the Creative Commons Attribution 4.0 License.
the Creative Commons Attribution 4.0 License.
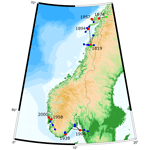
Earthquake-induced landslides in Norway
Mathilde B. Sørensen
Torbjørn Haga
Atle Nesje
Norway is located in an intraplate setting with low-to-moderate seismicity. The mountainous landscape leads to a high level of landside activity throughout the country. Earthquake-induced landslides (EQILs) are common in seismically active areas, but there are only a few studies of EQILs in intraplate regions. We systematically analyse all earthquakes in Norway with magnitudes ≥ 4.5 in the time period 1800–2021 CE. For each event we search for reports of EQILs in the available macroseismic data and in the Norwegian landslide database. We furthermore consider precipitation data from the Norwegian Centre for Climate Services to evaluate the role of precipitation in the triggering of the identified potential EQILs. Through this approach, we identify 22 EQILs that have been triggered by eight earthquakes in the magnitude range 4.5–5.9. The events are widely distributed in northern and southern Norway. The maximum landslide distance limits and landslide-affected areas are much larger than those found in empirical studies of global datasets and are in agreement with data from other intraplate regions. For four of the earthquakes, it seems that landslide triggering was due to a combined effect of precipitation and earthquake ground shaking. Our observations confirm that intraplate earthquakes have the potential to trigger EQILs over large distances, most likely due to the low ground motion attenuation in such regions. Slope susceptibility seems to be another important factor in the triggering. Our conclusions demonstrate the importance of considering EQIL potential in earthquake risk management in intraplate regions.
- Article
(10253 KB) - Full-text XML
- BibTeX
- EndNote
Norway, with its steep, high-relief terrain and northern location, is prone to all types of slope failures with rockslides, snow avalanches and debris slides being the most common. In the historic past, 4475 people have been killed by such slope failures and their secondary effects (such as collapsing landslide dams and landslide tsunamis) in Norway (Hermanns et al., 2012). The vast majority of slope failures in Norway are triggered by precipitation or snowmelt, while earthquakes, as in many intraplate settings, have not been considered a relevant trigger mechanism. There are several examples from international studies demonstrating that there is a potential for earthquake-induced landslides (EQILs) in intraplate settings as well (e.g. Keefer, 2002; Jibson and Harp, 2012), but the characteristics of intraplate EQILs, e.g. in terms of maximum epicentral distances of induced landslides or the size of the EQIL-affected area, are still poorly understood. Also in Norway there are a few reports of EQILs in the literature (e.g. Mäntyniemi et al., 2021), but such events have never been searched for systematically. In this study we perform such a systematic search to establish a list of landslides induced by earthquakes with magnitudes M ≥ 4.5 in Norway and contribute to the general understanding of EQILs in intraplate areas.
We first describe what is currently known about EQILs with a special focus on intraplate areas and provide a brief outline of the seismicity and landslide activity in Norway. We then describe the methods and data used for our systematic search for EQILs before presenting the results for the individual events. Finally, we discuss our results in relation to studies from other intraplate regions.
1.1 Earthquake-induced landslide studies
Several studies of EQILs have been published over the last few decades, based on global, regional or local datasets, but mostly focusing on areas with high seismicity (e.g. Bommer and Rodriguez, 2002; Delgado et al., 2011a; Hancox et al., 2002; Jibson and Tanyas, 2020; Keefer, 1984, 2002; Papadopoulos and Plessa, 2000; Prestininzi and Romeo, 2000; Rodriguez et al., 1999; Tadard et al., 2010). In his pioneering study, Keefer (1984) worked on a global dataset of EQILs triggered by 40 earthquakes to determine minimum magnitudes for landslide triggering as well as relations between earthquake magnitude and landslide area and maximum epicentral and fault distance to EQILs for different categories of landslides (disrupted, coherent, and lateral spreads and flows). He suggested that differences in attenuation have little effect on the area of triggered landslides; however, his database contains only very few events from intraplate areas and/or areas with low attenuation. Keefer's (1984) study has been updated, including more data from more diverse tectonic areas, in a number of publications (e.g. Delgado et al., 2011a; Keefer, 2002; Rodriguez et al., 1999). Delgado et al. (2011a) study a database of EQILs triggered by 270 earthquakes, 150 of which are considered to have high-quality data. They find that most events follow the EQIL areas and distance limits suggested by Keefer (1984) but that there are outliers, mostly disrupted or coherent landslides triggered by earthquakes in the low-to-moderate magnitude range.
In the last decade there have been several studies focusing on landslide triggering in the outermost distance limits (e.g. Jibson and Harp, 2012, 2016; Wistuba et al., 2018). Such extreme triggering is most often observed in intraplate areas with low ground motion attenuation and high slope susceptibility. Events that are well known to have triggered landslides at large distances include the 1988 Saguenay (M = 5.8) earthquake in Canada (Lefebvre et al., 1992); four earthquakes in the Colorado Plateau between 1988–1994 with magnitudes in the range 4.6–5.7 (Keefer, 2002); and the 2011 Mineral, Virginia (M = 5.8), earthquake (Jibson and Harp, 2012). The 2011 Mineral, Virginia, earthquake triggered landslides at distances up to 245 km. Jibson and Harp (2012) suggest that, in addition to the low level of ground motion attenuation, a factor explaining the long EQIL distance limit may be the detailed level of investigation for this and other recent events.
Delgado et al. (2011b) study EQILs in southern Spain and find that whereas landslide distance limits are within the limits of Keefer (1984) for larger events, the smaller events (M ≤ 5) tend to trigger landslides at larger distances than predicted by Keefer (1984). The authors conclude that slope susceptibility is an important factor for such small events. Tatard et al. (2010), analysing landslide triggering by precipitation and earthquakes in six different regions, also emphasise that the readiness of a slope for failure is an important factor in EQIL triggering. A few studies have evaluated the potential for combined triggering of landslides by earthquakes and water, indicating that long-distance EQIL triggering often occurs when slopes are water saturated, e.g. due to intense rainfall or snowmelt (e.g. Hancox et al., 2002; Frigerio Porta et al., 2021; Sassa et al., 2007).
The vast majority of EQIL studies have focused on EQILs triggered by large earthquakes in active tectonic areas. The studies described above are starting to shed some light on EQIL triggering in areas of moderate-to-low seismicity, but available data are still very limited, and there is a continued need for expanding the dataset to better understand the properties and causes of EQILs at large distances from smaller earthquakes in intraplate areas.
1.2 Seismicity of Norway
The Norwegian mainland is located far from active plate boundaries, and the level of seismicity is low to moderate. Small earthquakes (M < 4.0) occur regularly, while larger events occur at intervals of several years. Due to the stable continental setting of Norway, ground motion attenuation is low, and earthquakes of a given magnitude will cause ground shaking over larger areas than what is seen in more tectonically active regions. The largest historical earthquake in mainland Norway occurred on 31 August 1819 in Nordland, northern Norway, with a magnitude of 5.9. This event triggered several rockfalls in the epicentral area (Mäntyniemi et al., 2020). The event was widely felt in Norway, Sweden, Finland and northern Russia and caused some damage in the epicentral area. In total, the Norwegian National Seismic Network (NNSN; http://www.skjelv.no, last access: March 2023) has reported 21 earthquakes near the Norwegian mainland with M ≥ 5.0 since 1900. Most of those events are located offshore.
Figure 1 shows an overview of earthquakes recorded by the NNSN with a minimum magnitude of 2.0 in the period 1980–2021 CE. In addition to the plate boundary seismicity along the northern mid-Atlantic ridge, there is a high level of seismicity in Nordland, in northwestern Norway, along graben structures and the continental slope in the North Sea, as well as in parts of Svalbard. There is a moderate level of seismicity along the Oslo graben in eastern Norway and in Skagerrak. Most Norwegian earthquakes are at a 10–15 km depth. Several studies (e.g. Fejerskov and Lindholm, 2000; Fjeldskaar et al., 2000; Olesen et al., 2013) discuss neotectonic deformation and stress-generating mechanisms in Norway. There is general agreement that the present-day stress field originates from a combination of the ridge push force from the mid-Atlantic ridge, sediment loading at the continental shelf, glacial uplift, density contrasts in rocks, and local forces like topography and bedrock geology (Fejerskov and Lindholm, 2000). The relative importance of those mechanisms for the stress generation is, however, still an area of active research.
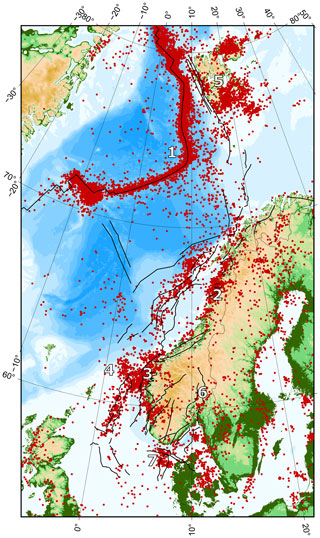
Figure 1Earthquakes with M ≥ 2.0 recorded by the NNSN in the period 1980–2021 CE. Black lines are significant faults as mapped by Smirnova (2019). Numbers mark the most seismically active areas: 1: the northern mid-Atlantic ridge, 2: Nordland, 3: northwestern Norway, 4: the North Sea, 5: Svalbard, 6: the Oslo graben and 7: Skagerrak.
Information about palaeoseismic events in Norway is scarce, and such events are usually identified due to widespread simultaneous landslides observed in geological data. During the last deglaciation (∼ 15 000–9000 yr cal BP), rapid melting of the continental ice sheet and the associated isostatic uplift led to a high level of seismicity in Norway. It is assumed that earthquakes on postglacial faults may have triggered rock avalanches along fjord areas after the last deglaciation (Blikra et al., 2006). Since then, the seismicity rate and rate of isostatic uplift have decreased. The postglacial uplift in Norway is presently up to 4 mm yr−1, increasing from west to east. Isostatic uplift is over the last centuries considered to be a minor mechanism for earthquake generation in Norway (Olesen et al., 2013). Bellwald et al. (2019) systematically compile Holocene mass transport deposits identified in seismic data and sediment cores in west and mid-Norway and evaluate their trigger mechanisms. Assuming that most of the observed mass movements are earthquake-triggered, they suggest enhanced seismic (and EQIL) activity during 11 000–9700, 8300–7700 and in the last 4200 yr cal BP with a period of seismic quiescence during the mid-Holocene. They estimate a recurrence rate of years for events (single earthquakes or clusters) large enough to trigger widespread landslides in the late Holocene. Mangerud et al. (2018) study a sediment sequence at Ringsaker, eastern Norway, with the purpose of determining the age of deglaciation in the region. They identify a major debris-flow deposit and argue, because of the very gentle slopes in the area, that it must be earthquake-triggered, indicating the occurrence of a major earthquake in the region after 6360 yr cal BP.
1.3 Landslides in Norway and their trigger mechanisms
The Norwegian landscape, with deep fjords and steep valleys, has developed through cycles of glaciations and interglacials over the last 2.58 million years. The western and northern parts of Norway are characterised by steep mountains and valley sides, whereas eastern and central Norway are dominated by more low-relief topography with thicker and more continuous sediment sequences. The landscape-forming processes, in particular during the Quaternary period, have made Norway prone to landslides of almost any type.
Rockfalls and rockslides are the most frequent types of landslides in Norway, which regularly cause damage to roads and other infrastructure (Jaedicke et al., 2009). Rock avalanches are much rarer but have widespread damage potential, especially if runout is into a lake or fjord with the potential to generate a tsunami. Since 1900, Norway has been exposed to three catastrophic tsunamis caused by rock avalanches in 1905, 1934 and 1936, killing a total of 175 people (Hermanns et al., 2012). Various types of soil failures (e.g. debris flows, debris avalanches) are also common in Norway, leading to frequent damage and loss of lives (e.g. Hermanns et al., 2012). Typical trigger mechanisms for landslides in rock and soil in Norway are long-term or heavy rainfall or rapid snow melting, leading to high water content in the bedrock and/or sediment cover. Other triggering factors include various types of weathering, erosion and seismic activity (Høeg et al., 2014).
Landslides in clayey soils and quick clay slides are most common in south-eastern and mid-Norway due to the thick clay sequences that were mainly deposited during the last deglaciation (Hermanns et al., 2012). Trigger mechanisms of (quick) clay slides include both natural causes (e.g. river erosion or long-term or heavy rainfall) and human causes (e.g. construction work).
There are only very few documented cases of modern EQILs in Norway, and those have been published in connection with seismological studies of large earthquakes. Mäntyniemi et al. (2020) present reports of several EQILs in connection with the 1819 M 5.9 Lurøy earthquake. Mäntyniemi et al. (2021) furthermore summarise environmental effects, including EQILs, triggered by the 1904 M 5.4 Oslofjord earthquake.
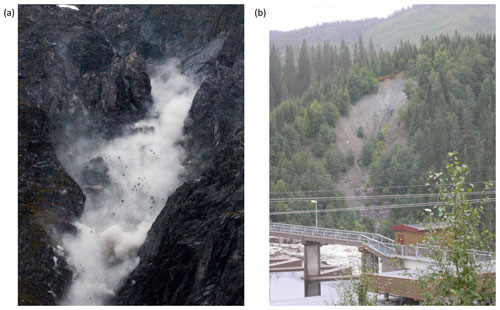
Figure 2Typical examples of (a) a rockfall (photo: Vegard Lødøen) and (b) a landslide in clayey soil (photo: Rune Halvorsen), which are the landslide typologies most commonly triggered by earthquakes in Norway.
Figure 2 shows typical examples of a rockfall and a landslide in clayey soil, which will be shown to be the landslide typologies most commonly triggered by earthquakes in Norway.
In order to systematically search for EQILs in Norway, we searched through available datasets of earthquake and landslide information, looking for events that are co-located in time and space. We describe the approach for the event search here; the individual datasets are described in detail in Sect. 3.
We extracted earthquakes with M ≥ 4.5 from the earthquake database of the Norwegian National Seismic Network (NNSN). For each event, we then searched for reports of triggered landslides in the macroseismic archives at the University of Bergen (UiB) and the Scandinavian Earthquake Archive (SEA). We also searched the Norwegian landslide database (NLD) for events coinciding in time and location with the earthquakes, as well as for events in the database that were mentioned in the commentary to be associated with earthquakes.
For each earthquake–landslide match, we critically evaluated the likelihood of the landslide being triggered by the earthquake. In many cases, it was evident from the descriptions that there was a triggering relation, and in other cases the link was more uncertain. We excluded cases where descriptions were speculative, if it was uncertain if the ground shaking described was due to an earthquake or the landslide itself, or if the landslide was triggered several days after the earthquake. Some examples are given in Sect. 4. A minimum requirement for including events in our list of Norwegian EQILs is that we have (at least approximate) locations of both the landslide and the earthquake triggering it, as well as an estimate of the earthquake magnitude. In practice that means that we need independent reports of the earthquake, as a few landslide reports are usually insufficient to determine the location and magnitude of an earthquake.
The most important trigger mechanism of landslides in Norway is precipitation, and even for landslides that are considered earthquake-induced, precipitation prior to the earthquake may have made slopes more prone to failure. In order to evaluate the relative importance of precipitation and ground shaking in the triggering of events, we downloaded precipitation data for the 30 d period before each triggered landslide from the Norwegian Centre for Climate Services and discussed the observations in relation to the triggered landslides and landslide susceptibility maps for the affected areas.
Earthquake information was extracted from the openly available earthquake database of the NNSN. The dataset is based on data processing at UiB considering data from the NNSN, currently 42 stations in mainland Norway and the Norwegian Arctic islands, as well as data from stations in the surrounding countries. We extracted all earthquakes with M ≥ 4.5 (considering here only Ml and Mw) at or near the Norwegian mainland in the time period 1800–2021 CE. Whereas smaller events may trigger landslides as well, we selected the lower magnitude threshold of M = 4.5 in order to have a systematic overview of the landslide-triggering-potential of earthquakes nationally. The study area was defined as the Norwegian mainland, including nearby parts of the North Sea, Sweden, Denmark and Finland (polygon in Fig. 3). Within this region, we found 78 earthquakes with M ≥ 4.5 in the time period 1800–2021 CE, as presented in Fig. 3 and Appendix A.
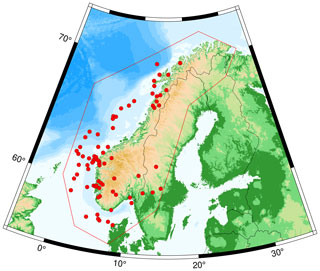
Figure 3Earthquakes with M ≥ 4.5 near the Norwegian mainland in the period 1800–2021 CE, which are checked for reports of EQILs. The red polygon outlines the area within which the earthquake search was performed.
The macroseismic archive at UiB contains earthquake information for Norway for the last 2 centuries. The archive contains systematically collected letters, questionnaires, newspaper articles and other reports of felt earthquakes; for many events several hundred pages of materials are available. For some events, additional information (newspaper articles, macroseismic intensity maps, etc.) is available through the Scandinavian Earthquake Archive (SEA; ICG, 2003). For all events in Fig. 3, the available materials in the macroseismic archive and the SEA were read to check for reports of triggered landslides.
The Norwegian landslide database (NLD) is maintained by the Norwegian Water Resources and Energy Directorate (NVE) and provides the most comprehensive listing of Norwegian landslides, with more than 80 000 events currently listed. Despite the high number of events, the database is not complete, and the precision and level of detail in the information included vary significantly among the events. It is important to note that the Norwegian term for landslide (skred) also contains snow avalanches, as opposed to all other languages (e.g. Hermanns et al., 2012; Herrera et al., 2018). The total numbers listed in this paper thus refer to landslides and snow avalanches combined. However, in this study we have not identified any snow avalanches triggered by earthquakes.
Landslide susceptibility maps are available for all of Norway through the NVE Atlas (https://atlas.nve.no, last access: March 2023). The NVE Atlas presents susceptibility maps for snow avalanches, rockfalls, and soil and debris flows in three separate map layers. The maps confirm the widespread potential for all types of landslides throughout the country. Susceptibility maps for quick clay slides are also available through the NVE Atlas and in more detail in the maps from the Norwegian Geological Survey displaying “possibility of marine clay (MML)” in their national database of superficial deposits (https://geo.ngu.no/kart/losmasse_mobil/, last access: March 2023). None of the available susceptibility maps consider the potential for earthquakes as a landslide trigger.
For each of the 78 earthquakes with M ≥ 4.5, we searched the NLD for landslides occurring within 5 d and 250 km of the earthquake. The time and distance limits were selected as plausible maxima based on previous observations (e.g. Delgado et al., 2011a; Jibson and Harp, 2012; Keefer, 2002) and considering that landslides occurring outside those limits would be difficult to directly associate with the considered earthquakes. We also made a text search for events with the word “earthquake” (jordskjelv in Norwegian) in the commentary. That was the case for 23 events. For each of the identified landslides we then evaluated whether the spatial and temporal links, as well as the information provided in the commentary, were sufficient to indicate a triggering relation.
Precipitation data were downloaded from the Norwegian Centre for Climate Services who offer observations from weather stations throughout Norway through the online platform Seklima (http://www.seklima.met.no, last access: March 2023). For each earthquake (when available), 24 h averaged precipitation data were downloaded for the 30 d period prior to the event and visually inspected to evaluate the potential role of precipitation in landslide triggering.
Through the systematic search of available earthquake and landslide data we identified 22 EQILs that were triggered by eight different earthquakes in Norway in the period 1800–2021. The events are presented in Fig. 4, with details on locations and epicentral distances given in Table 1. We discuss each event and the background data in the following sections.
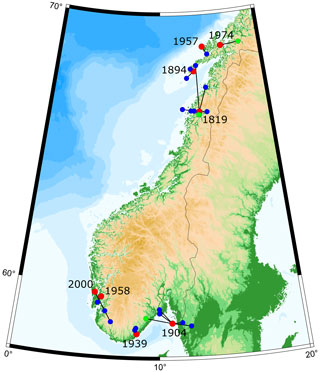
Figure 4Norwegian EQILs identified in this study. Red dots are earthquake locations, blue dots are rock falls and green dots are landslides in clayey soil. Lines connect each EQIL to the triggering earthquake.
Table 1Earthquake-induced landslides in Norway identified in this study. Magnitudes are local magnitude (Ml), surface wave magnitude (Ms) or moment magnitude (Mw).
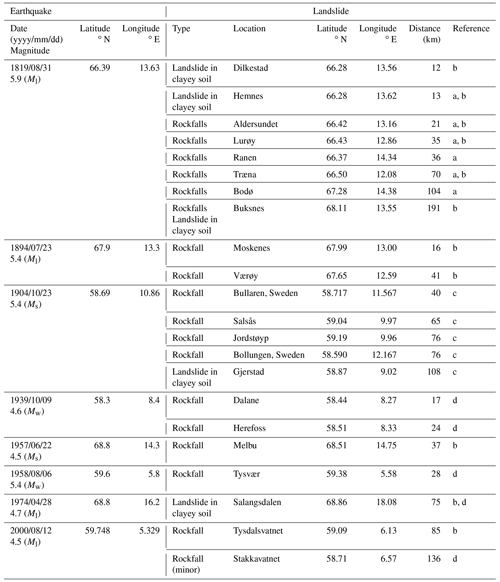
References: a Mäntyniemi et al. (2020), b the NLD, c Mäntyniemi et al. (2021) and d the macroseismic archive at UiB.
4.1 The 31 August 1819 Lurøy, Nordland, earthquake
The 1819 Lurøy earthquake is the largest earthquake in Fennoscandia in historical times. Several studies (e.g. Muir Wood, 1988; Bungum and Olesen, 2004; Mäntyniemi et al., 2020) have analysed the macroseismic reports of the event to determine its location and magnitude; most recently Mäntyniemi et al. (2020) reassessed intensities, including also a number of previously unknown observations, and found a magnitude of 5.9 ± 0.2 for the event. The largest observed intensity was VIII. It is well documented in the previous studies that this event triggered several landslides. The location of the event has never been evaluated using state-of-the-art methods. Muir Wood (1988) lists a location of the event near Mo i Rana (66.4∘ N, 14.4∘ E), which is to the east of the area experiencing the strongest effects of the earthquake. It is outside the scope of this study to perform an in-depth evaluation of the location of this earthquake, but in order to have more reliable landslide distance limits, the event was relocated with the BOXER code (Gasperini et al., 1999, 2010) using the intensities assigned by Mäntyniemi et al. (2020). We thereby obtained a location (66.39∘ N, 13.63∘ E) that is in better agreement with the macroseismic observations. We will use this new location for the event in this study.
The macroseismic reports of the earthquake have already been thoroughly analysed and presented in previous studies. Reports from the epicentral area are mostly available in contemporary newspapers, describing very strong shaking and minor-to-moderate damages (see Mäntyniemi et al., 2020, for a detailed description, including English translations of all reports).
Table 2Landslides reported in connection with the 1819 Lurøy earthquake. Locations are from Mäntyniemi et al. (2020) if not stated otherwise. Epicentral distance is relative to the BOXER location (66.39∘ N, 13.63∘ E). NLD: Norwegian landslide database.
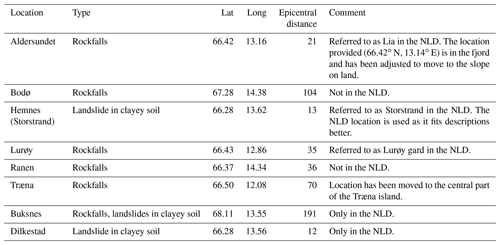
In this study, we used the macroseismic reports with associated coordinates listed by Mäntyniemi et al. (2020) as a starting point. We quality checked the locations of reported landslides and moved some to more meaningful locations (see comments in Table 2 for details). The NLD contains some of the reported landslides and lists two additional landslides that are not mentioned by Mäntyniemi et al. (2020). There are several reports of rockfalls and landslides in clayey soil causing damage to the soil and farmland. There are some mentions of houses being almost hit by falling rocks. A summary of the landslides that were identified in connection to this earthquake is given in Table 2, and the locations are presented in Fig. 5. We find at least eight landslides that were triggered by the 1819 earthquake at distances up to 191 km from the epicentre.
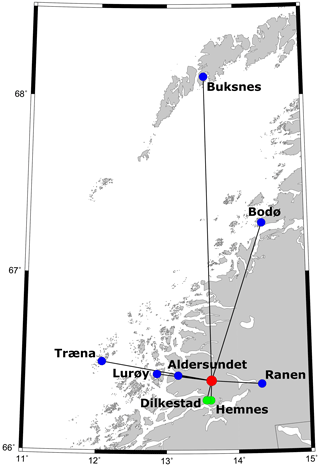
Figure 5Landslides triggered by the 1819 Lurøy earthquake. The red dot is the epicentre of the event, blue dots are rockfalls, and green dots are landslides in clayey soils.
Consulting the NVE Atlas for landside susceptibility, we found that the landslides observed in Aldersundet and Lurøy are in areas with widespread susceptibility to slope failures in snow, rock and soil. Also near Diklestad and Hemnes there are several areas exposed to various types of landslides. However, the EQILs observed in Ranen, Træna, Bodø and Buksnes all occurred in areas where only limited susceptibility to e.g. rockfalls has been identified. The MML maps of the Norwegian Geological Survey show that marine clays may potentially be a problem around Bodø. The remaining EQIL locations are not covered by the available maps.
There is no precipitation data available for the region at the time of the earthquake, but several macroseismic reports mention that the earthquake happened after a 3-week period of rainy weather. In that regard, water saturation of the slopes is expected to have contributed to their instability. However, based on the magnitude of the earthquake and the descriptions available, the earthquake is expected to be the main trigger of the reported landslides.
4.2 The 23 July 1894 Lofoten earthquake
The 1894 earthquake was located near the Lofoten islands in northern Norway with a magnitude of Mw = 5.4. The event was felt in the Nordland district with a maximum intensity of VII and also over large parts of central Norway. The NLD reports that several large rockfalls occurred at the Moskenes and Værøy islands in Lofoten in direct connection with the earthquake. The maximum distance to the triggered landslides is 41 km. Both islands are mapped as highly susceptible to rockfalls in the NVE Atlas. There is no weather data available from the Lofoten area at that time, and it is thus not possible to evaluate the role of precipitation in triggering the rockslides. It is considered highly likely that the earthquake played a significant role in triggering the rockfalls.
4.3 The 23 October 1904 Oslofjord earthquake
The 1904 Oslofjord earthquake is also a well-known and thoroughly studied Norwegian earthquake due to its location close to the capital city of Oslo. It occurred on a Sunday morning during church hours and was strongly felt, causing some panic in Oslo but only minor damages. The event had a magnitude of 5.4 and was located at 58.69∘ N, 10.86∘ E (Bungum et al., 2009), and the maximum observed intensity was VII. Environmental effects caused by the earthquake, including induced landslides, were recently summarised by Mäntyniemi et al. (2021). We base our analysis on those findings.
The landslides induced by the 1904 earthquake are presented in Table 1 and Fig. 4. In Norway, the NLD reports two rockfalls at Salsås and Jordstøyp near Larvik, mentioning that more slides occurred in the same area. An 18–20 ft (corresponding to about 6 m) wide landslide in clayey soil in a mixture of sawdust and sand was reported by Kolderup (1905) to have occurred along a riverbank near Gjerstad. In Sweden there are reports of two rockfalls near Bullaren and Bollungen. Rockfall activity is reported to have continued near Bullaren in the days after the earthquake, culminating in a large landslide during the night between 29–30 October, 6 d after the earthquake. There are also reports of a land subsidence near Väddö, 25 km from the earthquake (Mäntyniemi et al., 2021), but this event has not been included in the list, as it is not a fully developed landslide. We thus list five landslides triggered by the 1904 earthquake, at a maximum distance of 108 km.
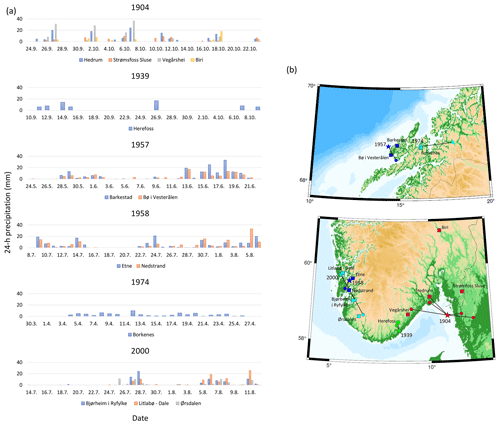
Figure 6(a) Precipitation observed 30 d before the earthquakes inducing landslides. (b) Locations of the weather stations shown in (a) (squares), earthquake epicentres (stars) and EQILs (circles). Lines connect EQILs to the epicentre of their triggering earthquake. Colours are the same for data referring to a given earthquake.
Salsås and Jordstøyp are both in areas that are susceptible to rockfalls at selected slopes, according to the NVE Atlas. Figure 6 presents precipitation data from four weather stations located around the epicentral area, near the observed landslides. Some precipitation is observed during the 30 d period before the event, especially in the western part of the affected area, but the total rainfall is not extreme. There was relatively little precipitation in the area on the days prior to the earthquake, supporting the earthquake being the main trigger of the slides.
In addition to the landslides listed above, there are reports in the NLD of a large rockfall crossing the railway passing through Etnedal on 25 October 1904, 2 d after the large earthquake, at an approximately 230 km distance. It is mentioned that aftershocks had been strongly felt in the area on that day, and it is speculated that the landslide is earthquake-triggered. The precipitation data (Fig. 6) show that there was significant rainfall on 25 October (18 mm in 24 h at station Biri near Etnedal) that may have contributed to triggering the rockfall. We find the triggering relation is uncertain, a combined effect of precipitation and ground shaking is likely, and the event is not included in our list.
4.4 The 9 October 1939 Lillesand earthquake
The 1939 earthquake is listed in the NNSN database with Mw = 4.6 and a location near Lillesand between Kristiansand and Arendal. The event was felt over a large area in southern Norway with a maximum intensity of V. In one questionnaire in the macroseismic archive at UiB, which is accompanied by a letter describing the observations in more detail, it is reported that at Dalane in Herefoss, the earthquake was clearly felt. On the day after the earthquake, on 10 October 1939, a rockfall was observed 300–400 m from the houses at Dalane, at a 17 km distance from the earthquake. Large blocks are reported to have fallen down, one of them the size of a house. An approximately 70 m long stretch of forest along the slope is reported to have been destroyed. There are also reports of rocks falling down on the road along the western shore of Herefossfjorden, between Odden and Herefoss Gård in Herefoss, at a ca. 24 km distance from the earthquake epicentre. There are no reports in the NLD of landslides in connection with this earthquake.
Dalane is in a valley with high susceptibility to rockfalls, according to the NVE Atlas. The western part of Herefossfjorden, on the other hand, only experiences very limited rockslide susceptibility. Precipitation data from the 30 d period before the event at the meteorological station in Herefoss are presented in Fig. 6. The weeks prior to the earthquake were relatively dry; 7.8 mm rainfall was observed on 7 October and 5.9 mm was observed on the day of the earthquake. Whereas that may have increased the susceptibility of the slope, it is considered insufficient to argue against the earthquake being the main trigger.
4.5 The 22 June 1957 Vesterålen earthquake
The 1957 earthquake was located just offshore Langøya in Vesterålen and had a magnitude Ml = 4.6 and a maximum intensity of V. The event was felt throughout Lofoten and Vesterålen. The NLD reports a rockfall close to Melbu with a very large block landing only about 20 m from a house. It is mentioned that there were several rockfalls in connection with the earthquake, but no further information is given on their locations. Melbu is a 37 km distance from the earthquake epicentre. There are no reports of EQILs in the macroseismic archive.
The slopes around Melbu are marked as susceptible to rockfalls in the NVE Atlas. Figure 6 shows precipitation data from two stations on Langøya, at similar distances from the earthquake. There was no precipitation on the day of the earthquake, but it happened after a period of about a week of rainy weather. The ground is thus expected to have been water saturated, making it more prone to failure. However, the amount of rainfall is not extraordinary, and several rockfalls were observed; therefore the earthquake is expected to have had a major role in triggering the rockfalls.
4.6 The 6 August 1958 Ølen earthquake
On 6 August 1958, a magnitude Mw = 5.4 earthquake occurred near Ølen in southwestern Norway. The event was felt throughout southern Norway with a maximum observed intensity of V–VI. The macroseismic archive at UiB contains a newspaper article from the local newspaper Haugesund Dagblad stating that a large rockfall occurred in Tysvær on the day after the earthquake and speculating that the earthquake was the trigger. The distance from Ølen to Tysvær is 28 km. There are no reports of landslides in connection with the earthquake in the NLD.
The location of the landslide is uncertain, but according to the NVE Atlas, only isolated slopes in the area are exposed to rockfalls. Precipitation data from surrounding weather stations (Fig. 6) show that the rockfall occurred after several days of rainy weather. The amount of precipitation is not extraordinary, but slopes are expected to have been water saturated and thus more prone to failure. We expect that this rockfall was triggered by a combination of precipitation and ground shaking. We choose to include the event in our list of EQILs, noting the uncertainty and limited information available.
4.7 The 28 April 1974 Kvæfjorden earthquake
The 1974 earthquake was located near Kvæfjorden in northern Norway with a magnitude of Mw = 4.9. The event was felt throughout the Nordland region with a maximum intensity of V. Both the macroseismic archive and the NLD contain reports of a large landslide in clayey soil that was triggered in Salangsdalen, at a 75 km distance from the epicentre, immediately after the earthquake. The slide temporarily dammed the river Salangselven, leading to flooding of the road running along the river. The NLD also lists a debris slide very close to the slide without any description of the event. Due to the proximity of the two events and the lack of information, the debris slide has not been included as a separate event, but it is expected that several slope failures have occurred along the Salangsdalen valley in connection with the earthquake.
According to the NVE Atlas, only very small areas along the Salangsdalen valley are susceptible to soil slides. There is a high risk of marine clays in the entire area, according to the MML database of the Norwegian Geological Survey. Precipitation data from the area during the 30 d period before the event (Fig. 6) show that there had been a steady, low level of precipitation before the earthquake without any intense precipitation event. This supports the hypothesis of the earthquake being the main trigger of the landslides.
4.8 The 12 August 2000 Stord/Bømlo earthquake
The 2000 earthquake was located in the Stord/Bømlo area. The event had a magnitude of Ml = 4.5 and was strongly felt in western Norway with a maximum intensity of V. The NLD contains a report of a rockfall near Tysdalsvatnet, at a 85 km distance from the epicentre, noting that the rockfall was most likely triggered by the earthquake. The macroseismic archive contains a questionnaire where the respondent describes a minor rockfall in the mountain near a tourist cabin by Stakkavatnet, at a distance of 136 km from the epicentre.
According to the NVE Atlas, the slopes surrounding Tysdalsvatnet are highly susceptible to rockfalls and soil slides. Also around Stakkavatnet, there are several rockfall-susceptible slopes. Precipitation data (Fig. 6) show that there were several periods of moderate rainfall before the landslide, which may have made the slope more prone to failure but is not considered sufficient to trigger the slide. We thus conclude that the earthquake was the main trigger of the rockfall.
4.9 Events with little documentation
In addition to the events listed above, we have found reports of EQILs that are sufficient to confirm that an EQIL was induced but insufficient to locate the landslide and/or the earthquake. There are also reports of landslides occurring in connection with earthquakes where the documentation is insufficient to conclude that the landslide was earthquake-induced. Those cases are described in the following.
4.9.1 The 15 May 1892 Askvoll earthquake
A large earthquake occurred on 15 May 1892 near Askvoll in western Norway (61.4∘ N, 5.1∘ E). The event is listed in the NNSN database with Mw = 5.7 and was felt throughout southern and parts of central Norway with a maximum intensity of VI. The NLD lists a landslide in clayey soil at Bakklandet, Trondheim (63.43∘ N, 10.40∘ E), on 19 May, 4 d after the earthquake. The NLD refers to an article in the newspaper Trondhjems Adresseavis which speculates that the earthquake may have caused cracks in the clay that have later developed into the landslide. The distance between the earthquake epicentre and the landslide is 350 km. According to the macroseismic reports, the earthquake was weakly felt (I = III) in Trondheim. Precipitation data show that about 10 mm of rainfall was recorded in Trondheim during the days between the earthquake and the landslide. This is not expected to have significantly affected the stability of the clays. We consider it likely that the earthquake has played a role in triggering the landslide. However, due to the long time and distance between the events, and as cracks following the earthquake are not documented, we choose not to include the event in our list of EQILs in Norway.
4.9.2 The 1932 event
The NLD contains a report of a rockfall near Sautso in northern Norway in 1932. The time of year is unknown. According to the NLD report, three people had observed the rockfall immediately after a large earthquake. There are no reports in the macroseismic archives of earthquakes in northern Norway in 1932. The only potential event that can be linked to the rockfall is an event on 14 April 1932 that is briefly mentioned in the SEA to have been felt on the island Bjørnøya, about 500 km north of the landslide location. We consider it likely that the rockfall was earthquake-triggered but cannot include the event in our list of Norwegian EQILs due to a lack of information on the earthquake.
The EQILs and their triggering earthquakes are widely distributed in southern and northern Norway (Fig. 4). The events in northern Norway are in one of the most seismically active areas in Norway. The seismicity level in southern Norway is lower, but with a higher population density it is more likely that EQIL events are detected and reported. It is somewhat surprising that there are no EQILs in the central parts of the country, especially in western and north-west Norway where seismicity is high and steep slopes abundant. However, this may be linked to a lower population density in those areas, and it is expected that EQILs have indeed occurred, though have not been reported in the sources studied here.
The landslide distance limits found in this study for Norway are generally much larger than what has been observed from global data (Keefer, 1984; Rodriguez et al., 1999; Delgado et al., 2011a) and fit well with the previously observed extreme distance limits from intraplate areas. Figure 7 shows the landslide distance limits found in this study (red dots) together with distance limits from other intraplate earthquakes (1988 Saguenay, Canada (Lefebvre et al., 1992); 1988–1994 Colorado Plateau, USA (Keefer, 2002); and 2011 Virginia, USA (Jibson and Harp, 2012); grey dots) and the maximum landslide distance limit curve for disrupted slides and falls obtained by Keefer (1984) (black line). The distance limits suggested by Keefer (1984) severely underestimate the landslide potential of intraplate earthquakes. For magnitudes less than 5.0, the observed intraplate landslide distance limits are up to more than an order of magnitude larger than those suggested by Keefer (1984). For larger events, the observed intraplate landslide distance limits are up to about half an order of magnitude larger.
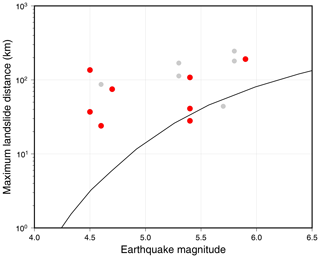
Figure 7Landslide distance limits for earthquakes in intraplate areas. Red dots: Norway (this study), grey dots: other areas (1988 Saguenay, Canada (Lefebvre et al., 1992); 1988–1994 Colorado Plateau, USA (Keefer, 2002); 2011 Virginia, USA (Jibson and Harp, 2012)) and black curve: maximum landslide distance limit for disrupted slides and falls from Keefer (1984).
For most of the landslide-triggering earthquakes identified in this study, only one or two EQILs have been identified, and it is not possible to calculate a well-constrained landslide area. The exceptions are the 1819 and 1904 earthquakes, for which eight and five EQILs, respectively, have been identified. Following Jibson and Harp (2012), we determine the landslide area as the area of a polygon containing all landslides triggered by an event. This approach will lead to a minimum estimate of the landslide-affected area. We obtain a landslide area of 12 000 km2 for the 1819 earthquake and an area of 3700 km2 for the 1904 earthquake. These values are plotted together with the landslide area of the 2011 Virginia, USA, earthquake (33 400 km2; Jibson and Harp, 2012) and the empirically derived curve of the maximum landslide area vs. distance by Rodriguez et al. (1999) in Fig. 8. Whereas the number of observations is small, they are in agreement with the systematically larger distance and area limits for landslide triggering by intraplate earthquakes.
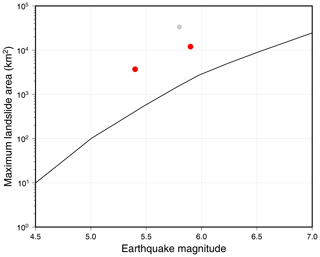
Figure 8Maximum landslide area for earthquakes in intraplate areas. Red dots: Norway (this study); grey dot: 2011 Virginia, USA, earthquake (Jibson and Harp, 2012); black curve: maximum landslide area limit from Rodriguez et al. (1999).
The EQILs identified in this study all occurred either before instrumental earthquake monitoring was established in Norway or when the seismic network consisted only of a few stations. Therefore, there are no direct recordings of ground shaking available near any of the EQILs. An alternative way of estimating the level of ground shaking at the EQIL locations is through the use of ground motion models (GMMs) linking ground shaking levels to earthquake magnitude, distance and potentially other parameters. For such GMMs to provide reliable ground motion estimates, the models must be derived from, or, as a minimum, be calibrated against regional ground motion data. Unfortunately, no such GMM based on modern approaches and data is available for Norway. We therefore do not attempt to evaluate the ground acceleration or velocity levels associated with landslide triggering as the outcome would be too uncertain to be of practical use.
A detailed map of the 1904 earthquake, with earthquake location, EQILs and intensity observations, is presented in Fig. 9. All EQILs triggered by that event are located at similar or higher latitudes than the earthquake due to the offshore location of the event. Most of the area within a plausible distance limit and south of the epicentre is covered by water, which makes landslide observations less likely. The area with the highest observed ground motion intensity (I = VII) is elongated in the WNW–ESE direction, and the distribution of observed EQILs follows this trend of the strongest ground shaking well (Fig. 9). Whereas Fig. 9 gives a rough indication of the ground shaking intensity observed near the EQIL locations for the 1904 earthquake, the available intensity data for the remaining landslide-triggering events identified in this study are insufficient to determine generalised threshold intensity levels for EQILs in Norway.
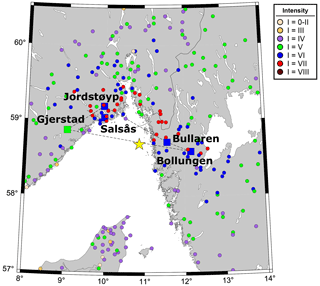
Figure 9Data available for the 23 October 1904 M 5.4 earthquake. Blue squares are rockfalls triggered by the earthquake; the green square is a landslide in clayey soil. The yellow star shows the epicentre location according to Bungum et al. (2009). Coloured dots are macroseismic intensity observations from Austegard (1975). The dotted polygon shows the landslide area determined from the observed EQILs.
From our data, as well as previous observations (Figs. 7, 8), there is little doubt that intraplate earthquakes have the potential to trigger landslides at much larger distances than plate boundary events. This is most likely due to differences in ground motion attenuation leading to stronger ground shaking over a larger area at a given magnitude for intraplate events, as has also been suggested by e.g. Jibson and Harp (2012). Jibson and Harp (2012) also suggest that differences in the levels of investigation for historical and more recent earthquakes lead to differences in the identified landslide distance limits, as recent events have been investigated more thoroughly. Whereas we agree that such differences are most likely present, our dataset is mostly based on historical or macroseismic reports and is thus directly comparable to global studies.
Earthquake depth is expected to also affect the potential for landslide triggering. Due to the coastal or offshore locations of most Norwegian earthquakes, event depths are often poorly constrained; this is especially the case for older events. We thus do not have sufficiently precise depth information on the earthquakes in our EQIL dataset to observe any depth dependence of the EQIL triggering.
It is expected that antecedent precipitation is important for the extent and distribution of EQILs, especially in intraplate areas where most earthquakes are of low-to-moderate magnitude. Whereas earthquakes are equally likely to occur at any time of the year, all the landslide-triggering earthquakes in our dataset occurred between April and October. The lack of events during the winter months may be linked to lower slope susceptibility in winter when mountain precipitation is more likely to come as snow and thus will not migrate into the ground. Figure 6 presents precipitation data for the 30 d before each earthquake, when available. The events in 1957, 1958 and 2000 occurred after periods with moderate rainfall which may have made the slopes more prone to failure. The same is the case for the 1819 earthquake that, according to the macroseismic reports, occurred after 3 weeks of intense rainfall (Mäntyniemi et al., 2020).
Most of the EQILs identified in this study occurred in areas that are mapped in the NVE Atlas as susceptible to the type of slope failure observed. However, several EQILs, especially in connection with the larger earthquakes in 1819 and 1904, were triggered in areas that are not identified in the landslide susceptibility maps. This is important as it indicates that future EQILs may occur in areas that are not prepared for landslide events.
This study is based mainly on historical reports describing landslide occurrences, mostly written by observers who happened to be at the site of the EQIL. Such reports are often vague, and we do not have information on the shapes and exact sizes of the landslides. However, based on the descriptions available in historical sources, the triggered events are mostly either rockfalls or small rockslides. This is in agreement with the expected effects of moderate-magnitude earthquakes that cause short-duration high-frequency ground shaking, which is most likely to trigger small, shallow landslides. From a hazard perspective, this means that EQIL hazard in Norway is mostly related to smaller landslide events that will have an impact over limited spatial scales.
There are no reports so far of EQILs leading to human casualties in Norway. For extremely susceptible slopes, even a moderate-size earthquake could trigger a larger landslide, but usually a large earthquake, leading to strong, low-frequency ground shaking, would be required to trigger e.g. a large rock avalanche. Keefer (1984) and Rodriguez et al. (1999) suggest that earthquakes of Ms ≥ 6.0–6.5 are required to trigger rock avalanches. No such event has been observed in Norway in the historical records, but considering that large events have been observed in other intraplate areas and the growing evidence of large palaeoseismic events in Norway (e.g. Bellwald et al., 2019; Mangerud et al., 2018), the possibility of such large events occurring in the future should not be neglected.
The dataset presented in this study is the first of its kind for Norway, but it is expected to be highly incomplete for several reasons. Many landslides in Norway occur in remote areas where many small rockfalls and landslides go unnoticed. It is therefore very likely that EQILs will not be identified or that they will be noticed long after the earthquake and therefore not identified as an EQIL. A further limitation is that much of the data considered here are from macroseismic questionnaires, where landslide observations are not specifically asked for. It is thus possible that landslides have been observed in connection with an earthquake but not reported because the respondent did not find the information relevant. Systematic investigation of remote sensing data before and after large earthquakes may allow for a more systematic mapping of EQILs in future studies. Such an approach may also allow for mapping EQILs triggered by earthquakes with smaller magnitudes than considered in this study.
In this study we have identified 22 earthquake-induced landslides (EQILs) that were triggered by eight Norwegian earthquakes of M ≥ 4.5 in the period 1800–2021 CE. Reports of the EQILs are found in macroseismic reports and in the Norwegian Landslide Database. The events are mostly rockfalls, as well as a few landslides in clayey soils, and they are distributed over large areas of northern and southern Norway.
The maximum EQIL distance limits and landslide-affected areas are consistent with observations from other intraplate areas, and a half to 1 order of magnitude larger than those predicted from empirical studies in tectonically active areas. The larger distance and area limits are most likely due to differences in ground motion attenuation, though other factors may influence those limits as well. Slope susceptibility is another important factor in the triggering of EQILs in intraplate areas, and for four of the earthquakes triggering EQILs, precipitation is expected to have increased the susceptibility of the affected slopes before the earthquake.
The EQILs are mostly small events, as one would expect considering the high frequency content and short duration of ground shaking from moderate-magnitude earthquakes. The probability of earthquakes large enough to trigger very large landslides or rock avalanches in Norway is very low, and the hazard related to EQILs is thus mostly at a local scale. However, considering the damage potential of even small rockfalls and slides, the potential for EQILs should be incorporated in natural hazard risk management in Norway.
Table A1Earthquakes with M ≥ 4.5 in the time period 1800–2021 CE within the polygon shown in Fig. 3, extracted from the NNSN earthquake database. Abbreviations: lat: latitude, long: longitude, M: magnitude, type: magnitude type, Ml: local magnitude and Mw: moment magnitude. For events with both Ml and Mw available, the largest is presented. ∗ is the magnitude derived from macroseismic data (Mäntyniemi et al., 2020).

Earthquake data used in this study are from the NNSN. The earthquake catalogue with event information is available through https://nnsn.geo.uib.no/nnsn/#/data/events/search (NNSN, 2023a). Waveform data can be downloaded through the EIDA system from https://eida.geo.uib.no/webdc3/ (NNSN, 2023b). The macroseismic archive at UiB can be accessed by contacting the lead author of this study; a scanned version of the SEA is available through https://www.geo.uib.no/seismo/REPORTS/Scandinavian_earthquake_archive/ (ICG, 2003).
The Norwegian landslide database is published and maintained by the NVE and can be accessed through their map services: https://nve.no/map-services/ (NVE, 2023).
Precipitation data used in this study can be downloaded through the Norwegian Centre for Climate Services web service: https://www.seklima.met.no (NCCS, 2023).
MBS designed the study with contributions from TH and AN. MBS and TH performed the data analysis and interpretation, and all authors discussed the results. MBS wrote the manuscript in consultation with TH and AN.
The contact author has declared that none of the authors has any competing interests.
Publisher’s note: Copernicus Publications remains neutral with regard to jurisdictional claims in published maps and institutional affiliations.
This article is part of the special issue “Earthquake-induced hazards: ground motion amplification and ground failures”. It is not associated with a conference.
We wish to thank Odd Are Jensen (NVE) for answering our questions and helping us access data from the Norwegian landslide database and Astor Furseth for sharing his collection of materials documenting EQILs in Norway. We also wish to thank Reginald Hermanns, Marta-Cristina Jurchescu and the two anonymous reviewers for their thoughtful comments that helped us improve the manuscript.
This paper was edited by Hans-Balder Havenith and reviewed by Reginald Hermanns, Marta-Cristina Jurchescu, and two anonymous referees.
Austegard, A.: The 1904-earthquake in the Oslofjord area, in: Phase 1 Studies; Preliminary Seismic Design Criteria; Five Alternative Sites around Oslofjord, for NVE Statskraftverkene, Oslo, Norway, Dames and Moore, Job No. 6777-001, 18 pp., 1975.
Bellwald, B., Hjelstuen, B. O., Sejrup, H. P, Stokowy, T., and Kuvås, J.: Holocene mass movements in west and mid-Norwegian fjords and lakes, Mar. Geol., 407, 192–212, https://doi.org/10.1016/j.margeo.2018.11.007, 2019.
Blikra, L. H., Longva, O., Braathen, A., Anda, E., Dehls, J., and Stalsberg, K.: Rock slope failures in Norwegian fjord areas: Examples, spatial distribution and temporal pattern, in: Landslides from Massive Rock Slope Failure, edited by: Evans, S. G., Mugnozza, G. S., Strom, A., and Hermanns, R. L., NATO Science Series, Springer, Dordrecht, vol. 49, https://doi.org/10.1007/978-1-4020-4037-5_26, 2006.
Bommer, J. J. and Rodríguez, C. E.: Earthquake-induced landslides in Central America, Eng. Geol., 63, 189–220, 2002.
Bungum, H. and Olesen, O.: The 31st of August 1819 Lurøy earthquake revisited, Norw. J. Geol., 85, 245–252, 2004.
Bungum, H., Pettenati, F., Schweitzer, J., Sirovich, L., and Faleide, J. I.: The 23 October 1904 Ms5.4 Oslofjord earthquake: Reanalysis based on macroseismic and instrumental data, B. Seismol. Soc. Am., 99, 2836–2854, 2009.
Delgado, J., Garrido, J., Lopez-Casado, C., Martino, S., and Pelaez, J. A.: On far field occurrence of seismically induced landslides, Eng. Geol., 123, 204–213, https://doi.org/10.1016/j.enggeo.2011.08.002, 2011a.
Delgado, J., Peláez, J. A., Tomás, R., García-Tortosa, F. J., Alfaro, P., and López Casado, C.: Seismically-induced landslides in the Betic Cordillera (S Spain), Soil Dyn. Earthq. Eng., 31, 1203–1211, 2011b.
Fejerskov, M. and Lindholm, C.: Crustal stress in and around Norway: an evaluation of stress-generating mechanisms, Geol. Soc. SP, 167, 451–467, https://doi.org/10.1144/GSL.SP.2000.167.01.19, 2000.
Fjeldskaar, W., Lindholm, C., Dehls, J. F., and Fjeldskaar, I.: Post-glacial uplift, neotectonics and seismicity in Fennoscandia, Quaternary Sci. Rev., 19, 1413–1422, 2000.
Frigerio Porta, G., Bebbington, M., Xiao, X., and Jones, G.: A Statistical Model for Earthquake And/Or Rainfall Triggered Landslides, Front. Earth Sci., 8, 605003, https://doi.org/10.3389/feart.2020.605003, 2021.
Gasperini, P., Bernardini, F., Valensise, G., and Boschi, E.: Defining seismogenic sources from historical earthquake felt reports, B. Seismol. Soc. Am., 89, 94–110, 1999.
Gasperini, P., Vannucci, G., Tripone, D., and Boschi, E.: The location and sizing of historical earthquakes using the attenuation of macroseismic intensity with distance, B. Seismol. Soc. Am., 100, 2035–2066, https://doi.org/10.1785/0120090330, 2010.
Hancox, G., Perrin, N., and Dellow, G.: Recent studies of historical earthquake-induced landsliding, ground damage, and MM intensity in New Zealand, Bulletin of the New Zealand Society for Earthquake Engineering, 35, 59–95, 2002.
Hermanns, R. L., Hansen, L., Sletten, K., Böhme, M., Bunkholt, H. S. S., Dehls, J. F., Eilertsen, R. S., Fischer, L., L'Heureux, J. S., Høgaas, F., Nordahl, B., Oppikofer, T., Rubensdotter, L., Solberg, I. L., Stalsberg, K., and Yugsi Molina, F. X.: Systematic geological mapping for landslide understanding in the Norwegian context, in Landslides and Engineered Slopes: Protecting Society through Improved Understanding, Proceedings of the 11th International & 2nd North American Symposium on Landslides, Banff, Canada, 3–8 June 2012, 265–271, ISBN 978-0-415-62123-6, 2012.
Herrera, G., Mateos, R. M., García-Davalillo, J. C., Grandjean, G., Poyiadji, E., Maftei, R., Filipciuc, T.-C., Auflič, M. J., Jež, J., and Podolszki, L., Trigila, A., Iadanza, C., Raetzo, H., Kociu, A., Przyłucka, M., Kułak, M., Sheehy, M., Pellicer, X. M., McKeown, C., Ryan, G., Kopačková, V., Frei, M., Kuhn, D., Hermanns, R. L., Koulermou, N., Smith, C. A., Engdahl, M., Buxó, P., Gonzalez, M., Dashwood, C., Reeves, H., Cigna, F., Liščák, P., Pauditš, P., Mikulėnas, V., Demir, V., Raha, M., Quental, L., Sandić, C., Fusi, B., and Jensen, O. A.: Landslide databases in the Geological Surveys of Europe, Landslides, 15, 359–379, https://doi.org/10.1007/s10346-017-0902-z, 2018.
Høeg, K., Lied, K., Karlsrud, K., and Gregory, T.: Skred: skredfare og sikringstiltak, NGI Universitetsforlag, Oslo, Norway, ISBN 9788215023915, 2014.
ICG: Scandinavian Earthquake Archive, CD with scanned reports for ICGproject No. 3, ICG 3-2003-3, ICG [data set], https://www.geo.uib.no/seismo/REPORTS/Scandinavian_earthquake_archive/ (last access: March 2023), 2003.
Jaedicke, C., Lied, K., and Kronholm, K.: Integrated database for rapid mass movements in Norway, Nat. Hazards Earth Syst. Sci., 9, 469–479, https://doi.org/10.5194/nhess-9-469-2009, 2009.
Jibson, R. W. and Harp, E. L.: Extraordinary distance limits of landslides triggered by the 2011 Mineral, Virginia, earthquake, B. Seismol. Soc. Am., 102, 2368–2377, https://doi.org/10.1785/0120120055, 2012.
Jibson, R. W. and Harp, E. L.: Ground Motions at the Outermost Limits of Seismically Triggered Landslides, B. Seismol. Soc. Am., 106, 708–719, https://doi.org/10.1785/0120150141, 2016.
Jibson, R. W. and Tanyas, H.: The influence of frequency and duration of seismic ground motion on the size of triggered landslides – A regional view, Eng. Geol., 273, 105671, https://doi.org/10.1016/j.enggeo.2020.105671, 2020.
Keefer, D. K.: Landslides caused by earthquakes, Geol. Soc. Am. Bull., 95, 406–421, 1984.
Keefer, D. K.: Investigating landslides caused by earthquakes – a historical review, Surv. Geophys., 23, 473–510, 2002.
Kolderup, C. F.: Jordskjælvet den 23. Oktober 1904, Bergens Mus. Aarbok, 1, 172, 1905.
Lefebvre, G., Leboeuf, D., and Hornych, P.: Slope failures associated with the Saguenay earthquake, Quebec, Canada, Can. Geotech. J., 29, 117–130, 1992.
Mangerud, J., Birks, H. H., Halvorsen, L. S., Hughes, A. L., Nashoug, O., Nystuen, J. P., Paus, A., Sørensen, R., and Svendsen, J.-I.: The timing of deglaciation and the sequence of pioneer vegetation at Ringsaker, eastern Norway – and an earthquake-triggered landslide, Norw. J. Geol., 98, 301–318, https://doi.org/10.17850/njg98-3-03, 2018.
Mäntyniemi, P. B., Sørensen, M. B., Tatevossian, T. N., Tatevossian, R. E., and Lund, B.: A reappraisal of the Lurøy, Norway, earthquake of 31 August 1819, Seismol. Res. Lett., 91, 2462–2472, 2020.
Mäntyniemi, P. B., Sørensen, M. B., and Tatevossian, R. E.: Testing the Environmental Seismic Intensity scale on data derived from the earthquakes of 1626, 1759, 1819 and 1904 in Fennoscandia, northern Europe, Geosciences, 11, 14, https://doi.org/10.3390/geosciences11010014, 2021.
Muir Wood, R.: The Scandinavian earthquakes of 22 December 1759 and 31 August 1819, Disasters, 12, 223–236, https://doi.org/10.1111/j.1467-7717.1988.tb00672.x, 1988.
NCCS: SeKlima observations and weather statistics, Norwegian Centre for Climate Services [data set], https://seklima.met.no/, last access: March 2023.
NNSN: NNSN earthquake catalog, Norwegian National Seismic Network [data set], https://nnsn.geo.uib.no/nnsn/#/data/events/search, last access: March 2023a.
NNSN: UIB-NORSAR EIDA node web interface, Norwegian National Seismic Network [data set], https://eida.geo.uib.no/webdc3/, last access: March 2023b.
NVE: The Norwegian landslide database, Norwegian Water Resources and Energy Directorate [data set], https://nve.no/map-services/, last access: March 2023.
Olesen, O., Bungum, H., Dehls, J., Lindholm, C., Pascal, C., and Roberts, D.: Neotectonics, seismicity and contemporary stress field in Norway – mechanisms and implications, Quaternary Geology of Norway, Geological Survery of Norway Special Publication, 13, 145–174, 2013.
Papadopoulos, G. A. and Plessa, A.: Magnitude-distance relations for earthquake–induced landslides in Greece, Eng. Geol., 58, 377–386, 2000.
Prestininzi, A. and Romeo, R.: Earthquake-induced ground failures in Italy, Eng. Geol., 58, 387–397, https://doi.org/10.1016/S0013-7952(00)00044-2, 2000.
Rodriguez, C. E., Bommer, J. J., and Chandler, R. J.: Earthquake-induced landslides: 1980–1997, Soil Dyn. Earthq. Eng., 18, 325–346, 1999.
Sassa, K., Fukuoka, H., Wang, F., and Wang, G.: Landslides Induced by a Combined Effect of Earthquake and Rainfall, in: Progress in Landslide Science, edited by: Sassa, K., Fukuoka, H., Wang, F., and Wang, G., Springer, Berlin, Heidelberg, https://doi.org/10.1007/978-3-540-70965-7_14, 2007.
Smirnova, K.: Potential Seismogenic Faults in Norway, MSc thesis, University of Bergen, https://hdl.handle.net/1956/20412 (last access: March 2023), 2019.
Tatard, L., Grasso, J. R., Helmstetter, A., and Garambois, S.: Characterization and comparison of landslide triggering in different tectonic and climatic settings, J. Geophys. Res., 115, F04040, https://doi.org/10.1029/2009JF001624, 2010.
Wistuba, M., Malik, I., Krzemien, K., Gorczyca, E., Sobucki, M., Wronska-Walach, D., and Gawior, D.: Can low-magnitude earthquakes act as a triggering factor for landslide activity? Examples from the Western Carpathian Mts, Poland, Catena, 171, 359–375, https://doi.org/10.1016/j.catena.2018.07.028, 2018.