena.
In this study, most high-erosion areas are identified high in the watershed. Steep slopes and lack of accessibility likely preclude active restoration of these channels or any hillslope activities other than revegetation by hand labor. Frequent debris flows, a separate sediment transport mechanism, also complicate restoration in the headwater steep slopes (Porter et al., 2023; McGuire et al., 2024b). The nature of the channels (mostly G and F “Rosgen” type channels) indicates that the channel form is in the early stages of evolving to a stable form (Rosgen, 2009). Formation of a small floodplain and reasonably stable channel side slopes (2H : 1V minimum) will require the erosion of significant amounts of sediment. The process will likely take years to decades before relative stability has been reached (e.g., Hupp and Simon, 1991; Montgomery and Buffington, 1993; Jumps et al., 2022). As such, there is a potential for elevated sediment loading for the foreseeable future and subsequent elevated life and safety risk to the community (Fuller, 2024; McGuire et al., 2024a).
Several subwatersheds were identified that exhibited higher hillslope erosion rates than adjacent channels. Initial post-wildfire sediment studies found that channel processes are generally larger sources of erosion, though that narrative is rapidly changing with more case studies and better landscape scale surveying and monitoring (Neary et al., 2012; Rengers et al., 2016; McGuire et al., 2024a). The nonequilibrium hillslope conditions are cause for concern if they do not begin to improve soon, as high sediment loads from hillslopes will generally contribute to further degradation of the receiving channel. Two consecutive years of drought likely contributes to this condition. However, continued erosion and rilling hinder seed establishment, further retarding recovery. The sediment transport models indicate a high potential for successful reduction in sediment as flows cross restored alluvial fan areas. This was observed in 2022 where observations at the nearby Pipeline Fire indicated a sediment retention greater than 70 % in the completed alluvial fan projects within some of the impacted watersheds (Lucinda Andreani, Coconino County Flood Control District Administrator, personal communication, 2023). Similarly, this was observed after the 2012 Waldo Canyon Fire in Colorado, USA, which contained similar alluvial fan restorations (Rosgen and Rosgen, 2015). However, there were some steep-slope (> 2 %) alluvial fan work areas in Spruce Wash that performed poorly due to floods greater than the design storm (Beers et al., 2023; Rebecca Beers, personal communication, 2023). Some fan areas (especially the West Tributary or Ginger) have the potential to reduce not only sediment transport but also sediment contribution from bank erosion. Current high bank erosion rates can be eliminated by eliminating the current gullied channel and restoring the fan function. Fan areas on the main channel of Spruce Wash that already store some sediment can be greatly improved by grading to restore the consistent fan feature.
The sediment mitigation structures, or work areas, consist of an upstream, single-thread “feeder” channel and a multithread anastomosing “fan” channel supported by lateral hardened grade control (e.g., rock sills) before constricting back to a single-thread channel to feed into the existing drainage downstream (see Rosgen and Rosgen, 2015, for more detail). Sediment output from the restored fans appears to be moderate (approximately half of upstream sediment input) over a multi-year average (authors' field observations). However, the relatively steep fans will produce higher shear stresses at high, infrequent flows. For example, peak discharges modeled for a 2 (5 cm h−1) precipitation event over the whole watershed (∼37 m3 s−1, 4 % annual exceedance probability) produce enough shear stress on the Paradise fan to move 30 cm diameter sediment. Consequently, these infrequent precipitation scenarios will have the potential to move large quantities of material through the fan system, even though most is retained on the fan.
The need for accurate and rapid post-wildfire sediment yield and transport modeling is evident by the increased role of wildfires in the wildland–urban interface and subsequent flooding. This case study shows the utility of both WEPPcloud and WARSSS for predicting sediment transport to the city of Flagstaff, Arizona. The agreement between both models for sediment sourcing and transport, as well as the comparison of within an order of magnitude to empirical observations from flood events in 2021, is encouraging. The difference between models was largely in the spatial pattern of sediment yield. Both models indicated a slightly higher contribution from channels than hillslopes, but WARSSS, because it is partly empirically based, was better at identifying hot spots of both channel and hillslope sediment yield. Hillslope sediment yield nearly matched channels, indicating a high degree of hillslope gully and rill erosion, a process that needs further study. Continued advancements in post-wildfire sediment modeling will help inform managers and policymakers on sediment and flood mitigation strategies, planning, and design.
This study also introduced a post-wildfire sediment mitigation strategy through the restoration of alluvial fans. The fan work areas were identified using the POWERSED–FLOWSED sediment transport model, which was likewise informed by the sediment yield predictions of BANCS and ERMiT in the WARSSS model. Each restored fan work area included the removal of a single-thread channel to a graded slope with lateral rock sills for grade control. These mitigations allow for the natural creation of an anastomosing channel that drops out sediment due to the change in shear stress and stream power. Initial sediment transport model results indicate a reduction in downstream sediment transport of 70 %. Ongoing monitoring of these mitigation structures is occurring both in Spruce Wash as well as in adjacent burn scar areas in Coconino County, Arizona; initial results indicate success during small to moderate flow events.
All datasets are referenced in their respective original technical reports (which are provided in the reference list). The hydrology datasets are available in Schenk et al. (2021, 2023), the soil burn severity data are available at https://inciweb.wildfire.gov/ (U.S. Forest Service, 2025), the 2021 precipitation event data are available at https://www.weather.gov/fgz/FlagstaffJuly2021 (U.S. National Weather Service, 2021), and the geomorphic field data are available in Natural Channel Design (2022). The 2015 and 2019 lidar elevation datasets are available on the United States Geological Survey National Map portal (https://www.usgs.gov/programs/, U.S. Geological Survey, 2025).
ES: project administration, resources, visualization, data curation, funding acquisition, and writing. AW: sediment investigation, formal analysis, writing, and data curation. AH: methodology, project administration, funding acquisition, supervision, review, and resources. GB: review and substantial editing, data curation, and formal analysis. JF: data curation and formal analysis. JL: data curation and formal analysis, including hydrology and FLO-2D modeling.
The contact author has declared that none of the authors has any competing interests.
Publisher’s note: Copernicus Publications remains neutral with regard to jurisdictional claims made in the text, published maps, institutional affiliations, or any other geographical representation in this paper. While Copernicus Publications makes every effort to include appropriate place names, the final responsibility lies with the authors.
This project is a collaboration of many partners from Coconino County, the City of Flagstaff, the US Forest Service, the US National Weather Service, and Northern Arizona University, and there was a great deal of assistance from local hydrology and civil engineering consultants. Special acknowledgement goes to Lucinda Andreani, Coconino County Flood Control District Administrator, for her leadership during multiple fires and subsequent flood events in the Flagstaff area. Funding was provided by the Coconino County general fund, the Coconino County Flood Control District, and the City of Flagstaff Stormwater Fund. We would like to thank our journal reviewers as well as our colleagues for informal reviews, comments, and suggestions, which improved this article a lot compared to our original work.
Funding was provided by the Coconino County general fund, the Coconino County Flood Control District, and the City of Flagstaff Stormwater Fund.
This paper was edited by Olivier Dewitte and reviewed by two anonymous referees.
Baker Jr., M. B.: Hydrologic regimes of forested areas in the Beaver Creek watershed. USDA Forest Service, General Technical Report RM-90, http://beavercreek.nau.edu/assets/publications/Publications/Hydrology/Hydrologic Regimes.pdf (last access: 1 December 2024), 1982.
Beers, R., Youberg, A., McGuire, L., Robichaud, P., and Schenk, E. R.: Monitoring the efficacy of novel flood-mitigation structures below the 2022 Pipeline Fire Scar, GSA Connects abstract 14-10, Pittsburgh, PA, https://doi.org/10.1130/abs/2023AM-391399, 2023.
Benda, L., Miller, D., Bigelow, P., and Andras, K.: Effects of post-wildfire erosion on channel environments, Boise River, Idaho, Forest Ecol. Manag., 178, 105–119, 2003.
Coconino County: Aerial orthoimagery of Coconino County. Digital database, https://data-coconinocounty.opendata.arcgis.com/ (last access: 1 January 2024), 2015.
Dobre, M., Srivastava, A., Lew, R., Deval, C., Brooks, E. S., Elliot, W. J., and Robichaud, P. R.: WEPPcloud: an online watershed-scale hydrologic modeling tool. Part II. Model performance assessment and applications to forest management and wildfires, J. Hydrol., 610, 127776, https://doi.org/10.1016/j.jhydrol.2022.127776, 2022.
East, A. E., Logan, J. B., Dartnell, P., Lieber-Kotz, O., Cavagnaro, D. B., McCoy, S. W., and Lindsay, D. N.: Watershed sediment yield following the 2018 Carr fire, Whiskeytown national recreation area, northern California, Earth and Space Science, 8, e2021EA001828, https://doi.org/10.1029/2021EA001828, 2021.
East, A. E., Logan, J. B., Dow, H. W., Smith, D. P., Iampietro, P., Warrick, J. A., Lorenson, T. D., Hallas, L., and Kozlowicz, B.: Post-fire sediment yield from a central California watershed: Field measurements and validation of the WEPP model, Earth and Space Science, 11, e2024EA003575, https://doi.org/10.1029/2024EA003575, 2024.
Ebel, B. A., Shephard, Z. M., Walvoord, M. A., Murphy, S. F., Partridge, T. F., and Perkins, K. S.: Modeling Post-Wildfire Hydrologic Response: Review and Future Directions for Applications of Physically Based Distributed Simulation, Earth's Future, 11, e2022EF003038, https://doi.org/10.1029/2022EF003038, 2023.
ESRI: ArcGIS Desktop: Release 10.8. Redlands, CA: Environmental Systems Research Institute, https://www.esri.com/en-us/arcgis/products/arcgis-desktop/resources (last access: 11 February 2025), 2020.
Fernández, C. and Vega, J. A.: Evaluation of the rusle and disturbed wepp erosion models for predicting soil loss in the first year after wildfire in NW Spain, Environ. Res., 165, 279–285, 2018.
Fulé, P. Z., Barrett, M. P., Cocke, A. E., Crouse, J. E., Roccaforte, J. P., Normandin, D. P., Covington, W. W., Moore, M. M., Heinlein, T. A., Stoddard, M. T., and Rodman, K. C.: Fire Regimes Over a 1070-m Elevational Gradient, San Francisco Peaks/Dook'o'oosłííd, Arizona USA, https://doi.org/10.21203/rs.3.rs-2647940/v1, 2023.
Grover, H.: Mitigating postfire runoff and erosion in the southwest using hillslope and channel treatments. ERI Working Paper, https://aftertheflames.com/wp-content/uploads/2021/02/44_Working-Paper_Mitigating-Postfire-Erosion_WEB.pdf (last access: 1 January 2024), 2021.
Hall, H. R. and Bledsoe, B. P.: Integrating channel design and assessment methods based on sediment transport capacity in gravel bed streams, J. Am. Water Resour. As., 59, 681–700, doi.org/10.1111/1752-1688.13108, 2023.
Hill, G. W., Hales, T. A., and Aldridge, B. N.: Flood hydrology near Flagstaff, Arizona, Vol. 87, No. 4210, Department of the Interior, US Geological Survey, https://pubs.usgs.gov/publication/wri874210 (last access: 1 January 2024), 1988.
Holm, R. F.: Geology of Flagstaff and Geologic History of Rio de Flag, Northern Arizona with Trail Guides to Geology along Rio de Flag, Arizona Geological Survey Down-To-Earth #23, https://repository.arizona.edu/handle/10150/632924 (last access: 1 January 2024), 2019.
Homer, C. H., Fry, J. A., and Barnes, C. A.: The national land cover database, US Geological Survey Fact Sheet, 3020(4), 1–4, https://doi.org/10.3133/fs20123020, 2012.
Hupp, C. R. and Simon, A.: Bank accretion and the development of vegetated depositional surfaces along modified alluvial channels, Geomorphology, 4, 111–124, 1991.
Igwe, P. U., Onuigbo, A. A., Chinedu, O. C., Ezeaku, I. I., and Muoneke, M. M.: Soil Erosion: A Review of Models and Applications, International Journal of Advanced Engineering Research and Science, 4, 138–150, https://doi.org/10.22161/ijaers.4.12.22, 2017.
Fuller, J. E.: Museum Fire Post-wildfire Flood Risk Analysis. Technical Report to the Coconino County Flood Control District, 27 pp., https://flagstaff.az.gov/DocumentCenter/View/88148/Spruce-Watershed---Pre-vs-Post-Fire-Comparison (last access: 1 February 2025), 2019.
Fuller, J. E.: Pipeline Fire Post-wildfire Flood Risk Analysis. Technical Report to the Coconino County Flood Control District, https://flagstaff.az.gov/DocumentCenter/View/88147/Pipeline-West-Postfire-H-and-H-Report-October-2022 (last access: 1 February 2025), 2022.
Fuller, J. E.: Spruce Wash – Futurecasting of Long-Term Hydrologic Impacts from the 2019 Museum Fire with a Comparison to the 1977 Radio Fire, Technical Report to the City of Flagstaff, https://www.flagstaff.az.gov/DocumentCenter/View/79448/Spruce-Wash—Futurecasting-of-Long-Term-Hydrologic-impacts—20240320 (last access: 1 December 2024), 2024.
Jumps, N., Gray, A. B., Guilinger, J. J., and Cowger, W. C.: Wildfire impacts on the persistent suspended sediment dynamics of the Ventura River, California, J. Hydrol.: Regional Studies, 41, 101096, https://doi.org/10.1016/j.ejrh.2022.101096, 2022.
Kasprak, A., Hough-Snee, N., Beechie, T., Bouwes, N., Brierley, G., Camp, R., Fryirs, K., Imaki, H., Jensen, M., O'Brien, G., and Rosgen, D.: The blurred line between form and process: a comparison of stream channel classification frameworks, PloS One, 11, e0150293, https://doi.org/10.1371/journal.pone.0150293, 2016.
Kinoshita, A. M., Chin, A., Simon, G. L., Briles, C., Hogue, T. S., O'Dowd, A. P., Gerlak, A. K., and Albornoz, A. U.: Wildfire, water, and society: Toward integrative research in the “Anthropocene”, Anthropocene, 16, 16–27, 2016.
Lave, R..: The Controversy Over Natural Channel Design: Substantive Explanations and Potential Avenues for Resolution, J. Am. Water Resour. As., 45, 1519–1532, 2009.
Leao, D. and Tecle, A.: A Review of the Hydrology of the Upper Rio de Flag Watershed, Flagstaff, AZ, Journal of Arizona-Nevada Academy of Sciences, https://repository.arizona.edu/handle/10150/296634 (last access: 1 January 2024), 2005.
Lew, R., Dobre, M., Srivastava, A., Brooks, E. S., Elliot, W. J., Robichaud, P. R., and Flanagan, D. C.: WEPPcloud: an online watershed-scale hydrologic modeling tool. Part I. Model description, J. Hydrol., 608, 127603, https://doi.org/10.1016/j.jhydrol.2022.127603, 2022.
Lopes, A. R., Girona-García, A., Corticeiro, S., Martins, R., Keizer, J. J., and Vieira, D. C. S.: What is wrong with post-fire soil erosion modelling? A meta-analysis on current approaches, research gaps, and future directions, Earth Surf. Proc. Land., 46, 205–219, 2021.
McGuire, L. A., Ebel, B. A., Rengers, F. K., Vieira, D. C., and Nyman, P.: Fire effects on geomorphic processes, Nature Reviews Earth & Environment, 5, 486-503, 2024a.
McGuire, L. A., Rengers, F. K., Youberg, A. M., Gorr, A. N., Hoch, O. J., Beers, R., and Porter, R.: Characteristics of debris-flow-prone watersheds and debris-flow-triggering rainstorms following the Tadpole Fire, New Mexico, USA, Nat. Hazards Earth Syst. Sci., 24, 1357–1379, https://doi.org/10.5194/nhess-24-1357-2024, 2024b.
Montgomery, D. R. and Buffington, J. M.: Channel classification, prediction of channel response, and assessment of channel condition, University of Washington, Seattle, p. 84, https://w.krisweb.com/biblio/gen_wadnr_montgomeryetal_1993_tfwsh1093002.pdf (last access: 1 February 2025), 1993.
Moody, J. A., Shakesby, R. A., Robichaud, P. R., Cannon, S. H., and Martin, D. A.: Current research issues related to post-wildfire runoff and erosion processes. Earth-Science Reviews, 122, 10–37, https://doi.org/10.1016/j.earscirev.2013.03.004, 2013.
Natural Channel Design (NCD): Schultz Fire and Flood Assistance Area: Sediment Analysis Refinement & Reduction Options Technical Report, http://ncdengineeringinc.com/wp-content/uploads/2025/02/Sediment-Refinement-Study-Final-v5.pdf (last access: 1 February 2025), 2012.
Natural Channel Design (NCD): Museum Fire Sediment Reduction Project, Sediment Budget Analysis. Natural Channel Design Inc. technical report to Coconino County, Flagstaff AZ, USA, http://ncdengineeringinc.com/wp-content/uploads/2025/02/Museum-Fire-Sediment-Analysis-Final-Report.pdf last access: 1 February 2025), 2022.
Neary, D. G., Koestner, K. A., Youberg, A., and Koestner, P. E.: Post-wildfire rill and gully formation, Schultz Fire 2010, Arizona, USA, Geoderma, 191, 97–104, 2012.
Neary, D. G., Koestner, K. A., and Youberg, A.: Hydrologic impacts of high severity wildfire: Learning from the past and preparing for the future. In 24th Annual Symposium of the Arizona Hydrological Society, 18–20, https://www.researchgate.net/profile/Daniel-Neary-2/publication/267379594_Hydrologic_Impacts_of _High_Severity_Wildfire_Learning_from_the_Past_and _Preparing_for_the_Future/links/557a0a7708ae752158717 cf6/Hydrologic-Impacts-of-High-Severity-Wildfire-Learning-from-the-Past-and-Preparing-for-the-Future.pdf (last access: 1 February 2025), 2012.
Partington, D., Thyer, M., Shanafield, M., McInerney, D., Westra, S., Maier, H., Simmons, C., Croke, B., Jakeman, A. J., Gupta, H., and Kavetski, D.: Predicting wildfire induced changes to runoff: A review and synthesis of modeling approaches, Wires Water, 9, e1599, https://doi.org/10.1002/wat2.1599, 2022.
Porter, R., Joyal, T., Beers, R., Loverich, J., Laplante, A., Spruell, J., Youberg, A., Schenk, E., Robichaud, P., and Springer, A.: Seismic Monitoring of Post-Wildfire Debris Flows Following the 2019 Museum Fire, Arizona, Front. Earth Sci., 9, 235, https://doi.org/10.3389/feart.2021.649938, 2021.
Porter, R., Joyal, T., Beers, R., Youberg, A., Loverich, J., Schenk, E., and Robichaud, P. R.: Characterization of Environmental Seismic Signals in a Post-Wildfire Environment: Examples From the Museum Fire, AZ, J. Geophys. Res.-Earth, 128, e2022JF006962, https://doi.org/10.1029/2022JF006962, 2023.
Quisenberry, J. K.: Impacts of Climate Instability on Flood Management Decisions of the Rio De Flag in Flagstaff, Arizona, Journal Arizona-Nevada Academy of Science, https://repository.arizona.edu/handle/10150/296693 (last access: 1 February 2025), 2009.
Rengers, F. K., Tucker, G. E., Moody, J. A., and Ebel, B. A.: Illuminating wildfire erosion and deposition patterns with repeat terrestrial lidar, J. Geophys. Res.-Earth, 121, 588–608, 2016.
Rengers, F. K., McGuire, L. A., Kean, J. W., Staley, D. M., Dobre, M., Robichaud, P. R., and Swetnam, T.: Movement of sediment through a burned landscape: Sediment volume observations and model comparisons in the San Gabriel Mountains, California, USA, J. Geophys. Res.-Earth, 126, e2020JF006053, https://doi.org/10.1029/2020JF006053, 2021.
Robichaud, P. R., Elliot, W. J., Pierson, F. B., Hall, D. E., Moffet, C. A., and Ashmun, L. E.: Erosion Risk Management Tool (ERMiT) user manual (version 2006.01.18), Gen. Tech. Rep. RMRS-GTR-188, Fort Collins, CO: US Department of Agriculture, Forest Service, Rocky Mountain Research Station, 188, 24 pp., https://doi.org/10.2737/RMRS-GTR-188, 2007.
Robichaud, P. R., Elliot, W. J., Pierson, F. B., Hall, D. E., and Moffet, C. A.: Erosion Risk Management Tool (ERMiT), Moscow, ID: US Department of Agriculture. Forest Service, Rocky Mountain Research Station, https://forest.moscowfsl.wsu.edu/fswepp/ (last access: 1 November 2024), 2014.
Rosgen, D. and Rosgen, B.: Restoring alluvial fan connectivity for post-fire flood alleviation and sediment reduction, Proceedings of the Third Joint Federal Interagency Conference, 5th Federal Interagency Hydrologic Modeling Conference and 10th Federal Interagency Sediment Conference, Reno, Nevada, 1350–1360, https://www.flagstaff.az.gov/DocumentCenter/View/88146/Rosgen-and-Rosgen-2015 (last access: 1 February 2025), 2015.
Rosgen, D. L.: Applied River Morphology, Second Edition. Wildland Hydrology, Ft. Collins, CO USA, ISBN 0965328902, 9780965328906, 1996.
Rosgen, D. L.: FLOWSED-POWERSED: Prediction models for suspended and bedload transport, in: Proc. Eighth Federal Interagency Sedimentation Conference, Reno, Nevada, 1, 761–769, https://pubs.usgs.gov/misc/FISC_1947-2006/pdf/1st-7thFISCs-CD/8thFISC/8thFISC.pdf (last access: 1 February 2025), 2006.
Rosgen, D. L.: The application and validation of dimensionless sediment rating curves, 2nd Joint Federal Interagency Conference, Las Vegas, NV, 27 June–1 July, https://wildlandhydrology.com/resources/docs/FLOWSED-POWERSED/Rosgen_2010_DSRCs.pdf (last access: 1 February 2025), 2010.
Rosgen, D. L.: Watershed assessment of river stability and sediment supply (WARSSS), Wildland Hydrology, ISBN 0979130832, 9780979130830, 2009.
Sankey, J. B., Kreitler, J., Hawbaker, T. J., McVay, J. L., Miller, M. E., Mueller, E. R., Vaillant, N. M., Lowe, S. E., and Sankey, T. T.: Climate, wildfire, and erosion ensemble foretells more sediment in western USA watersheds, Geophys. Res. Lett., 44, 8884–8892, 2017.
Sankey, T. T., Tango, L., Tatum, J., and Sankey, J. B.: Forest fire, thinning, and flood in wildland-urban interface: UAV and lidar-based estimate of natural disaster impacts, Landscape Ecol., 39, 58, https://doi.org/10.1007/s10980-024-01811-5, 2024
Santi, P. M., DeWolfe, V. G., Higgins, J. D., Cannon, S. H., and Gartner, J. E.: Sources of debris flow material in burned areas, Geomorphology, 96, 310–321, https://doi.org/10.1016/j.geomorph.2007.02.022, 2008.
Schenk, E. R., Schiefer, E., Young, E., and Helton, C.: Surface Water Hydrology and Flood Recurrence in the Flagstaff, Arizona Area, 2008–2019, City of Flagstaff Technical Report, Flagstaff, AZ, 91 pp., https://doi.org/10.4211/hs.8da8bb7cb66d475ea03af1a79b38a446, 2021.
Schenk, E. R., Loverich, J., and Haden, A.: Modeling post-wildfire flood dynamics to determine urban stormwater infrastructure needs: Flagstaff Arizona case study, SEDHYD Proceedings, Saint Louis, Missouri, https://www.sedhyd.org/2023Program/1/165.pdf (last access: 1 February 2025), 2023.
Schiefer, E. and Schenk, E.: Changed Seasonality and Forcings of Peak Annual Flows in Ephemeral Channels at Flagstaff, Northern Arizona, USA, Hydrology, 11, 115, https://doi.org/10.3390/hydrology11080115, 2024.
Shakesby, R. A.: Post-wildfire soil erosion in the Mediterranean: Review and future research directions, Earth-Sci. Rev., 105, 71–100, 2011.
Shakesby, R. A., Moody, J. A., Martin, D. A., and Robichaud, P. R.: Synthesising empirical results to improve predictions of post-wildfire runoff and erosion response, Int. J. Wildland Fire, 25, 257–261, 2016.
Smith, H. G., Sheridan, G. J., Lane, P. N., Noske, P. J., and Heijnis, H.: Changes to sediment sources following wildfire in a forested upland catchment, southeastern Australia, Hydrol. Process., 25, 2878–2889, https://doi.org/10.1002/hyp.8050, 2011.
Stempniewicz, V. A.: Evaluating erosion risk mitigation due to forest restoration treatments using alluvial chronology and hydraulic modeling, MS Thesis, Northern Arizona University, 169 pp., https://www.proquest.com/openview/b0fbc063ff93ddc059889cb40e4302b1/1?pq-origsite=gscholar&cbl=18750 (last access: 1 February 2025), 2014.
U.S. Forest Service: Museum Fire soil burn severity map, https://inciweb.wildfire.gov/, last access: 12 February 2025.
U.S. Geological Survey: The National Map, https://www.usgs.gov/programs/national-geospatial-program/national-map last access: 13 February 2025.
U.S. National Weather Service: Flash flooding in Flagstaff July 13 & 14, https://www.weather.gov/fgz/FlagstaffJuly2021 (last access: 13 February 2025), 2021.
USGS (U.S. Geological Survey): USGS 3D Elevation Program Digital Elevation Model, https://elevation.nationalmap.gov/arcgis/rest/services/3DEPElevation/ImageServer (last access: 1 February 2025), 2019.
Vanmaercke, M., Panagos, P., Vanwalleghem, T., Hayas, A., Foerster, S., Borrelli, P., Rossi, M., Torri, D., Casali, J., Borselli, L., and Vigiak, O.: Measuring, modelling and managing gully erosion at large scales: A state of the art, Earth-Sci. Rev., 218, 103637, https://doi.org/10.1016/j.earscirev.2021.103637, 2021.
Williams, J. R. and Berndt, H. D.: Sediment yield prediction based on watershed hydrology, T. ASAE, 20, 1100–1104, 1977.
Wu, J., Nunes, J. P., Baartman, J. E., and Urbina, C. F.: Testing the impacts of wildfire on hydrological and sediment response using the OpenLISEM model. Part 1: Calibration and evaluation for a burned Mediterranean forest catchment, Catena, 207, 105658, https://doi.org/10.1016/j.catena.2021.105658, 2021.
Youberg, A. M., Loverich, J. B., Kellogg, M. J., and Fuller, J. E.: Before the fire: Assessing post-wildfire flooding and debris-flow hazards for pre-disaster mitigation, Nat. Hazards Earth Syst. Sci. Discuss. [preprint], https://doi.org/10.5194/nhess-2019-74, 2019.